Abstract
Purpose
Imbalances between osteogenic and adipogenic differentiation leads to diseases such as osteoporosis. The aim of our study was to demonstrate the differences in extracellular signal-regulated kinase (ERK) phosphorylation during both adipogenesis and osteogenesis of human bone marrow-derived stem cells (BMSCs).
Materials and Methods
Using troglitazone, GW9662 and U0126, we investigated their role in hBMSC differentiation to adipogenic and osteogenic fates.
Results
ERK1/2 inhibition by U0126 suppressed proliferator-activated receptor (PPAR)γ expression and lipid accumulation, while it decreased the mRNA expression of adipogenic genes (lipoprotein lipase, PPARγ, and adipocyte protein) and osteogenic genes (type I collagen and osteopontin). ERK phosphorylation was transient and decreased during adipogenesis, whereas it occurred steadily during osteogenesis. Troglitazone, a PPARγ agonist, induced adipogenesis by inhibiting ERK phosphorylation even in an osteogenic medium, suggesting that ERK signaling needs to be shut off in order to proceed with adipose cell commitment. Cell proliferation was greatly increased in osteogenesis but was not changed during adipogenesis, indicating that ERK might play different roles in cellular proliferation and differentiation between the two committed cell types.
Human bone marrow-derived stem cells (BMSCs) have a capacity to multi-differentiate, into osteoblast, adipocyte, chondrocytes, muscle cells, and neuronal cells, and allowing for self-renewal.1 However, imbalances between osteogenic and adipogenic differentiation have been reported to be related to aging or diseases. In aging patients, or those with osteoporosis, decreased bone density was observed with reduced osteoblast numbers, but with increased adipocyte numbers, suggesting that it is important to balance adipogenesis and osteogenesis in order to maintain bone structure and volume.
Many cytokines control adipogenesis and osteogenesis in BMSCs through multiple signaling pathways. Bone morphogenetic protein (BMP) and transforming growth factor (TGF)β control the expression of cbfa1/runx2 in osteoblast differentiation through the Sma-and Mad-related protein (SMAD) pathway,2 whereas TGFβ and parathyroid hormone (PTH) inhibit adipocyte differentiation.3,4 Dexamethasone and 1,25-dihydroxyvitamin D3 inhibit adipogenic differentiation, but enhance osteogenic differentiation.5,6 It is, therefore, likely that the signals transduced from BMP, TGFβ, PTH, and other local factors act in concert to regulate the differentiation balance between osteoblasts and adipocytes. The mitogen-activated protein kinase (MAPK) pathway is one potential signal transduction pathway that may regulate the proliferation and differentiation of BMSCs. Activation of the MAPK pathway promotes cell differentiation in several cell types such as neuronal cells, T-cells, and muscle cells.7,8 It is possible to control osteogenesis and adipogenesis through the activation or inhibition of MAPKs such as extracellular signal-regulated kinase (ERK), c-Jun N-terminal kinase (JNK), and p38-reactivating kinase (p38). It has been reported that the transdifferentiation between osteoblasts and adipocytes might be regulated by ERK.9 A correlation between ERK and adipocyte differentiation has intensively been studied in mouse 3T3-L1 preadipocytes, however, the results appear contradictory. Inhibition of ERK activity decreased the expression of CCAAT/enhancer-binding protein (C/EBP)α and peroxisome proliferator-activated receptor (PPAR)γ in adipogenesis, while drug or effectors that block adipogenesis activated MAPK activity.3,10-12 Overall, little is known about the regulatory mechanism of ERK in distinguishing between adipogenesis and osteogenesis from human adult stem cells. In this study, therefore, we aimed to identify the exact role of ERK played during the adipogenesis and osteoblastogenesis of BMSCs, and demonstrated the differences in ERK phosphorylation during adipogenesis and osteogenesis of human BMSCs, using agonists or antagonists of PPARγ ligand as well as U0126, an inhibitor of MEK1/2.
