Abstract
In this study of a developed soft tissue filler, adipose tissue equivalents were constructed using adipose stem cells (ASCs) and micronized acellular dermal matrix (Alloderm). After labeling cultured human ASCs with fluorescent green protein and attaching them to micronized Alloderm (5×105 cells/1 mg), ASC-Alloderm complexes were cultured in adipogenic differentiation media for 14 days and then injected into the dorsal cranial region of nude male mice. The viabilities of ASCs in micronized Alloderm were determined at 1, 4, 7, and 14 days, and complexes, which had been cultured for 14 days and implanted in vivo for 2 months, were histologically evaluated by light, confocal, and scanning electron microscopy. The viabilities represented that ASCs in micronized Alloderm were alive during the culture period. ASC-Alloderm complexes cultured for 14 days contained round cells with large lipid vesicles by light microscopy and many spherical cells by SEM. ASCs in implanted ASC-Alloderm complexes harvested from mice at 2 months postinjection were histologically found to have differentiated into adipocytes which had green fluorescence dye. Micronized Alloderm may be found useful as scaffold for human ASCs when constructing fat tissue for three-dimensional soft tissue filling. The present study suggests that ASC-Alloderm complexes can be used as injectable three-dimensional soft tissue fillers.
A number of injectable materials have popularly been used to treat soft tissue defects, although fat injections or grafts are most popular. Micronized acellular dermal matrix (Alloderm), an injectable material, is a highly versatile intermediate-length implant with many applications. However, though cell ingrowths and revascularization of implanted micronized Alloderm are an important factor for lasting the length of results, this process unfortunately does not appear to occur in everyone (1). To improve results after injecting micronized Alloderm, the authors admixed micronized Alloderm with adipose stem cells (ASCs). ASCs have been used in adipose tissue engineering (2, 3), because ASCs, harvested sufficiently from adipose tissue, are known to possess the ability of high proliferation and strong differentiation to adipocytes (4) and endothelial cells (5). The authors considered that adipocytes differentiated from ASCs in micronized Alloderm would make the injected micronized Alloderm a more acceptable soft tissue substitute, and the differentiated endothelial cells would enhance implantation of the injected soft tissue constructs.
To develop an injectable soft tissue filler, the authors tried to produce adipose tissue equivalents using ASCs and micronized Alloderm as scaffold by introducing the concept of tissue engineering.
Human ASCs were isolated from adipose tissue obtained by abdominoplasty via enzymatic digestion. Briefly, after removing visible fibrous tissue and vessels, adipose tissue was finely minced and enzymatically digested in dulbeccos modified eagles medium (DMEM)/F-12 media (Gibco, Gaithersburg, MD, U.S.A.) containing 0.1% type I collagenase (Sigma Chemical Co., St. Louis, MO, U.S.A.), 1% fatty acid free bovine serum albumin (Sigma), and 1 × Penicillin-Streptomycin (Gibco) for 30 min at 37℃ at adipose tissue to a dissociation medium ratio of 2 g/1 mL. The digested tissue was filtered through cotton gauze, and the filtrate suspension was centrifuged at 1,000 rpm for 5 min, cell pellet were then resuspended in DMEM/F-12 media (Gibco) supplemented with 10% bovine calf serum (Hyclone, Logan, UT, U.S.A.) and 1 × penicillin-streptomycin (Sigma). ASCs were plated at 104 cells/mL and passaged two or three times.
