Abstract
Hippo/YAP signaling hinders cancer progression. Inactivation of this pathway contributes to the development of esophageal cancer by activation of Akt. However, the possible interaction between Akt and Hippo/YAP pathways in esophageal cancer progression is unclear. In this study, we found that ursolic acid (UA) plus 3′3-diindolylmethane (DIM) efficiently suppressed the oncogenic Akt/Gsk-3β signaling pathway while activating the Hippo tumor suppressor pathway in esophageal cancer cells. Moreover, the addition of the Akt inhibitor LY294002 and the PI3K inhibitor 3-methyladenine enhanced the inhibitory effects of UA plus DIM on Akt pathway activation and further stimulated the Hippo pathway, including the suppression of YAP nuclear translocation in esophageal cancer cells. Silencing YAP under UA plus DIM conditions significantly increased the activation of the tumor suppressor PTEN in esophageal cancer cells, while decreasing p-Akt activation, indicating that the Akt signaling pathway could be down-regulated in esophageal cancer cells by targeting PTEN. Furthermore, in a xenograft nude mice model, UA plus DIM treatment effectively diminished esophageal tumors by inactivating the Akt pathway and stimulating the Hippo signaling pathway. Thus, our study highlights a feedback loop between the PI3K/Akt and Hippo signaling pathways in esophageal cancer cells, implying that a low dose of UA plus DIM could serve as a promising chemotherapeutic combination strategy in the treatment of esophageal cancer.
Esophageal cancer is a malignant disease with the eighth highest cancer incidence worldwide, with more than 500,000 new cases in 2020 [1]. Mortality from esophageal cancer is the sixth highest of any cancer worldwide [2]. In Republic of Korea and some other East Asian countries, the predominant type of esophageal cancer, at almost 90%, is esophageal squamous cell carcinoma (ESCC) [3,4]. ESCC is derived from esophageal squamous epithelium. After the esophageal mucosa is exposed to carcinogenic factors, epithelial cells start to proliferate abnormally, finally developing into ESCC [5]. Due to unapparent early symptoms, most patients are diagnosed with esophageal cancer at an advanced stage [6]. Chemotherapy and radiotherapy are the major treatments for patients with advanced esophageal cancer [7]. Unfortunately, chemotherapeutic resistance and adverse events are the main reason for therapeutic failure in esophageal cancer [7-9]. Hence, there is an urgency to develop novel drugs. Previous studies have shown that increasing the intake of fruits and vegetables negatively correlates with the risk of esophageal cancer [10,11]. Therefore, identification of effective compounds from vegetables and other organic matter may offer an opportunity to develop a new drug for esophageal cancer.
Ursolic acid (UA) (3-β-hydroxy-urs-12-en-28-oic acid) is a ubiquitous natural product that can be identified in many plants and has various antitumor [12-15], anti-inflammatory [16], antioxidant [17,18], and antibacterial [18,19] properties. UA has been found to regulate multiple signaling pathways such as those in cell growth, cell death, apoptosis, metastasis, autophagy, and reactive oxygen species in esophageal cancer [20], lung cancer [21-23], gastric cancer [24,25], liver cancer [26,27], and prostate cancer [14,28]. Our recent research has demonstrated that UA can suppress esophageal cancer cell growth, metastasis, and autophagy [20]. 3′3-Diindolylmethane (DIM), a dimeric product of indole-2-carbinol in cruciferous vegetables, may have anti-tumor effects in gastric cancer [29-31], esophageal cancer [32,33], colon cancer [34], prostate cancer [34,35], and breast cancer [36] by contributing to multiple signaling pathways. Our previous study clarified that DIM inhibited ESCC tumorigenesis in a dose-dependent manner [33]. Although UA and DIM have shown excellent anti-tumor effects, drug toxicity is a concern. For drug safety and feasibility reasons, we aimed to explore combined low-dose administration of UA and DIM and studied their effect on signaling mechanisms in ESCC cells. We clarified that UA plus DIM was more effective in restraining cell growth, invasion, proliferation, and migration and further stimulated cell apoptosis and prompt G1 cell cycle arrest in ESCC. Furthermore, in experiments in vivo, combined treatment with UA and DIM safely and successfully inhibited tumorigenesis in an esophageal cancer nude mouse xenograft model.
The Hippo and PI3K/Akt signaling pathways are essential in regulating cell growth in esophageal cancer cells [37,38]. Activating the oncogenic PI3K/Akt signaling pathway promotes cell growth, proliferation, and invasion and regulates various downstream pathways in esophageal cancer cells [39,40]. On the contrary, as a tumor suppressor signaling pathway, the Hippo pathway is activated, in which phosphorylated Mst1/2 activates LATS1/2 together with SAV and Mst1/2, phosphorylating and inhibiting YAP/TAZ cytoplasm translocation to further inhibit downstream genes such as CTGF [41-43]. These two signaling pathways were found to have a joint effect [34,44-46]. Some studies have posited that the Hippo signaling system, an upstream pathway in various cells, could be negatively regulated by the PI3K/Akt signaling pathway [34,46-48]. Additionally, it has been discovered that YAP, a core molecule of the Hippo signaling pathway, plays an important role in the interaction between the Hippo and PI3K/Akt signaling pathways [45,47,49]. PTEN, a tumor suppressor upstream of Akt, is considered to be targeted by YAP in a Hippo-Akt feedback loop [44,50,51]. Our present study found that UA and DIM combination treatment activated the Hippo signaling pathway through inhibition of YAP, a core molecule in Hippo signaling, and down-regulated the PI3K/Akt signaling pathway by targeting PTEN in ESCC both in vivo and in vitro. This suggests that UA plus DIM stimulated a feedback loop between the PI3K/Akt signaling pathway and Hippo signaling pathway in ESCC. Thus, our present research suggests that a low UA plus DIM combination dose could function as a new and safe chemotherapy strategy in ESCC.
The esophageal cancer cell lines TE-8 and TE-12 were obtained from Dr. Izzo (University of Texas MD Anderson Cancer Center) and were grown in DMEM-F12 media (Gibco) with FBS (Welgene) and penicillin-streptomycin (Sigma). Primary antibodies against YAP, Mst1, Mst2, caspase-9, c-caspase-9, p-YAP, PARP, c-PARP, Akt, p-Akt, Mob-1, p-Mob1, p-GSK-3β, E-cadherin, MMP-9, CDK4, CDK6, cyclin D1, Sav1, p-PTEN, PTEN, and GAPDH were obtained from Cell Signaling Technology, and primary antibodies against MMP-13, Rassf1, and CTGF were purchased from Santa Cruz Biotechnology Inc. UA was obtained from Cayman Chemical Company, and DIM was purchased from LKT Laboratories. 3-Methyladenine (3-MA) and LY294002 were obtained from Sigma Chemicals and Cell Signaling Technology, respectively. Both were dissolved in dimethyl sulfoxide (DMSO) (Sigma Chemical Co.).