Three bone marrows were included in this study. Bone marrow (5 mL) aspirates were obtained from the posterior iliac crest of three healthy volunteers, aged 30-62 years (2 females and 1 male), after obtaining approval from the Institutional Review Board (IRB). All of the donors were patients who underwent bone graft surgery, however, not suffering from any particular disease and were healthy otherwise. Human BMSCs were isolated by Percoll density gradient centrifugation and cultured in DMEM-low glucose medium (Gibco, Carlsbad, CA, USA) as previously described.13 Five-passaged cells were used in all experiments. Cells were cultured in an adipogenic medium [(DMEM-low glucose supplemented with 10% fetal bovine serum (FBS), 0.5 mM isobutyl-methylxanthin, 1 µM dexamethasone, 200 µM indomethacin and 5 µM insulin (Sigma, St. Louis, MO, USA)] or osteogenic medium [(DMEM-low glucose supplemented with 10% FBS, 100 mM dexamethasone, 10 mM β-glycerophosphate, and 50 µg/mL ascorbic acid-2-phosphate (Sigma)]. Human BMSCs were also cultured in an adipogenic medium with 25 µM troglitazone, an agonist of the PPARγ ligand (Sigma). The MEK1/2 inhibitors, U0126 (Promega, Madison, WI, USA), and GW9662 (Sigma), an antagonist of the PPARγ ligand, were used.
Cells were rinsed with phosphate-buffered saline (PBS), fixed in 3.7% formaldehyde for 20 minutes and stained with an Oil Red O solution for 1 hour. The stained cells were photographed and destained in 100% isopropyl alcohol for 20 minutes. The optical density (OD) of the solution was measured at 500 nm. The assays were performed in triplicate wells and each experiment was repeated three times.
Cells were rinsed with PBS and fixed with a 1 : 1 acetone: methanol solution for 30 seconds. They were stained with an Alizarin Red solution for 20 minutes. The stained cells were photographed and destained in 10% cetylpyridinium chloride monohydrate for 30 minutes. The OD of the solution was measured at 540 nm. The assays were performed in triplicate wells and each experiment was repeated at least three times.
The total RNA was isolated using an RNeasy kit (Qiagen, Valencia, CA, USA), and it was reverse-transcribed using an Omniscript kit (Qiagen). PCR reagents were purchased from Qiagen and the specific oligonucleotide primers used were; PPARγ (5'-CCAGAAAGCGATTCCTTCAC, 3'-CACGTTAGTTTCACCTCGGA), aP2 (5'-GGCCAGGAATTTGACGAAGTC, 3'-AGCGTAACTTGAGATGTTGTAAGACA), LPL (5'-GAGATTTCTCTGTATGG CACC, 3'-CTCTTTCACGAGTAAACGTC), Type I collagen (5'-TCCGACCTCTCTCCTCTGAA, 3'-GAGTGG GGTTATGGAGGGAT), Osteopontin (5'-CCAAGTAAG TCCAACGAAA, 3'-GGTGATGTCCTCGTCTGT), GAP DH (5'-GAAGGTGAAGGTCGGAGTC, 3'-GAAGATGGTGATGGGATTTC). The reaction products were analyzed by 1.5% agarose gel electrophoresis. Photographs were quantified using a densitometer and density values for the PCR products were normalized to glyceraldehyde-3-phosphate dehydrogenase (GAPDH) values to yield a semiquantitative assessment (Multigauge, Fujifilm, Tokyo, Japan).
Cell extracts were prepared with 1x passive lysis buffer (Promega), which contained a cocktail of protease and phosphatase inhibitors (Sigma). Twenty microgram aliquots of cell lysates were separated by 10% SDS-PAGE under reducing conditions. Separated proteins were transferred to a PVDF membrane (Amersham Pharmacia Biotech, Piscataway, NJ, USA) at 50 volts for 2 hours in transfer buffer. Membranes were blocked with 5% skimmed milk dissolved in 1x TBST buffer at room temperature for 1 hour. The membranes were probed with the following primary antibodies: activated ERK1/2, ERK1/2, C/EBPα, C/EBPβ, PPARγ and GAPDH (Santa Cruz, Santa Cruz, CA, USA). The immunoblots were visualized using ECL Plus detection kit (Amersham Pharmacia). Photographs were quantified using a densitometer and density values for the PCR products were normalized to GAPDH values to yield a semiquantitative assessment (Multigauge, Fujifilm, Tokyo, Japan).
Osteogenesis and adipogenesis were induced in 12-well plates for 14 days with each induction medium. Cells were fixed with cold methanol and acetone (1 : 1) for 3 minutes. After washed twice with distilled water, the cells were stained with 20% crystal violet (Merck, Darmstadt, Germany) for 10 minutes. After washing the cells twice with distilled water, the stain absorbance was detected at 595 nm after destaining with 95% ethanol.