PKH67 green fluorescent cell linker kits (Sigma) were used for labeling ASCs. Briefly, ASCs were trypsinized and washed once with DMEM/F-12 medium without serum. 2 × 107 cells suspended in medium were placed in 15 mL centrifuge tubes, and centrifuged (400 × g) for 5 min to form loose pellets. Medium was then carefully aspirated to leave no more than 25 µL of residual medium on pellets. The cells were then resuspended in this residual medium, and 1 mL of Diluent C was added. Immediately prior to staining, a 2 × staining solution of PKH67 was prepared in polypropylene tube by diluting 4 µL of 1 mM dye stock in 1 mL of Diluent C. Staining was initiated by rapidly adding a 2 × concentrated cell suspension to the 2 × dye solution. Staining was stopped after 5 min by adding an equal volume (2 mL) of fetal bovine serum over a period of 1 min followed by an equal volume (4 mL) of complete medium containing 10% serum. Cells were then centrifuged and washed three times with 10 mL of complete medium. Stained cells were cultured as monolayers in adipogenic differentiation media (Zen-Bio Co, Research Triangle, NC, U.S.A.) for 14 days. ASCs cultured in adipogenic differentiation media were visualized and photographed under an inverted microscope (Axiovert 200 M, Zeiss, Göttingen, Germany) equipped with a color digital camera. A fluorescein isothiocyanate fluorescent filter set was used to visualize labeled cells.
Micronized acellular dermal matrix (micronized Alloderm) was purchased from Sheba™ (Hans Biomed Co., Seoul, Korea). Briefly, micronized Alloderm in a syringe was hydrated with 6 mL of DMEM/F-12 media (Gibco) for 30 min, and centrifuged at 1,000 rpm for 5 min. Supernatant was aspirated off, and 3mL media was added.
ASC-Alloderm complexes were made by mixing human ASCs and micronized Alloderm at 5×104 cells/mg of micronized Alloderm. After incubating with rocking overnight, 10 µL aliquots of the complex (10 mg Alloderm with ASCs) were divided into each well of ten 24-well culture plates containing 1 mL DMEM/F-12 media supplemented with 10% bovine calf serum, and 100 U/mL penicillin-streptomycin. To induce differentiation, media were replaced with adipogenic differentiation media 24 hr after plating, and plates were incubated for 14 days in an incubator (37℃, 5% CO2). Differentiation media were changed every 3 days.
At 1, 4, 7, and 14 days after seeding ASCs in micronized Alloderm, 200 µL of sodium 3'-[1-(phenylamino-carbonyl)-3,4-tetrazolium]-bis (4-methoxy-6-nitro) benzene sulfonic acid hydrate labeling reagent (XTT; Boehringer Mannheim GmbH, Mannheim, Germany) with electron-coupling reagent were added to each well (described above) and incubated for 24 hr. The absorbances of media in wells were determined at 490 nm and 690 nm by spectrophotometry. Cell viabilities were expressed by calculating the difference between 'the defference between two absorbances of media of sample in well' and 'the difference between two absorbances of DMEM/F-12 medium containing XTT reagent'.
Cultured human ASCs were labeled using PKH67 green fluorescent cell linker kits (Sigma) to determine whether adipose formation in complexes originated from seeded cells or from surrounding perivascular tissue. Subsequently, ASCs were mixed with micronized Alloderm as described above. ASC-Alloderm complexes were cultured in adipogenic differentiation media for 2 weeks, then 50 mg of ASC-Alloderm complexes were gently injected subcutaneously into the dorsal cranial region of nude male mice using 1 mL syringe with 18 G needle. Among the 8 mice used, 4 mice were injected with only micronized Alloderm, and 4 mice were injected with ASC-Alloderm complex. Two months later, the four mice were sacrificed and the injected tissues were excised. The excised masses were weighed and then fixed in 10% formalin or OCT compound.
ASC-Alloderm complexes were obtained after 14 days of in vitro culture, and injected tissues were obtained from nude mice 2 months after implantation.
For hematoxylin and eosin (H&E) staining, samples were fixed in 10% formalin, prepared as paraffin blocks, sectioned at 6 µm, and stained with H&E.
For Oil-red O staining, injected ASC-Alloderm complexes excised from nude mice at 2 months after implantation (frozen in dry ice and stored at -80℃) were placed in a cryomold with OCT (Tissue-Tek®, Sakura Finetek Inc., Torrance, CA, U.S.A.). Ten-micrometer sections were cut using a microtome, placed on slides, and stained with Oil-red O. Nuclei were then counterstained with hematoxylin, and sections were then coverslipped. The slides were examined under a confocal microscope (MRC 1024 MP, Bio-Rad, Hercules, CA, U.S.A.).