Esophageal cancer cells TE-8 and TE-12 (104/well) were seeded in 96-well plates, and UA 20 μM was added with or without DIM (50 μM) for 48 h. The WST-1 assay was conducted using an EZ-CYTOX kit according to the manufacturer’s manual (DoGenBio). Briefly, 10 μl EZ-CYTOX was diluted in 100 μl RPMI-1640 per well and shaken for 5 min in the dark. Cells were cultured for 2 h at 37°C, and the absorbance (OD) was measured at 450 nM using a model Epoch microplate reader (Bio Tek).
A layer of soft agarose (1%) was added to the bottom of six-well plates and left to solidify. Cells (5 × 104 cells/well) were added to the top layer of soft agarose (0.7%) with or without UA 20 μM or/and DIM 50 μM, respectively. Following the culture of cells at 37°C with 5% CO2 for 4 weeks, colonies of ≥ 30 cells were counted with a microscope.
After drug treatment, extracts of esophageal cancer cells (TE-8 and TE-12) were prepared in RIPA lysis buffer (Thermo Fisher Scientific). The cell pellets were vortexed for 30 min and then centrifuged for 20 min at 13,200 rpm at 4°C to obtain protein extracts. An equal volume of protein sample was loaded onto 6%–15% SDS-PAGE and then transferred to PVDF membranes (Bio-Rad). After blocking with milk at room temperature for 1 h, blots were incubated at 4°C overnight with specific primary antibodies against caspase-9/c-caspase-9, PARP/c-PARP, AKT/p-Akt, Gsk-3β/p-Gsk-3β, E-cadherin, MMP-9, CDK4, CDK6, cyclin D1, Yap, p-Yap, Mst1, Mst2, Sav1, Mob-1, p-Mob1, MMP-13, Rassf1, PTEN, p-PTEN, CTGF, and GAPDH and incubated with peroxidase-conjugated secondary antibodies. All antibodies were diluted at a ratio of 1:1,000. Images of Western blots were obtained using an improved chemiluminescence kit (Amersham). Bands were quantified through ImageJ software.
For cell cycle analysis, after 48 h treatment with UA (20 μM) or/and DIM (50 μM), TE-8 and TE-12 cells were collected, rinsed twice with cold DPBS, and fixed with 75% ethanol for 2 h at –20°C. Fixed cells were re-suspended in DPBS and stained with propidium iodide (PI, Sigma) for 30 min. For FITC/Annexin V staining analysis, cells were stained using a FITC Annexin V Apoptosis Detection Kit II (Becton-Dickinson Biosciences) at 37°C for 30 min. Stained cells were examined by a FACStar flow cytometer (Becton-Dickinson) and analyzed with Becton-Dickinson software.
UA (20 μM) or/and DIM (50 μM) were used to culture TE-8 and TE-12 esophageal cancer cells, and Trizol was used to extract the total RNA (Ambion by Life Technologies, Thermo Fisher Scientific). A PrimeScript RT reagent kit was used to synthesize cDNA from 1 g of total RNA (Takara Bio). Quantitative real-time PCR was carried out with SYBR Premix Ex Taq (Takara Bio) and an ABI Prism 7900 Sequence Detection System (Applied Biosystems). Primer sequences were as follows: CTGF sense, 5′-CGACTGGAAGACACGTTTGG-3′ and antisense, 5′-AGGCTTGGAGATTTTGGGAGA-3′; GAPDH sense, 5′-GTCTCCTC TGACTTCAACAGCG-3′ and antisense, 5′-ACCACCCTGTT GCTGTAGCCAA-3′. All results were analyzed with the ΔΔCt method.
TE-8 and TE-12 cells (3 × 105/well) were seeded into 24-well plates and allowed to reach 80%–90% confluence in the presence of 5 μg/ml mitomycin-C (Sigma-Aldrich). A wound was scratched on the surface of cells with a 200 μl pipette tip. Cells were further cultured with 1% penicillin without fetal bovine serum. The average distance of wound invasion was detected at the indicated time point and the migration rate was calculated.
TE-8 and TE-12 cells were grown to 70% confluence. Reagent A was prepared as 75 pmol YAP siRNA or control siRNA (Santa Cruz) in 125 μl opti-MEM (Gibco), and reagent B was 7.5 μl lipofectamine 3000 (Invitrogen) in 125 μl opti-MEM. These reagents were gently incubated for 15 min at room temperature. The mixture (250 μl) was added to 750 μl opti-MEM, plated with cells, and swirled to mix. After 6 h, the DMEM was changed. After 24 h, UA 20 μM and DIM 50 μM were added, and cells were incubated for 48 h. Cells were then collected for the following experiments.
Cell cytoplasmic and nuclear fractions were separated using an NE-PER kit (Thermo Fisher Scientific). After drug treatment, cells were gently collected in an EP tube and rinsed with DPBS once. CERI reagent was added, and cells were placed on ice for 10 min. Next, CERII reagent was added, and the cells were incubated for 1 min on ice and centrifuged at 13,200 rpm in 4°C for 5 min. The supernatant (cytoplasm protein) was collected in a new EP tube. The insoluble pellet was washed twice with DPBS, and NER reagent was added. Cells were put on ice for 40 min and centrifuged at 13,200 rpm at 4°C for 20 min. The supernatant (nuclear protein) was transferred into a new EP tube. Proteins were stored at –80°C or used directly.
TE-8 and TE-12 cells were seeded onto glass coverslips and further treated with LY294002 with or without UA (20 μM) plus DIM (50 μM) for 48 h in a six-well plate. Cells were rinsed with DPBS twice and fixed with 4% methanol in DPBS for 40 min. Then, 0.1% Triton X-100 (Sigma-Aldrich) in DBPS was added for 10 min for permeabilization. After blocking, anti-YAP antibody (dilution 1:100) was added to cover glasses and stored at 4°C overnight. Cover glasses were rinsed, and rabbit secondary antibody (dilution 1:100) was added at room temperature for 1 h in the dark. Cells were stained with DAPI (dilution 1:500) for 5 min at room temperature in the dark. Cover glasses were attached to microscope slides (Paul Marienfeld GmbH & Co. KG) with mounting solution (Vector Laboratories, Inc.), and the edges were fixed with Canada balsam (Junsei Chemical Co.). Images were obtained under a fluorescence microscope (Zeiss Model Axio Imager M1). Fluorescence values were detected and merged using ImageJ.
The esophageal cancer xenograft model was established under the approval of the Institutional Animal Care and Use Committee (IACUC#CBNU2017-0001, 3 January 2017) of Jeonbuk National University under NIH guidelines (USA). TE-8 cells (1.5 × 107) in 100 μl of Matrigel (BD Bioscience) were inoculated subcutaneously into the flanks of five-week-old SPF/VAF immunodeficient mice, which were purchased from Orient Bio. Four groups of five mice per group were established after tumor implantation: i) vehicle group (i.p. injected with 10 mg/kg DMSO in 50 μl DPBS every day), ii) UA only-treatment group (i.p. injected with 10 mg/kg in 50 μl DPBS every day), iii) DIM only-treatment group (i.p. injected with 10 mg/kg in 50 μl DPBS every two days), and iv) DIM and UA combination treatment group (i.p. injected with DIM 10 mg/kg in 50 μl PBS every two days and UA 10 mg/kg in 50 μl PBS every day). Mouse body weight and tumor size were measured every three days. The formula for measuring tumor volume was (width)2 × length/2. When tumor volume reached 4,000 mm3, mice were euthanized, and the tumor was weighed. The samples were stored in formalin for staining, and the samples for Western blotting were stored at −80°C.
Mouse blood was collected after euthanasia, and the serum was obtained. Biochemical parameters of alanine aminotransferase (ALT, Asan Pharmaceutical), aspartate aminotransferase (AST, Asan Pharmaceutical), blood urea nitrogen (BUN, BUN Serum Detection Kit; Arbor Assays), and creatinine (Creatinine Serum Detection Kit; Arbor Assays) were detected according to the manufacturer's manual.
