Abstract
Intracortical microstimulation (ICMS) is a technique that was developed to derive movement representation of the motor cortex. Although rats are now commonly used in motor mapping studies, the precise characteristics of rat motor map, including symmetry and consistency across animals, and the possibility of repeated stimulation have not yet been established. We performed bilateral hindlimb mapping of motor cortex in six Sprague-Dawley rats using ICMS. ICMS was applied to the left and the right cerebral hemisphere at 0.3 mm intervals vertically and horizontally from the bregma, and any movement of the hindlimbs was noted. The majority (80%±11%) of responses were not restricted to a single joint, which occurred simultaneously at two or three hindlimb joints. The size and shape of hindlimb motor cortex was variable among rats, but existed on the convex side of the cerebral hemisphere in all rats. The results did not show symmetry according to specific joints in each rats. Conclusively, the hindlimb representation in the rat motor cortex was conveniently mapped using ICMS, but the characteristics and inter-individual variability suggest that precise individual mapping is needed to clarify motor distribution in rats.
Intracortical microstimulation (ICMS) can derive movement representation of the motor cortex in central nervous system disease. Neurophysiologic experimental studies using ICMS in animals began recently and are ongoing (1, 2, 3, 4). As studies of ICMS, using the rodents are generalized and experiments with the rats are becoming more common being cost effective and convenient to manage (5). However, despite the existence of a number of ICMS studies using rats, accurate maps of the rat motor cortex and optimal stimulation parameters have not yet been established (5).
The motor cortex mapping is essential to develop a new treatment or device using the animal models in the central nervous system disorder. If hindlimb mapping is achieved accurately and consistently, it will be helpful to research the effect of the motor cortex stimulation for treating the intractable pain or Parkinson's disease (6, 7). Also, it is expected to be the basic step for developing artificial neural network device such as spinal cord-machine interface to treat spinal cord injury models and research of neural plasticity (6, 7).
In this study, we focused on the analysis of characteristics of hindlimb motor cortex such as symmetricity in each animal, the consistency across animals, and possibility of repeated stimulation in each point.
Sixteen male Sprague Dawley rats (Orient Bio Inc., Seongnam, Korea), weighing 250-300 g were used in this study. Before mapping, all rats were housed two per cage under simulated daylight conditions with alternating 12-hr light-dark cycles, and had free access to food and water. The environment temperature was maintained at 22±2℃.
Because the purpose of our study is to find out the movement of hindlimb induced by ICMS, Rompun (Bayer, Leverkusen, Germany) for a muscle relaxant was excluded and we used only Zoletil (40 mg/kg) (Virbac S.A., Carros, France) which was injected intraperitoneally for induction and maintenance of anesthesia. We performed a 10×4 mm craniotomy at each side after midline vertical scalp incision on the vertex of rat. Each side of bony window exposed from 5 mm anterior to 5 mm posterior of bregma and from 1 mm to 5 mm lateral of midline (Fig. 1). With an 33-gauge needle, dura was carefully removed.
Bipolar electrodes were inserted to a depth of 1.5 mm from the neocortical surface. The diameter of bipolar electrodes was 0.2 mm. Bregma was chosen as the center of matrix which divided by 0.3 mm square coordinates to penetrate and stimulate in the center of each square, and skipped when located over a blood vessel. Brain swelling or bleeding by minor injury disturbing a stable stimulation were tried to be avoided. Stimulation parameters consisted of 0.02 msec-long, a frequency of 300 Hz, an intensity 4 mA and 12 pulse trains/sec.
First, we investigated the movement of hindlimb provoked by the left side ICMS. Three days later, the mapping at the right side motor cortex was performed in the same way, and we evaluated the bilateral symmetricity of stimulation points. Antibiotics administration started from the first operation for preventing infection.
Rats were kept in prone position during stimulation. Bilateral forelimbs and hindlimbs were not fixed and were freely hanging so that it was possible to observe the movement of hip, knee and ankle joints at a time. We conducted the mapping of exposed cortical surface except blood vessels. Cortical surface was stimulated from 5 mm anterior to 5 mm posterior of bregma and from 1 mm to 5 mm lateral of midline with intervals of 0.3 mm. The results were recorded using a matrix table composed of squares corresponding to each stimulation points in brain (0.3×0.3 mm). Only the respond points were demarcated at the matrix table as an abbreviation (Fig. 2).