ASC-Alloderm complexes obtained after 14 days of in vitro culture were treated with a fixative containing 3% glutaraldehyde and 4% paraformaldehyde in 0.1 M cacodylate buffer (all pH 7.2) for 1 hr at room temperature. Samples were rinsed with 0.1 M cacodylate buffer (3×, 5 min), post-fixed with 1% osmium tetroxide in 0.1 M cacodylate buffer for 2 hr, dehydrated in ethanol, subjected to critical point drying, and sputter coated. The samples were examined in a JSM 5410 LV (Jeol LTD, Tokyo, Japan) at 5 kV.
Results are expressed as mean±standard deviation. Statistical analysis was performed by one-way analysis of variance (one-way ANOVA) using SPSS Ver. 10 for Windows. Multiple comparison of Scheffe was used for comparing the differences for these different culture periods. Differences were considered significant at p<0.05.
ASCs viabilities were; 0.61±0.19, 0.71±0.09, 0.79±0.15, and 0.86±0.14 after culture for 1, 4, 7, and 14 days (one-way ANOVA, F=2.939, p=0.065). Although these rates showed an increasing tendency with culture time (Fig. 2), no significant difference was observed for these different culture periods (p>0.05).
By H&E histological examination, some ASCs in micronized Alloderm were observed to have differentiated and to contain large lipid vesicles after 14 days (Fig. 3).
All animals remained healthy throughout the experimental period. At two months after implantation, injected tissues were easily identified in the cranial region. The weight of injected tissues of micronized Alloderm injected group and ASC-Alloderm complex injected group was 51.4±5.8 mg and 54.6±3.7 mg, respectively, but there was no significant difference between the weights of two groups (p>0.05). An H&E histological examination revealed that some cells and small capillary had infiltrated the micronized dermal matrix in controls (Fig. 5A), whereas markedly increased numbers of large signet-ring cells and large capillaries were observed in ASC-Alloderm complex injected mice (Fig. 5B). Moreover, in injected ASC-Alloderm complexes, signet-ring cells were stained with Oil-red O and some cells showed weak green fluorescent under the confocal microscope (Fig. 6).
Soft tissue defects range from the furrow of rhytid to a major loss of subcutaneous tissue secondary to congenital malformation, trauma, or extirpation. The reconstruction of such defects is the province of plastic surgery. Over the years, various methods of fat injection or graft have been used, and various materials evolved to treat such defects. However, these treatment have many limitations, such as donor-site morbidity and deformity, unsatisfactory and unpredictable results, and complications resulting from the toxicity of implants (6). For abrogating these limitations, adipose tissue engineering recently has been proposed as an alternative for current treatment (7). The choice of the types of seeding cells and scaffold used in tissue engineering determines the success of adipose tissue engineering (7).
The adipose stem cells (ASCs) may be an ideal autologous cell source for adipose tissue engineering (8). ASCs are much more resistant to mechanical damage and ischemic conditions than mature adipocytes (9). Moreover, ASCs can be readily harvested from excised human subcutaneous fat or liposuction samples, and proliferate rapidly and differentiate into mature adipocytes both in vitro and in vivo (4, 10, 11). Many studies have recently done using ASCs in adipose tissue engineering because ASCs have been demonstrated as a good cell source for adipose tissue engineering because of their high proliferation, strong differentiation, and less invasive procurement (2, 3). In the present study, as in previous studies, human ASCs were found to differentiate readily to adipocytes in monolayer (2-dimensional culture), in 3-dimensional in vitro cultures, and in vivo.