Tissue specimens were fixed with 10% formalin immediately after being removed from mice. Specimens were decalcified in Calci-Clear Rapid histological decalcifying agent (National Diagnostics) for 72 h and then embedded in paraffin. Sections were cut to 4 μm thickness and covered with H&E solution. Images were obtained under a microscope.
The sections were obtained as mentioned above. Sections were incubated in anti-Ki67 antibody (Invitrogen) after de-paraffining and dehydrating. On the next day, sections were washed and probed with an anti-rabbit HRP/DAB IHC kit (Abcam) for 2 h. Images were taken under a microscope.
Each experiment was carried out at least three times. The mean and standard error of the mean are used to express data. Treated cells and their corresponding controls were compared utilizing the GraphPad Prism software (version 6.0; GraphPad Software, Inc.). Data were analyzed using one-way analysis of variance and Dunn's least significant difference tests. The threshold for statistical significance was set at p < 0.05.
WST cell viability and soft agar colony formation assays were used to investigate the role of UA + DIM combination treatment on esophageal cancer cell proliferation. The combination treatment decreased the viability of TE-8 and TE-12 cells more markedly than UA (20 μM) or DIM (50 μM) treatment alone (Fig. 1A and Supplementary Fig. 1). Colony formation assay results revealed the same tendency. Compared to the UA or DIM single treatment groups, the combined treatment group has a much lower number of colonies (Fig. 1B). Therefore, these results suggested that UA plus DIM treatment inhibited cell proliferation and cell growth in ESCC more significantly. The cell distribution and protein expression of apoptosis-regulator factors in TE-8 and TE-12 cell lines were assessed by flow cytometry and Western blot assays to ascertain if the combination of UA and DIM triggered cell apoptosis. After the combined treatment of UA plus DIM, the distribution of sub-G1 phase cell percentage was significantly higher than those treated with UA or DIM alone (Fig. 1C). Caspase 9 and PARP protein levels were noticeably lower in the combination group compared to cleaved-caspase 9 and cleaved-PARP protein levels, which were both considerably higher (Fig. 1D). Annexin V/PI staining shown that UA plus DIM markedly induced both early-stage and late-stage apoptosis cell accumulation compared to UA or DIM single treatment (Fig. 1E). Thus, our results demonstrate that the UA plus DIM combination treatment can induce ESCC cell apoptosis more effectively than UA or DIM single drug treatment.
FACS analysis with PI staining was used to examine whether the UA plus DIM combination treatment affected the cell cycle in ESCC cells. In contrast to the single-agent treatment, UA (20 μM) plus DIM (50 μM) combination treatment induced significant G1 phase arrest in TE-8 and TE-12 cells (Fig. 2A). To further confirm the G1 phase arrest, we used Western blot analysis to measure G1 phase-related proteins CDK4, CDK6, and cyclin D1. The protein levels of CDK4, CDK6, and cyclin D1 in the combination treatment group declined more markedly than in the UA or DIM groups (Fig. 2B). Thus, our results suggested that UA plus DIM more strongly caused G1 phase arrest in ESCC cells.
To explore the effect of UA plus DIM in cell migration in ESCC cells, we detected the migration rate by wound healing assay in TE-8 and TE-12 cells. Fig. 3A shows that at both 12 h and 24 h, the migration rate of the combination group is the lowest, which indicates that the combination treatment group more dramatically suppressed migration ability. To explore the inhibitory impact of UA and DIM on cell migration at the molecular level, migration-related proteins were detected by Western blot analysis. The E-cadherin protein level notably increased whereas MMP-9 and MMP-13 protein levels markedly decreased in the combination group compared with the UA or DIM treated group (as shown in Fig. 3B) These results showed that UA plus DIM combination treatment significantly suppressed the migration ability of ESCC cells compared with UA or DIM treatment alone.
Western blot analysis was used to explore alterations in Akt/phosphorylated-Akt proteins and its downstream protein GSK-3/phosphorylated-GSK-3 to investigate the function of UA plus DIM on the PI3K/Akt signaling pathway. As shown in Fig. 4, combination treatment with UA and DIM markedly reduced protein expression of phosphorylated-Akt and phosphorylated-GSK-3β compared to those treated with UA or DIM alone. These findings suggest that UA plus DIM combination more effectively inactivates the PI3K/Akt/GSK-3β signaling pathway which leads to the suppression of tumorigenesis in ESCC cells.
To evaluate how the combination of UA plus DIM works on the Hippo signaling pathway in ESCC, Western blot analysis was performed to check for alterations in the Hippo signaling pathway. Levels of Hippo signaling pathway proteins Rassf1, Mst1, Mst2, Mob-1, p-Mob1, and Sav1 increased in the combination treatment group compared to the single-agent groups (Fig. 5A). On the other hand, levels of YAP, a critical protein that phosphorylated and inhibited in the Hippo pathway, significantly decreased (Fig. 5B), whereas phosphorylated-YAP notably increased in the combination treatment group compared with the groups treated with UA or DIM alone. We then performed real-time PCR and Western blot analysis to investigate the CTGF (the direct downstream target gene of YAP) regulation by UA and DIM treatment in TE-8 and TE-12 cells. The combination treatment group's mRNA level (C) and protein level (D) of CTGF were significantly lower than those of the single treatment group (Fig. 5). These findings suggested that the combination of UA and DIM inhibited the growth of esophageal cancer cells by targeting YAP inhibition that results in CTGF expression in ESCC. This activation of the Hippo signaling pathway was thought to be the mechanism by which the combination treatment of UA and DIM inhibited esophageal cancer cell growth.
The PI3K/Akt signaling and the Hippo signaling pathways are crucial for mediating cell proliferation, and previous research has demonstrated that the PI3K/Akt signaling system can suppress the Hippo signaling pathway [47,49,50]. Our study revealed that UA plus DIM inhibited the Akt signaling pathway (Fig. 4) and stimulated the Hippo signaling pathway (Fig. 5) in ESCC cells. To further explore whether the Hippo pathway can be affected by the PI3K/Akt signaling pathway under UA plus DIM combination treatment, we used Akt inhibitor LY294002 and PI3K inhibitor 3-MA in TE-8 and TE-12 cells. As seen in Fig. 6A and B, CTGF levels were greatly reduced by the combination treatment while the expression of Hippo signaling genes (Rassf1, Mst1, Mst2, Sav1, and Mob1) and p-YAP were markedly accelerated and raised by LY294002 or 3-MA. Nuclear translocation of YAP activates a downstream target gene as the key step in the Hippo signaling pathway that mediates tumor growth [41,42,52], we analyzed the effect of co-treatment with the inhibitors and UA plus DIM on YAP nucleus translocation. As shown in Fig. 6C and D, YAP nucleus translocation was more significantly inhibited in the UA plus DIM group co-treated with LY294002 or 3-MA than in the other group. These findings showed that the inhibition of the PI3K/Akt signaling pathway further promoted Hippo pathway activation after UA plus DIM treatment in ESCC cells.