Sixteen rats were used in this study, but finally motor cortex mapping was obtained only in six rats. The cases unable to perform mapping in early ten rats were due to brain swelling caused by repeated electrode penetration as well as inappropriate stimulation parameter or microbleeding during dura opening.
The motor abilities of the six rats that completed the study did not decrease by penetration technique and cortical stimulation. All the hindlimb movements were developed from the contralateral side of hemisphere ICMS conducted. In most rats, the stimulation points that caused movement of a given joint were irregulary and widely distributed. Particulary, the motor cortex of hindlimbs was found to be located on the convex side which is different from the human motor cortex of lower extremity that located in the interhemispheric area dominantly.
All movement of hindlimb's joints appeared in flexion only, not appeared in extension. The majority (80%±11.2%) of responses were not restricted to a single joint, and movement occurred simultaneously in two or three hindlimb joints (Table 1). The results of mapping in six rats are shown in Fig. 2. The motor maps were asymmetric, and were not consistent across the six rats. There were thirty points which showing the same joints response in more than four rats, and only one point (3.1 mm left lateral from the midline, 1.5 mm posterior to the bregma) that elicited the same movement (knee flexion) in all six rats (Fig. 3). However, when indicate three-dimensional summary of cortical mapping according to individual joints, the overall shape is seem to be symmetrical (Fig. 4). The mean areas of hindlimb representation were 2.5±5.1 mm2 at left hemisphere, 1.9±0.9 mm2 at right hemisphere and 2.2±5.3 mm2 in total.
Seven days after brain mapping, the cortex was reexposed to evaluate the possibility of repeated stimulation. However, repeated motor cortex stimulation was not possible in any of the six rats due to brain swelling and surface hemorrhage even though we used anti-adhesion film (Surgiwrap®, MAST, Zurich, Switzerland) between brain cortex and scalp to prevent wound adhesion.
The study on the cortical surface stimulation was begun earlier than intracortical stimulation, which can stimulate motor cortex with lower amplitude, but the specificity is lower than intracortical stimulation (1, 2). ICMS has the advantage of pyramidal cell stimulation (1, 2, 8). In this study, ICMS was tried in 16 rats and only final six rats showed cortical stimulation with hindlimb response over ten points of stimulation. In early ten rats, stimulation of motor cortex was not possible due to hematoma or swelling mainly from damage during dural opening and electrode insertion injury. These obstacles were overcome by use of a microscope and fine bipolar electrode with 0.2 mm diameter. The optimal parameters for motor cortex stimulation are not known, but in this study, we used stimuli of 0.02 ms, 300 Hz, 4 mA, 12 times pulses/sec delivered at 1.5 mm depth where layer 5, 6 exist. These parameters are similar to previous reports (5, 9, 10, 11, 12).
In the human cortex, the motor area of hindlimb is located in the interhemispheric region. On the contrary, motor cortex in the rat was reported to be located on the convex side of the cerebral hemisphere and our data also support these previous reports (3, 4, 16, 17). Early studies of the rat motor cortex showed that it is represented in the medial and lateral agranular fields of the frontal lobe, which controls face, forelimb, hindlimb and body (13, 14, 15), and most studies except two (16, 18) described the area as a whole movement of forelimb, whisker, neck or tail rather than specific joints (5, 9, 10, 11). The studies which analysed individual joint response reported that hindlimb area is behind bregma; ankle and knee joints tend to be located lateral side of hip joint (16, 18). By contrast, our results showed that all hindlimb joints including the hip, knee, and ankle were intermingled without any consistent distribution.
In regarding to the areal extents of hindlimb representation, this study showed slightly larger dimension with wide variation (2.2±5.3 mm2 in total) than previous report (2.0±0.5 mm2) (16). In addition, one experimental animal showed that anterior margin of hindlimb representation at left side was located beyond bregma anteriorly in our study. This finding is different from other studies which showed the hindlimb motor cortex was distributed behind bregma (16, 19). These discrepancies about the size and distribution of hindlimb motor cortex might be caused by strain related phenomenon as mentioned in previous study (17).