Adipogenic BMSCs treated with GW9662, a PPARγ antagonist, showed reduced expression of C/EBPα and PPARγ, whereas U0126, MEK1/2 inhibitor, inhibited the expression of PPARγ only (Fig. 1A). BMSCs treated with troglitazone, a PPARγ agonist, showed high lipid accumulation at day 14, whereas cells treated with GW9662 or U0126 showed a significant decrease in adipogenic differentiation (Fig. 1B). This suggests that ERK might inhibit adipogenic differentiation though PPARγ suppression, but not through C/EBPα or C/EBPβ. Lipid accumulation was still found in cells treated with U0126 compared to cells treated with GW9662, because U0126 did not strongly inhibit adipogenic regulatory genes, indicating that ERK signaling is important but not sufficient to regulate adipogenic differentiation. BMSCs treated with troglitazone, GW9662, or U0126 in the adipogenic medium were not stained by Alizarin Red S (Fig. 1B and D). Unexpectedly, in the osteogenic medium, troglitazone induced high-lipid accumulation, but it had no inhibitory effect on osteogenic differentiation (Fig. 1C and D). As seen with the Alizarin Red S staining, neither troglitazone nor GW9662 inhibited osteogenic differentiation, whereas the osteogenic differentiation was significantly suppressed by U0126.
In adipogenesis, ERK phosphorylation in cells cultured in the adipogenic medium alone or with troglitazone was decreased within 1 hour, however, the decrease of ERK phosphorylation was recovered by 20 µM GW9662. ERK phosphorylation showed a second decrease from day 3 post-induction in all the groups. This suggests that GW9662 might inhibit adipogenic differentiation by targeting ERK phosphorylation, and that a transient decrease of ERK phosphorylation was necessary to initiate adipogenesis (Fig. 2A). In osteogenesis, ERK phosphorylation was steadily expressed in all groups except for those in the U0126-supplemented medium (Fig. 2B). However, a decrease of ERK phosphorylation was observed in osteogenic cells treated with troglitazone from day 3 to day 5 post-induction, suggesting that troglitazone might induce or transdifferentiate cells into adipocytes by inhibiting ERK phosphorylation even in the osteogenic medium.
As shown in Fig. 2, different patterns of ERK phosphorylation were observed during adipogenesis and osteogenesis. It is highly likely that different ERK phosphorylation might be correlated with cell proliferation because ERK regulates cell proliferation as well as cell differentiation. To examine the above possibility, human BMSCs were seeded in 12-well plates with equal numbers of cells and cultured in osteogenic or adipogenic induction medium for 14 days. The total cell numbers were counted after staining with crystal violet,14 a dye that binds to DNA (Fig. 3A), and staining-pattern of crystal violet was found to be not different in other donors. There was a great increase in the number of osteogenic cells, whereas the total number of adipogenic cells did not increase compared to the undifferentiated control (Fig. 3B). Based on the different patterns of ERK activation between osteogenic and adipogenic differentiation, we suggest the possibility that the increased cell proliferation in osteogenic differentiation might be regulated by sustained ERK phosphorylation. In contrast, decreased ERK phosphorylation might be necessary for adipogenic differentiation by inhibiting cell proliferation. Further cell cycle analysis would help clarify the role of the ERK signaling in relation to cell proliferation of two differentiation systems.
Adipogenic-specific genes, lipoprotein lipase (LPL), PPARγ2 and adipocyte protein (aP2), were inhibited during adipogenesis in the medium containing GW9662 or U0126, while type I collagen and osteopontin, an osteogenic marker genes, were not expressed (Fig. 4A). The inhibited adipogenesis by U0126 suggests that LPL and aP2, as well as PPARγ2, are regulated by the ERK pathway. In osteogenesis, the expression of LPL, PPARγ2, and aP2 were increased in the osteogenic medium containing troglitazone (*p < 0.05, vs. control) (Fig. 4B). These results support the idea that adipogenic differentiation could be induced even in an osteogenic medium with the addition of troglitazone. The expression of type I collagen and osteopontin was increased time-dependently during osteogenesis (data not shown), but it was inhibited by U0126. Troglitazone and GW9662 did not affect the expression of osteogenic-related genes (*p < 0.05, vs. control), suggesting that ERK activation might play a critical role in both osteogenic and adipogenic differentiation, and that ERK has diverse effects according to its microenvironment.
BMSCs differentiate into distinct lineages through specific signaling pathways in their microenvironment. Investigation of the role of ERK has been focused on the maturation of 3T3-L1 preadipocytes into adipocytes, but each report has showed controversial results.9,14