Our present data demonstrate that UA plus DIM combination treatment effectively activated the Hippo signaling pathway, including YAP nuclear translocation suppression, by suppressing the Akt signaling pathway in ESCC. However, it is still unclear whether the Hippo signaling pathway can regulate the PI3K/Akt signaling pathway in ESCC. Some studies have found that YAP exerts a vital function in regulating PI3K/Akt signaling pathway through the Hippo signaling pathway [34,45,47]. Moreover, a latest study has reported that the YAP-regulated PI3K/Akt/mTOR pathway is significantly impacted by PTEN, a tumor suppressor that negatively regulates the PI3K/Akt pathway [45,51,52]. Thus, we hypothesized that YAP could restrain PI3K/Akt signaling pathway activation through PTEN in the presence of UA plus DIM in TE-8 and TE-12 cell lines. To verify our hypothesis, we carried out Western blot analysis to measure Akt/p-Akt and PTEN/p-PTEN after YAP siRNA knockdown with or without the presence of UA plus DIM combination treatment. Fig. 7 shows that the knockdown of YAP, a negative regulator of Akt, improved PTEN expression and decreased p-PTEN levels, indicating that the knockdown of YAP activated PTEN in TE-8 and TE-12 cells. Furthermore, the levels of p-Akt were lower. UA plus DIM further induced PTEN expression in the YAP siRNA treated-combination treatment group, whereas p-Akt expression was more significantly decreased. These data suggested that UA plus DIM induced YAP inhibition can down-regulate the PI3K/Akt signaling pathway by targeting PTEN in ESCC.
We used an esophageal cancer xenograft nude mouse model to discover how the combination therapy of UA and DIM affects ESCC tumorigenesis. The mice were divided into four groups at random once the esophageal cancer xenograft mouse model had been established: the control group, the UA group, the DIM group, and the UA + DIM combination group. After 3 weeks of drug treatment, the maximum tumor growth reached 4,000 mm3 and mice were sacrificed. To evaluate drug safety in the mouse model (Fig. 8A), we measured serum biochemical markers, including liver function markers ALT and AST and renal function markers BUN and creatinine. All serum biochemical markers showed no significant difference between each group. In addition, there was no significant difference in body weight between each group of mice presented in Fig. 8B. As shown in Fig. 8C–E, UA plus DIM combination treatment group shows a greater inhibitory effect on tumor volume (C), tumor weight (D), and tumor size (E). These results indicated that the UA and DIM combination treatment safely and effectively inhibited tumorigenesis in the esophageal cancer xenograft model. As shown in Fig. 8F, H&E staining showed poorly differentiated carcinoma without clear-cut squamous differentiation in the control group, whereas augmented ranges of necrosis/apoptosis with penetrated lymphocytes were observed in the combination of UA and DIM treatment group. Ki67 IHC staining result showed fewer positive cells in the combination treatment group. These results indicated that UA plus DIM combination treatment inhibited more tumor proliferation and differentiation compared to the single treatment in vivo. We further investigated the alteration of the Akt and Hippo pathway after drug treatment by Western blot analysis in mouse tumor tissue. Similar to the in vitro results, the UA plus DIM combination group suppressed p-Akt levels (Fig. 8G) and activated the Hippo signaling pathway (Fig. 8H) in vivo. These results suggest that the UA plus DIM combination treatment inhibited tumorigenesis safely and effectively through the Akt and Hippo signaling pathways in the esophageal cancer xenograft mouse model.
Although diagnosis and current therapies have improved, the incidence of esophageal cancer and mortality remain high worldwide [1,2]. Poor diagnosis and ambiguous early symptoms result in many patients being diagnosed at an advanced stage [5]. Chemotherapeutic resistance and adverse events have become the main reason for the therapeutic failure of the two primary treatments in advanced esophageal cancer, chemotherapy and radiotherapy [7-9,53]. Thus, developing novel drugs for esophageal cancer therapy is required urgently. Numerous studies show a negative correlation between esophageal cancer risk and increased fruit and vegetable intake [11,12]. Hence, finding effective ingredients from vegetables and other organic matters may offer opportunities to develop a new drug for esophageal cancer. Our current study demonstrated that the combination of two natural extracts, UA and DIM, safely and effectively inhibited tumorigenesis in ESCC by regulating the PI3K/Akt signaling pathway and Hippo signaling pathway in vitro and in vivo.
Our previous study revealed that the IC50 of UA in TE-8 and TE-12 cells was 31.66 ± 0.52 and 34.48 ± 0.75, respectively [54]. In contrast, DIM, even with a high concentration of 100 μM, only resulted in 65% cell death in another of our previous investigations [33]. Moreover, DIM concentrations vary widely among cancer types [55], and both DIM and UA face limitations in their individual use due to factors like drug resistance and toxicity. Therefore, a combination of these two drugs may offer potential benefit. Both UA and DIM are natural products derived from various plants, and previous studies have demonstrated their abilities to inhibit cell growth, invasion, and migration, as well as to induce cell apoptosis and G1 phase arrest in several forms of cancer, including esophageal cancer [22-24,30,33,34,56,57]. Similarly, our data showed that cell viability and colony formation were decreased after treatment with UA or DIM alone. However, combination treatment of UA and DIM resulted in an even greater significant reduction in these factors in TE-8 and TE-12 cells. As an integral part of many chemotherapy targets, apoptosis plays an vital role in treating cancer [58]. UA and DIM dose-dependently accelerated esophageal cancer cell apoptosis in our study published previously [20,33]. Our flow cytometry assay results revealed that UA plus DIM more markedly induced subG1 phase cells (Fig. 1C) and both early-stage and late-stage apoptosis cells (Fig. 1E) than UA or DIM alone. The combination group had higher levels of the apoptotic marker proteins cleaved-PARP and cleaved-caspase-9 as measured by Western blot analysis (Fig. 1D). Additionally, although UA and DIM single drug treatment induced G1 cell arrest, combination treatment stimulated more G1 cycle cell accumulation. G1 cell cycle-related proteins (CDK4, CDK6, and Cyclin D1) measured by Western blotting also showed the same tendency (Fig. 2). Distant metastasis is considered to rely on cancer cell migration and is closely related to advanced stages of esophageal cancer [59,60]. The single reagent treatment with high concentrations of UA and DIM showed effective migration suppression in esophageal cancer [20,32]. Our wound healing assay results and the alteration of epithelial-mesenchymal transition (EMT)-related proteins E-cadherin, MMP-9, and MMP-13 showed that a low dose of UA and DIM combination treatment has a more effective suppression of migration in ESCC. Therefore, our current research indicated that UA plus DIM considerably inhibited cell growth, proliferation, invasion, and migration more than UA or DIM single drug treatment in ESCC.