In this study, we clearly observed that stimulation at a single location could elicit simultaneous movements in multiple joints. Especially, 47% of stimulation showed three joints contraction and the average of response with more than two joints was 80%±11.2%. In the literature, multiple joints response in one stimulation was reported variously and overlapping of sensory and motor cortex also reported (14, 19, 20). This is likely due to cortico-cortical connection of axons within the motor cortex, as has been reported using the tract-tracing technique (21).
We performed motor mapping in both hemispheres to clarify the degree of symmetry in the somatotopic organisation of the rat motor cortex. Although the precise joints stimulated by ICMS do not show symmetricity in each animal (Fig. 2), the overall shape of motor maps indicated with numbers of stimulated joint exhibited grossly symmetrical shape (Fig. 4), supporting previous reports of symmetrical motor maps for gross movements of forelimb, whisker, neck muscle and tail (5, 9, 10, 11, 15). The only two previous studies which described specific joints analyzed unilateral hemisphere and did not study the symmetricity (16, 18).
All movements of hindlimb's joints appeared in flexion only without extension in this study. Also, the previous reports of hindlimb mapping of specific joints in the rat observed predominantly flexion responses, with the exception of plantar flexion, even though they stimulated with very low amplitude (16, 18). We hypothesize that in the rat motor cortex, intercellular connections for flexion movement are dominant rather than extension movement in rats, and this phenomenon could explain the exclusive responses of hindlimb flexion to ICMS.
We performed hindlimb mapping of the rat motor cortex using ICMS. The results showed high inter-individual variations in the hindlimb motor map, asymmetry according to specific joints, and response of multiple joints to stimulation at a single location, which suggest that precise individual mapping is needed to clarify motor distribution in rats.
Figures and Tables
Fig. 1
A schematic diagram of craniotomy in rat. A median incision was performed on the scalp and the dura mater was exposed after two (left and right) rectangular craniotomy (each 10 mm length and 4 mm width) using the bregma as the reference point. Finally, the dura was removed.
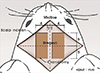
Fig. 2
Motor maps in six rats (A-F). The functional mappings obtained by intracortical motor cortex stimulation in bilateral hemisphere were recorded using a matrix table composed of squares corresponding to each stimulation points in the brain (0.3 mm × 0.3 mm). The motor maps were asymmetric, and were not consistent across the six rats. H, hip flexion; K, knee flexion; A, ankle dorsiflexion; FL, forelimb; HL, hind limb; T, tail; W, whiskers.
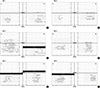
Fig. 3
Summary of motor maps in six rats. Numbers in each squares of a matrix table indicate the numbers of rats that exhibited a motor response at each joint (hip, knee, ankle) in response to intracortical microstimulation of left (A) and right (B) hemisphere. The stimulations of each site usually represent more than two joints (80%). There were thirty points which showing the same joints response in more than four rats, and we found only one point (3.1 mm left lateral from the midline, 1.5 mm posterior to the bregma, indicated by grey highlight) that elicited the same movement (knee flexion) in all six rats. H, hip flexion; K, knee flexion; A, ankle dorsiflexion.
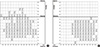
Fig. 4
Three-dimensional summary of motor maps in six rats showing the distribution of each joint according ankle (A, B), knee (C, D) and hip (E, F) to intracortical microstimulation of left and right hemisphere. Even though the precise joints stimulated by ICMS do not show symmetricity in each animal, the overall shape of motor maps indicated with numbers of stimulated joint exhibited grossly symmetrical shape.
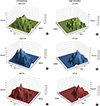
Notes
References
1. Asanuma H, Rosén I. Topographical organization of cortical efferent zones projecting to distal forelimb muscles in the monkey. Exp Brain Res. 1972; 14:243–256.
2. Asanuma H, Ward JE. Patterns of contraction of distal forelimb muscles produced by intracortical stimulation in cats. Brain Res. 1971; 27:97–109.
3. Kleim JA, Barbay S, Nudo RJ. Functional reorganization of the rat motor cortex following motor skill learning. J Neurophysiol. 1998; 80:3321–3325.