In our results, U0126 did not affect the expression of C/EBPα or C/EBPβ, but inhibited the expression of PPARγ, indicating that PPARγ is a downstream target of ERK during adipogenesis. Other studies found that ERK activity is necessary for the expression of C/EBPα and PPARγ in 3T3-L1 preadipocytes,12 and that PPARγ is activated by ERK or interacts with ERK-kinase1/2 (MEKs) in cancer.15 In addition, C/EBPβ is a target of ERK2 but not ERK1 in NIH 3T3 fibroblasts,16 indicating that the effect of ERK on the expression of adipogenic transcriptional genes might vary between cell lines or species.
In human diseases such as osteoporosis, the balance between adipocyte and osteoblast differentiation is disrupted.17,18 Therefore, we compared the inhibitory effect of U0126 on differentiation into adipocytes or osteoblasts. Both adipogenic and osteogenic differentiation were blocked by U0126: osteogenic BMSCs treated with U0126 were not differentiated into adipocytes, and also did not induce the expression of adipogenic-specific genes. In contrast, Jaiswal, et al. reported that PD98059, an inhibitor of MEK1, blocks osteogenic differentiation of human BMSCs and induces adipogenic differentiation in an osteogenic medium. In osteoprogenitor KS483 cells, PD98059 dose-dependently inhibits osteogenesis, but stimulates adipogenesis through estrogenic transcriptional activity. However, U0126 inhibites both osteogenesis and adipogenesis,19 suggesting that osteogenesis and adipogenesis from human BMSCs are independently regulated at the downstream of ERK pathways by the modulation of transcriptional factors.
We also observed a different pattern of ERK phosphorylation in the early differentiation stage between osteogenic and adipogenic differentiation. ERK activation was sustained during osteogenic differentiation, but decreased during adipogenic differentiation, indicating that a decrease of ERK activation is necessary to proceed with adipocyte maturation. This possibility was also supported by our observation that adipogenic differentiation could be induced in an osteogenic medium containing troglitazone, accompanied with a decrease of ERK activation. These findings are consistent with previous studies that reported a decrease of ERK phosphorylation during the maturation of 3T3-L1 preadipocytes,12 and that ERK phosphorylation was not affected until day 5 in the osteogenic medium.9 Earlier studies showed that the inhibition of ERK activation blocked both adipogenesis and osteogenesis.9,12
We suggest, therefore, that ERK is necessary for the differentiation of human BMSCs into adipocytes and osteoblast, however, it seems to play a different role in each differentiation process. It is quite possible that cell proliferation would also be affected by ERK activation, because ERK regulates two antagonistic processes, cell proliferation and differentiation, depending upon the situation.20-22 Furthermore, differentiation of cells requires sustained phosphorylation of ERK, whereas transient phosphorylation of ERK induces cell proliferation.20,23 According to our results, the duration and magnitude of ERK phosphorylation were different between adipogenic and osteogenic differentiation, and the differences were also correlated with cell proliferation. Cell numbers were greatly increased during osteogenic differentiation compared to adipogenic differentiation, suggesting that ERK activated during osteogenic differentiation might induce both cell proliferation and differentiation, whereas it might be involved in differentiation rather than cell proliferation during adipogenic differentiation. There is considerable evidence to indicate that adipocyte maturation requires a precise proliferative step, called mitotic clonal expansion (MCE), with ERK activation in the beginning stage; ERK inhibition blocks MCE and the expression of cell cycle markers (cyclin A and cdk2) in the middle stage, whereas ERK activation returned to a low level accompanying PPARγ expression during terminal differentiation.24 This observation is consistent with our results, which showed a burst of ERK activation from 3 hours and up to day 1 without the expression of PPARγ2, followed by a decrease of ERK activation concurrently with PPARγ2 induction from day 3. Furthermore, induced adipogenic genes, including PPARγ2, were suppressed by ERK inhibition, suggesting that a minimum or transient ERK activation is necessary for the induction of adipogenic regulatory genes rather than cell proliferation during the adipogenesis of human BMSCs.