PI3K/Akt is a well-known oncogenic pathway that contributes to various cellular processes resulting in tumor occurrence and progression [61]. According to Shin et al. [60], activation of the PI3K/Akt signaling pathway may promote the cell cycle-dependent proliferation of cancer cells. Additionally, Datta et al. [61] discovered that the PI3K/Akt signaling pathway might reduce apoptosis in certain cancer cells. The PI3K/Akt signaling pathway was also discovered to dysregulate migration that was dependent on cancer cell EMT in numerous investigations [62,63]. Glycogen synthase kinase‐3β (GSK-3β), a primary downstream gene of the PI3K/Akt signaling pathway, participates closely in cell growth, apoptosis, and migration and is also involved in drug resistance [62,63]. In addition, DIM may also inhibit colon cancer cell growth and proliferation by down-regulating the Akt/PTEN signaling pathway [34]. In line with prior research, our study revealed that combination treatment of UA and DIM significantly reduced the expression of p-Akt and p-GSK-3β in ESCC compared to using each reagent individually. Furthermore, the anticancer activity of UA and DIM in ESCC was amplified more when used together than when used alone.
The Hippo pathway is central for organ growth and cell proliferation. Hippo signaling functions are a positive characteristic in blocking cancer progression [37]. Under Hippo pathway activation, phosphorylated Mst1/2 activates LATS1/2 together with SAV and Mst1/2, thereby phosphorylating YAP/TAZ, leading to YAP/TAZ cytoplasm translocation and the inhibition of the downstream gene CTGF [39-44]. Previous studies have reported that either UA or DIM could interrupt gastric cancer tumorigenesis by stimulating the Hippo signaling pathway [24,30,34]. Our present results demonstrate that the Hippo signaling pathway-related proteins Rassf1, Mst1, Mst2, Mob-1, p-Mob1, and Sav1 dramatically increased in the UA and DIM combination treatment compared to those of the single treatment. In addition, the expression of YAP, a core molecule in the Hippo signaling pathway, was significantly lowered whereas phosphorylated-YAP expression was notably enhanced in the combination treatment group. Similar to previous research, the protein and mRNA levels of CTGF, a direct downstream target gene of YAP, were likewise significantly reduced in the combination treatment group than in the single treatment group. These results indicate that UA and DIM combination therapy activates the Hippo signaling pathway and thereby inactivates downstream genes such as YAP and CTGF more than the single treatments.
The Hippo and PI3K/Akt signaling pathways are gaining increasing attention due to their pivotal roles in regulating cell proliferation, and there is growing evidence of interactive effects between these two pathways [34,44,45,47,49]. According to Hu et al. [48] reported, the Hippo pathway serves as a downstream target of Akt signaling in primordial follicles. Ye et al. [46] found that Akt signaling negatively regulates the Hippo pathway in Drosophila. Recent research has shown that YAP has an important role in the interaction between the Hippo and PI3K/Akt signaling pathways [44,45]. Based on these previous studies, we determined whether the Hippo signaling system is modulated by the PI3K/Akt pathway through combination therapy of UA and DIM. The present data showed that the Akt inhibitor LY294002 or PI3K inhibitor 3-MA further enhanced the activation of the Hippo signaling pathway when used in combination with UA and DIM therapy. This combination treatment also significantly inhibited YAP nuclear translocation. Additionally, the Akt inhibitors lead to a notable reduction in CTGF expression in ESCC. The results of the present study demonstrate an association between the Akt and Hippo signaling pathways, and their combined treatment activated the Hippo signaling pathways by suppressing the Akt pathway in ESCC. These findings suggest that UA combined with DIM can be a potential treatment strategy for ESCC. A previous study in our laboratory proved that DIM inhibited colon cancer growth via Akt and YAP inhibition [34]. Moreover, PTEN, an upstream tumor suppressor of Akt, is considered to be targeted by YAP in a Hippo-Akt feedback loop [44,50,51]. Tumaneng et al. [44] shown that PTEN is involved in the YAP-regulated PI3K/Akt/mTOR pathway. Qian et al. [45] also found that YAP activated the PI3K/Akt pathway through PTEN and PI3K/Akt finally inhibiting the Hippo pathway in glomerular mesangial cells. Additionally, research in vivo indicated that the YAP and TEAD complex induced PI3K transcription and further promoted PI3K/Akt pathway to enhance myocardial cell growth in mice [48,49,64]. In our present data, knockdown of YAP inhibited p-Akt protein expression and increased PTEN protein expression in ESCC. In the presence of UA and DIM, YAP interference induced more inhibition of p-Akt and an increase of PTEN, indicating that UA plus DIM combination treatment can stimulate the PI3K/Akt signaling pathway and induce Hippo signaling pathway activation through the inhibition of the core molecule YAP and conversely down-regulate Akt signaling pathway by targeting PTEN in ESCC. Furthermore, in vivo experiments shows that UA and DIM combination treatment safely and successfully inhibited tumor genesis through the PI3K/Akt signaling pathway and Hippo signaling pathway in an esophageal cancer nude mouse xenograft model.
In summary, we demonstrated that the interaction of the PI3K/Akt pathway and the Hippo signaling system in esophageal cancer cells in vitro and in vivo safely and effectively decreased cell proliferation and migration, induced apoptosis, and suppressed tumorigenesis (Fig. 9). We discovered that in ESCC, the combination of UA and DIM induced the feedback loop between the PI3K/Akt signaling pathway and the Hippo signaling pathways. Consequently, a low dose of UA combined with DIM may be a possible chemotherapeutic approach in ESCC.
Notes
SUPPLEMENTARY MATERIALS
Supplementary data including one figure can be found with this article online at https://doi.org/10.4196/kjpp.2023.27.5.493
REFERENCES
1. Uhlenhopp DJ, Then EO, Sunkara T, Gaduputi V. 2020; Epidemiology of esophageal cancer: update in global trends, etiology and risk factors. Clin J Gastroenterol. 13:1010–1021. DOI: 10.1007/s12328-020-01237-x. PMID: 32965635.