Alloderm has been used as a good scaffold for skin and oral mucosa in tissue engineering, and it has been reported to organize the arrangement of seeded keratinocytes and cultured keratinocyte sheets into three-dimensional epidermal structure that has several layers, and to recreate the original rete ridges at the interface of the dermis (12). The Alloderm has been found to produce composite oral mucosa equivalent consisting of a stratified epidermis on a dermal matrix using cultured oral keratinocytes and Alloderm (13, 14). In the present study, micronized Alloderm was used as a scaffold of ASCs for adipose tissue engineering. In the previous study, a number of different scaffolds have been investigated for adipose tissue engineering applications, e.g., polyglycolic acid (PGA) (10), poly (lactic-co-glycolic acid) (PLGA) (15), and hyaluronic acid (16). In addition, injectable materials, such as, fibrin (17), matrigel (18), and alginate gel (19) have also been studied as scaffolds for adipose tissue engineering. Injectable scaffolds can widen the scope of the engineered adipose tissue. This injectable engineered adipose tissue would be easily useful in softening wrinkles, augmenting depressed scars, and reconstructing nipples. As micronized Alloderm have been used for soft tissue augmentation of facial rhytids, scars, and deformities in injectable form (20), micronized Alloderm has succeeded to this characteristic when they would be used as a scaffold for adipose tissue engineering. In the present study, XTT cell viability testing showed that ASCs remained viable in micronized Alloderm in vitro. Moreover, these adopted a round morphology and contained large lipid vesicles after 14 days in culture, which suggests that micronized Alloderm is a suitable ASCs scaffold for adipose tissue engineering purposes.
ASC-Alloderm complexes after 2 months in vivo contained many signet-ring cells and large capillaries, whereas micronized Alloderm alone contained some fibroblast-like cells and few small capillaries. Moreover, many signet-ring cells in ASC-Alloderm complexes were stained positively by Oil-red O and also showed green fluorescence indicating that the observed differentiated adipocytes originated from injected ASC-Alloderm rather than surrounding fibrovascular tissues. Moreover, implanted ASC-Alloderm complex is softer than micronized Alloderm alone because of this adipose tissue development.
In conclusion, the present study suggests that micronized Alloderm provides a suitable scaffold for ASCs for adipose tissue engineering purpose, and that ASC-Alloderm complex has potential as a softer, more natural injectable soft tissue filler.
Figures and Tables
Fig. 1
Microscopic findings of adipose stem cells (ASCs) cultured in a monolayer for 14 days. (A) Some ASCs had differentiated into adipocytes, which contained many lipid droplets as visualized by Oil-red O staining. (B) ASCs were well labeled with PHK67 green fluorescent dye (×400).
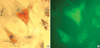
Fig. 2
Changes in ASCs viabilities over 14 days in culture. ASCs were cultured in vitro after mixing then with micronized Alloderm (5×104 cells/1 mg of Alloderm). Cell viabilities were determined using XTT colorimetric assays. Values represent mean±standard deviation (error bar).
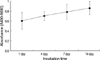
Fig. 3
Microscopic findings of in vitro cultured ASC-Alloderm complex for 14 days. ASCs in micronized Alloderm differentiated to round cells (arrows) with large lipid vesicles (H&E stain, ×400).
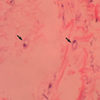
Fig. 4
Scanning microscopic findings after 14 day of in vitro culture. (A) Micronized Alloderm alone. (B) ASC-Alloderm complex. Many cells were found to cover the micronized Alloderm, and some spherical adipocyte-like cells were observed.
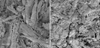
References
2. Rodriguez AM, Elabd C, Delteil F, Astier J, Vernochet C, Saint-Marc P, Guesnet J, Guezennec A, Amri EZ, Dani C, Ailhaud G. Adipocyte differentiation of multipotent cells established from human adipose tissue. Biochem Biophys Res Commun. 2004. 315:255–263.