We also found a positive regulatory effect of ERK on osteogenic genes, because osteopontin and type I collagen were inhibited by U0126. It should be noted that Cbfa1, one of the osteogenic transcription factors, was phosphorylated by ERK in rat osteoblastic cell lines,19 and some studies showed that osteogenic genes such as cbfa1 and osteocalcin are regulated by the ERK pathway, and that ERK inactivation blocked alkaline phosphatase (ALP) activity and nodule formation in osteoprogenitor KS483 cells.25-28
In conclusion, we demonstrated herein different but common roles of ERK in the adipogenesis and osteogenesis of human BMSCs. At the initial stage of differentiation, the duration and magnitude of ERK activation are highly critical factors in the determination of osteogenesis and adipogenesis of the human BMSCs (Fig. 5). A precise understanding of these mechanisms in human BMSCs would certainly be crucial to developing potent drugs against diseases modulating the ERK pathway.
Figures and Tables
Fig. 1
Effect of agonists or antagonists of PPARγ and U0126 on adipogenic or osteogenic differentiation. (A) Human BMSCs were cultured in an adipogenic medium with or without troglitazone (25 µM), GW9662 (20 µM), or U0126 (20 µM) for 7 days. The lysates were subjected to western blot analysis. (B) BMSCs were induced adipocyte differentiation in the adipogenic medium (AD) for 14 days, and stained by Oil red O or Alizarin red S. (C) BMSCs were differentiated into osteoblasts in an osteogenic medium (OS) for 14 days, and stained by Oil red O or Alizarin red S. (D) The degree of adipogenic or osteogenic differentiation was determined as OD units by destaining (*p < 0.05, vs media). GAPDH, glyceraldehyde-3-phosphate dehydrogenase; PPARγ, peroxisome proliferator-activated receptor; BMSCs, bone marrow-derived stem cells.
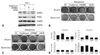
Fig. 2
ERK phosphorylation during the adipogenesis and osteogenesis of human BMSCs. (A) Human BMSCs were cultured in an adipogenic medium (AD) with or without troglitazone, GW9662, or U0126 for 5 days. The lysates were subjected to western blot analysis using antibodies specific for ERK1/2 and pERK. (B) Human BMSCs were cultured in an osteogenic medium (OS) with or without troglitazone, GW9662, or U0126. The lysates were subjected to western blot analysis using antibodies specific for ERK1/2 and pERK. ERK, extracellular signal-regulated kinase; BMSCs, bone marrow-derived stem cells.
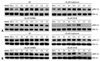
Fig. 3
Change in total cell numbers during the osteogenesis and adipogenesis of human BMSCs. (A) Cells were incubated in an osteogenic (OS) (c and e) or adipogenic (AD) (d and f) medium for 14 days, and stained by Alizarind red S (a and c), Oil red O (b and d), and crystal violet (e and f). a and b are control comparing to adipogenesis and osteogenesis. a and b cultured control medium, DMEM-low glucose with 10% fetal bovine serum. (B) Crystal violet (CV) stain was quantified by destaining with 95% ethanol, and expressed as OD units (*p < 0.05, vs -OS media). BMSCs, bone marrow-derived stem cells.
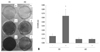
Fig. 4
RT-PCR analysis during the adipogenesis (AD) and osteogenesis (OS) of BMSCs. (A) BMSCs were cultured in the adipogenic medium with or without troglitazone, GW9662, or U0126. Equal aliquots of the total RNA were reverse transcribed and amplified with the oligonucleotide primers specific for LPL, aP2, and PPARγ2. (B) Cells were induced with troglitazone compounds in the osteogenic medium for 7 days. Equal aliquots of total RNA were reverse transcribed and amplified with the oligonucleotide primers specific for LPL, aP2, PPARγ2, Type I collagen, and osteopontin. BMSCs, bone marrow-derived stem cells; LPL, lipoprotein lipase; aP2, adipocyte protein; PPARγ2, peroxisome proliferator-activated receptorγ2; GAPDH, glyceraldehyde-3-phosphate dehydrogenase.
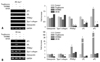
ACKNOWLEDGEMENTS
This research was supported by a grant (code: SC3210) from Stem Cell Research Center of the 21st Century Frontier Research Program funded by the Ministry of Education, Science and Technology, Republic of Korea.
References
1. Pittenger MF, Mackay AM, Beck SC, Jaiswal RK, Douglas R, Mosca JD, et al. Multilineage potential of adult human mesenchymal stem cells. Science. 1999. 284:143–147.