2. Then EO, Lopez M, Saleem S, Gayam V, Sunkara T, Culliford A, Gaduputi V. 2020; Esophageal cancer: an updated surveillance epidemiology and end results database analysis. World J Oncol. 11:55–64. DOI: 10.14740/wjon1254. PMID: 32284773. PMCID: PMC7141161.


3. Shin A, Won YJ, Jung HK, Kong HJ, Jung KW, Oh CM, Choe S, Lee J. 2018; Trends in incidence and survival of esophageal cancer in Korea: analysis of the Korea Central Cancer Registry Database. J Gastroenterol Hepatol. 33:1961–1968. DOI: 10.1111/jgh.14289. PMID: 29802647. PMCID: PMC6334276.


4. Sun D, Cao M, Li H, He S, Chen W. 2020; Cancer burden and trends in China: a review and comparison with Japan and South Korea. Chin J Cancer Res. 32:129–139. DOI: 10.21147/j.issn.1000-9604.2020.02.01. PMID: 32410791. PMCID: PMC7219092.


5. Cummings D, Wong J, Palm R, Hoffe S, Almhanna K, Vignesh S. 2021; Epidemiology, diagnosis, staging and multimodal therapy of esophageal and gastric tumors. Cancers (Basel). 13:582. DOI: 10.3390/cancers13030582. PMID: 33540736. PMCID: PMC7867245. PMID: f2dd64f9294344139bdb6b4ab170b9c1.