4. Kolb B, Tees RC. The cerebral cortex of the rat. Cambridge: MIT Press;1990.
5. Young NA, Vuong J, Flynn C, Teskey GC. Optimal parameters for microstimulation derived forelimb movement thresholds and motor maps in rats and mice. J Neurosci Methods. 2011; 196:60–69.
6. Gharbawie OA, Williams PT, Kolb B, Whishaw IQ. Transient middle cerebral artery occlusion disrupts the forelimb movement representations of rat motor cortex. Eur J Neurosci. 2008; 28:951–963.
7. Young NA, Vuong J, Ozen LJ, Flynn C, Teskey GC. Motor map expansion in the pilocarpine model of temporal lobe epilepsy is dependent on seizure severity and rat strain. Exp Neurol. 2009; 217:421–428.
8. Telfeian AE, Connors BW. Widely integrative properties of layer 5 pyramidal cells support a role for processing of extralaminar synaptic inputs in rat neocortex. Neurosci Lett. 2003; 343:121–124.
9. Fonoff ET, Pereira JF Jr, Camargo LV, Dale CS, Pagano RL, Ballester G, Teixeira MJ. Functional mapping of the motor cortex of the rat using transdural electrical stimulation. Behav Brain Res. 2009; 202:138–141.
10. Hosp JA, Molina-Luna K, Hertler B, Atiemo CO, Stett A, Luft AR. Thin-film epidural microelectrode arrays for somatosensory and motor cortex mapping in rat. J Neurosci Methods. 2008; 172:255–262.
11. Molina-Luna K, Buitrago MM, Hertler B, Schubring M, Haiss F, Nisch W, Schulz JB, Luft AR. Cortical stimulation mapping using epidurally implanted thin-film microelectrode arrays. J Neurosci Methods. 2007; 161:118–125.
12. Xie N, Yang Q, Chappell TD, Li CX, Waters RS. Prenatal alcohol exposure reduces the size of the forelimb representation in motor cortex in rat: an intracortical microstimulation (ICMS) mapping study. Alcohol. 2010; 44:185–194.
13. Brecht M, Krauss A, Muhammad S, Sinai-Esfahani L, Bellanca S, Margrie TW. Organization of rat vibrissa motor cortex and adjacent areas according to cytoarchitectonics, microstimulation, and intracellular stimulation of identified cells. J Comp Neurol. 2004; 479:360–373.
14. Donoghue JP, Wise SP. The motor cortex of the rat: cytoarchitecture and microstimulation mapping. J Comp Neurol. 1982; 212:76–88.
15. Tennant KA, Adkins DL, Donlan NA, Asay AL, Thomas N, Kleim JA, Jones TA. The organization of the forelimb representation of the C57BL/6 mouse motor cortex as defined by intracortical microstimulation and cytoarchitecture. Cereb Cortex. 2011; 21:865–876.
16. Frost SB, Iliakova M, Dunham C, Barbay S, Arnold P, Nudo RJ. Reliability in the location of hindlimb motor representations in Fischer-344 rats: laboratory investigation. J Neurosurg Spine. 2013; 19:248–255.
17. VandenBerg PM, Hogg TM, Kleim JA, Whishaw IQ. Long-Evans rats have a larger cortical topographic representation of movement than Fischer-344 rats: a microstimulation study of motor cortex in naïve and skilled reaching-trained rats. Brain Res Bull. 2002; 59:197–203.
18. Gu X, Staines WA, Fortier PA. Quantitative analyses of neurons projecting to primary motor cortex zones controlling limb movements in the rat. Brain Res. 1999; 835:175–187.
19. Neafsey EJ, Bold EL, Haas G, Hurley-Gius KM, Quirk G, Sievert CF, Terreberry RR. The organization of the rat motor cortex: a microstimulation mapping study. Brain Res. 1986; 396:77–96.
20. Gioanni Y, Lamarche M. A reappraisal of rat motor cortex organization by intracortical microstimulation. Brain Res. 1985; 344:49–61.
21. Weiss DS, Keller A. Specific patterns of intrinsic connections between representation zones in the rat motor cortex. Cereb Cortex. 1994; 4:205–214.