2. Yamaguchi A, Komori T, Suda T. Regulation of osteoblast differentiation mediated by bone morphogenetic proteins, hedgehogs, and Cbfa1. Endocr Rev. 2000. 21:393–411.


3. Chan GK, Deckelbaum RA, Bolivar I, Goltzman D, Karaplis AC. PTHrP inhibits adipocyte differentiation by down-regulating PPAR gamma activity via a MAPK-dependent pathway. Endocrinology. 2001. 142:4900–4909.
4. Chin BY, Petrache I, Choi AM, Choi ME. Transforming growth factor beta1 rescues serum deprivation-induced apoptosis via the mitogen-activated protein kinase (MAPK) pathway in macrophages. J Biol Chem. 1999. 274:11362–11368.


6. Beresford JN, Bennett JH, Devlin C, Leboy PS, Owen ME. Evidence for an inverse relationship between the differentiation of adipocytic and osteogenic cells in rat marrow stromal cell cultures. J Cell Sci. 1992. 102:341–351.


7. Zhao LR, Duan WM, Reyes M, Keene CD, Verfaillie CM, Low WC. Human bone marrow stem cells exhibit neural phenotypes and ameliorate neurological deficits after grafting into the ischemic brain of rats. Exp Neurol. 2002. 174:11–20.