6. Domper Arnal MJ, Ferrández Arenas Á, Lanas Arbeloa Á. 2015; Esophageal cancer: risk factors, screening and endoscopic treatment in Western and Eastern countries. World J Gastroenterol. 21:7933–7943. DOI: 10.3748/wjg.v21.i26.7933. PMID: 26185366. PMCID: PMC4499337.


7. Luan S, Zeng X, Zhang C, Qiu J, Yang Y, Mao C, Xiao X, Zhou J, Zhang Y, Yuan Y. 2021; Advances in drug resistance of esophageal cancer: from the perspective of tumor microenvironment. Front Cell Dev Biol. 9:664816. DOI: 10.3389/fcell.2021.664816. PMID: 33816512. PMCID: PMC8017339. PMID: 35de3603f9c64442b10a87332ef70935.


8. Lindner K, Eichelmann AK, Matuszcak C, Hussey DJ, Haier J, Hummel R. 2018; Complex epigenetic regulation of chemotherapy resistance and biohlogy in esophageal squamous cell carcinoma via microRNAs. Int J Mol Sci. 19:499. Erratum in: Int J Mol Sci. 2019;20:921. DOI: 10.3390/ijms20040921. PMID: 30791591. PMCID: PMC6412518. PMID: dacafc1a8d7341df99f259a2fdc05199.


9. Krasna MJ. 2010; Multimodality therapy for esophageal cancer. Oncology (Williston Park). 24:1134–1138. PMID: 21141694.
10. Islami F, Ren JS, Taylor PR, Kamangar F. 2009; Pickled vegetables and the risk of oesophageal cancer: a meta-analysis. Br J Cancer. 101:1641–1647. DOI: 10.1038/sj.bjc.6605372. PMID: 19862003. PMCID: PMC2778505.


11. Higdon JV, Delage B, Williams DE, Dashwood RH. 2007; Cruciferous vegetables and human cancer risk: epidemiologic evidence and mechanistic basis. Pharmacol Res. 55:224–236. DOI: 10.1016/j.phrs.2007.01.009. PMID: 17317210. PMCID: PMC2737735.


12. Khwaza V, Oyedeji OO, Aderibigbe BA. 2020; Ursolic acid-based derivatives as potential anti-cancer agents: an update. Int J Mol Sci. 21:5920. DOI: 10.3390/ijms21165920. PMID: 32824664. PMCID: PMC7460570. PMID: 60ea6e8af8944627b70f910451c0984a.


13. Alam M, Ali S, Ahmed S, Elasbali AM, Adnan M, Islam A, Hassan MI, Yadav DK. 2021; Therapeutic potential of ursolic acid in cancer and diabetic neuropathy diseases. Int J Mol Sci. 22:12162. DOI: 10.3390/ijms222212162. PMID: 34830043. PMCID: PMC8621142. PMID: e17bd67e0fb74c92a8e8c2850d80e840.


14. Kassi E, Papoutsi Z, Pratsinis H, Aligiannis N, Manoussakis M, Moutsatsou P. 2007; Ursolic acid, a naturally occurring triterpenoid, demonstrates anticancer activity on human prostate cancer cells. J Cancer Res Clin Oncol. 133:493–500. DOI: 10.1007/s00432-007-0193-1. PMID: 17516089.


15. Navin R, Kim SM. 2016; Therapeutic interventions using ursolic acid for cancer treatment. Med Chem (Los Angeles). 6:339–344. DOI: 10.4172/2161-0444.1000367.


16. Figueroa-Suárez MZ, González Christen J, Cardoso-Taketa AT, Gutiérrez Villafuerte MDC, Rodríguez-López V. 2019; Anti-inflammatory and antihistaminic activity of triterpenoids isolated from Bursera cuneata (Schldl.) Engl. J Ethnopharmacol. 238:111786. DOI: 10.1016/j.jep.2019.03.013. PMID: 30872171.


17. Habtemariam S. 2019; Antioxidant and anti-inflammatory mechanisms of neuroprotection by ursolic acid: addressing brain injury, cerebral ischemia, cognition deficit, anxiety, and depression. Oxid Med Cell Longev. 2019:8512048. DOI: 10.1155/2019/8512048. PMID: 31223427. PMCID: PMC6541953.