3. Hong L, Peptan IA, Colpan A, Daw JL. Adipose tissue engineering by human adipose-derived stromal cells. Cells Tissues Organs. 2006. 183:133–140.


4. Zuk PA, Zhu M, Mizuno H, Huang J, Futrell JW, Katz AJ, Benhaim P, Lorenz HP, Hedrick MH. Multilineage cells from human adipose tissue: implications for cell-based therapies. Tissue Eng. 2001. 7:211–228.


5. Fraser JK, Wulur I, Alfonso Z, Hedrick MH. Fat tissue: an underappreciated source of stem cells for biotechnology. Trends Biotechnol. 2006. 24:150–154.


6. Billings E Jr, May JW Jr. Historical review and present status of free fat graft autotransplantation in plastic and reconstructive surgery. Plast Reconstr Surg. 1989. 83:368–381.


7. Patrick CW Jr. Tissue engineering strategies for adipose tissue repair. Anat Rec. 2001. 263:361–366.


8. De Ugarte DA, Ashjian PH, Elbarbary A, Hedrick MH. Future of fat as raw material for tissue regeneration. Ann Plast Surg. 2003. 50:215–219.


9. von Heimburg D, Hemmrich K, Zachariah S, Staiger H, Pallua N. Oxygen consumption in undifferentiated versus differentiated adipogenic mesenchymal precursor cells. Respir Physiol Neurobiol. 2005. 146:107–116.
10. Cho SW, Kim SS, Rhie JW, Cho HM, Choi CY, Kim BS. Engineering of volume-stable adipose tissues. Biomaterials. 2005. 26:3577–3585.


11. Zuk PA, Zhu M, Ashjian P, De Ugarte DA, Huang JI, Mizuno H, Alfonso ZC, Fraser JK, Benhaim P, Hedrick MH. Human adipose tissue is a source of multipotent stem cells. Mol Biol Cell. 2002. 13:4279–4295.


12. Hickerson WL, Compton C, Fletchall S, Smith LR. Cultured epidermal autografts and allodermis combination for permanent burn wound coverage. Burns. 1994. 20:Suppl 1. S52–S55.


13. Izumi K, Takacs G, Terashi H, Feinberg SE. Ex vivo development of a composite human oral mucosal equivalent. J Oral Maxillofac Surg. 1999. 57:571–577.


14. Izumi K, Terashi H, Marcelo CL, Feinberg SE. Development and characterization of a tissue-engineered human oral mucosa equivalent produced in a serum-free culture system. J Dent Res. 2000. 79:798–805.


15. Patrick CW Jr, Chauvin PB, Hobley J, Reece GP. Preadipocyte seeded PLGA scaffolds for adipose tissue engineering. Tissue Eng. 1999. 5:139–151.


16. Halbleib M, Skurk T, de Luca C, von Heimburg D, Hauner H. Tissue engineering of white adipose tissue using hyaluronic acid-based scaffolds. I: in vitro differentiation of human adipocyte precursor cells on scaffolds. Biomaterials. 2003. 24:3125–3132.


17. Schoeller T, Lille S, Wechselberger G, Otto A, Mowlavi A, Piza-Katzer H, Mowlawi A. Histomorphologic and volumetric analysis of implanted autologous preadipocyte cultures suspended in fibrin glue: a potential new source for tissue augmentation. Aesthetic Plast Surg. 2001. 25:57–63.


18. Kawaguchi N, Toriyama K, Nicodemou-Lena E, Inou K, Torii S, Kitagawa Y. De novo adipogenesis in mice at the site of injection of basement membrane and basic fibroblast growth factor. Proc Natl Acad Sci USA. 1998. 95:1062–1066.


19. Yoo G, Yea BH, Rhie JW, Kwon H, Wee SS, Ahn ST. Growth and Differentiation of Preadipocytes in Alginate and Collagen Gels. J Korean Soc Plast Reconstr Surg. 2000. 27:386–392.