8. Toma C, Pittenger MF, Cahill KS, Byrne BJ, Kessler PD. Human mesenchymal stem cells differentiate to a cardiomyocyte phenotype in the adult murine heart. Circulation. 2002. 105:93–98.


9. Jaiswal RK, Jaiswal N, Bruder SP, Mbalaviele G, Marshak DR, Pittenger MF. Adult human mesenchymal stem cell differentiation to the osteogenic or adipogenic lineage is regulated by mitogen-activated protein kinase. J Biol Chem. 2000. 275:9645–9652.


10. Aubert J, Dessolin S, Belmonte N, Li M, McKenzie FR, Staccini L, et al. Leukemia inhibitory factor and its receptor promote adipocyte differentiation via the mitogen-activated protein kinase cascade. J Biol Chem. 1999. 274:24965–24972.
11. Bost F, Caron L, Marchetti I, Dani C, Le Marchand-Brustel Y, Binétruy B. Retinoic acid activation of the ERK pathway is required for embryonic stem cell commitment into the adipocyte lineage. Biochem J. 2002. 361:621–627.


12. Prusty D, Park BH, Davis KE, Farmer SR. Activation of MEK/ERK signaling promotes adipogenesis by enhancing peroxisome proliferator-activated receptor gamma (PPARgamma) and C/EBPalpha gene expression during the differentiation of 3T3-L1 preadipocytes. J Biol Chem. 2002. 277:46226–46232.
13. Kim SH, Choi YR, Park MS, Shin JW, Park KD, Kim SJ, et al. ERK 1/2 activation in enhanced osteogenesis of human mesenchymal stem cells in poly(lactic-glycolic acid) by cyclic hydrostatic pressure. J Biomed Mater Res A. 2007. 80:826–836.


14. Hossain MM, Hwang DY, Huang QQ, Sasaki Y, Jin JP. Developmentally regulated expression of calponin isoforms and the effect of h2-calponin on cell proliferation. Am J Physiol Cell Physiol. 2003. 284:C156–C167.


15. Burgermeister E, Seger R. MAPK kinases as nucleo-cytoplasmic shuttles for PPARgamma. Cell Cycle. 2007. 6:1539–1548.


16. Hanlon M, Sturgill TW, Sealy L. ERK2- and p90(Rsk2)-dependent pathways regulate the CCAAT/enhancer-binding protein-beta interaction with serum response factor. J Biol Chem. 2001. 276:38449–38456.


17. Verma S, Rajaratnam JH, Denton J, Hoyland JA, Byers RJ. Adipocytic proportion of bone marrow is inversely related to bone formation in osteoporosis. J Clin Pathol. 2002. 55:693–698.


19. Dang ZC, Lowik CW. Differential effects of PD98059 and U0126 on osteogenesis and adipogenesis. J Cell Biochem. 2004. 92:525–533.


20. Traverse S, Seedorf K, Paterson H, Marshall CJ, Cohen P, Ullrich A. EGF triggers neuronal differentiation of PC12 cells that overexpress the EGF receptor. Curr Biol. 1994. 4:694–701.


21. Schaeffer HJ, Weber MJ. Mitogen-activated protein kinases: specific messages from ubiquitous messengers. Mol Cell Biol. 1999. 19:2435–2444.


22. Tan PB, Kim SK. Signaling specificity: the RTK/RAS/MAP kinase pathway in metazoans. Trends Genet. 1999. 15:145–149.


23. Marshall CJ. Specificity of receptor tyrosine kinase signaling: transient versus sustained extracellular signal-regulated kinase activation. Cell. 1995. 80:179–185.


24. Tang QQ, Otto TC, Lane MD. Mitotic clonal expansion: a synchronous process required for adipogenesis. Proc Natl Acad Sci U S A. 2003. 100:44–49.


25. Salasznyk RM, Klees RF, Hughlock MK, Plopper GE. ERK signaling pathways regulate the osteogenic differentiation of human mesenchymal stem cells on collagen I and vitronectin. Cell Commun Adhes. 2004. 11:137–153.


26. Hipskind RA, Bilbe G. MAP kinase signaling cascades and gene expression in osteoblasts. Front Biosci. 1998. 3:d804–d816.