18. do Nascimento PG, Lemos TL, Bizerra AM, Arriaga ÂM, Ferreira DA, Santiago GM, Braz-Filho R, Costa JG. 2014; Antibacterial and antioxidant activities of ursolic acid and derivatives. Molecules. 19:1317–1327. DOI: 10.3390/molecules19011317. PMID: 24451251. PMCID: PMC6271190. PMID: 25fcb9a47785495fb68e2ddcc5134986.


19. Hassan HM, Jiang ZH, Asmussen C, McDonald E, Qin W. 2014; Antibacterial activity of northern Ontario medicinal plant extracts. Can J Plant Sci. 94:417–424. DOI: 10.4141/cjps2013-258.


20. Lee NR, Meng RY, Rah SY, Jin H, Ray N, Kim SH, Park BH, Kim SM. 2020; Reactive oxygen species-mediated autophagy by ursolic acid inhibits growth and metastasis of esophageal cancer cells. Int J Mol Sci. 21:9409. DOI: 10.3390/ijms21249409. PMID: 33321911. PMCID: PMC7764507. PMID: 32da4af9afe64e46a080552397a0fd6a.


21. Yang K, Chen Y, Zhou J, Ma L, Shan Y, Cheng X, Wang Y, Zhang Z, Ji X, Chen L, Dai H, Zhu B, Li C, Tao Z, Hu X, Yin W. 2019; Ursolic acid promotes apoptosis and mediates transcriptional suppression of CT45A2 gene expression in non-small-cell lung carcinoma harbouring EGFR T790M mutations. Br J Pharmacol. 176:4609–4624. DOI: 10.1111/bph.14793. PMID: 31322286. PMCID: PMC6965687.


22. Huang CY, Lin CY, Tsai CW, Yin MC. 2011; Inhibition of cell proliferation, invasion and migration by ursolic acid in human lung cancer cell lines. Toxicol In Vitro. 25:1274–1280. DOI: 10.1016/j.tiv.2011.04.014. PMID: 21539908.


23. Hsu YL, Kuo PL, Lin CC. 2004; Proliferative inhibition, cell-cycle dysregulation, and induction of apoptosis by ursolic acid in human non-small cell lung cancer A549 cells. Life Sci. 75:2303–2316. DOI: 10.1016/j.lfs.2004.04.027. PMID: 15350828.


24. Kim SH, Jin H, Meng RY, Kim DY, Liu YC, Chai OH, Park BH, Kim SM. 2019; Activating Hippo pathway via Rassf1 by ursolic acid suppresses the tumorigenesis of gastric cancer. Int J Mol Sci. 20:4709. DOI: 10.3390/ijms20194709. PMID: 31547587. PMCID: PMC6801984. PMID: 098852b82a354918a8075acbca83ab19.


25. Kim ES, Moon A. 2015; Ursolic acid inhibits the invasive phenotype of SNU-484 human gastric cancer cells. Oncol Lett. 9:897–902. DOI: 10.3892/ol.2014.2735. PMID: 25621065.


26. Liu T, Ma H, Shi W, Duan J, Wang Y, Zhang C, Li C, Lin J, Li S, Lv J, Lin L. 2017; Inhibition of STAT3 signaling pathway by ursolic acid suppresses growth of hepatocellular carcinoma. Int J Oncol. 51:555–562. DOI: 10.3892/ijo.2017.4035. PMID: 28714512.


27. Zhou W, Lin L, Cheng Y, Liu Y. 2017; Ursolic acid improves liver transplantation and inhibits apoptosis in miniature pigs using donation after cardiac death. Cell Physiol Biochem. 43:331–338. DOI: 10.1159/000480413. PMID: 28854432. PMID: 3807e3e23c2b462a9ee8951cbd5259df.


28. Shanmugam MK, Rajendran P, Li F, Nema T, Vali S, Abbasi T, Kapoor S, Sharma A, Kumar AP, Ho PC, Hui KM, Sethi G. 2011; Ursolic acid inhibits multiple cell survival pathways leading to suppression of growth of prostate cancer xenograft in nude mice. J Mol Med (Berl). 89:713–727. DOI: 10.1007/s00109-011-0746-2. PMID: 21465181.


29. Ye Y, Fang Y, Xu W, Wang Q, Zhou J, Lu R. 2016; 3,3'-Diindolylmethane induces anti-human gastric cancer cells by the miR-30e-ATG5 modulating autophagy. Biochem Pharmacol. 115:77–84. DOI: 10.1016/j.bcp.2016.06.018. PMID: 27372603.


30. Li XJ, Park ES, Park MH, Kim SM. 2013; 3,3'-Diindolylmethane suppresses the growth of gastric cancer cells via activation of the Hippo signaling pathway. Oncol Rep. 30:2419–2426. DOI: 10.3892/or.2013.2717. PMID: 24008339.


31. Jin H, Park MH, Kim SM. 2015; 3,3'-Diindolylmethane potentiates paclitaxel-induced antitumor effects on gastric cancer cells through the Akt/FOXM1 signaling cascade. Oncol Rep. 33:2031–2036. DOI: 10.3892/or.2015.3758. PMID: 25633416.


32. Zhu P, Yu H, Zhou K, Bai Y, Qi R, Zhang S. 2020; 3,3'-Diindolylmethane modulates aryl hydrocarbon receptor of esophageal squamous cell carcinoma to reverse epithelial-mesenchymal transition through repressing RhoA/ROCK1-mediated COX2/PGE2 pathway. J Exp Clin Cancer Res. 39:113. DOI: 10.1186/s13046-020-01618-7. PMID: 32546278. PMCID: PMC7298755. PMID: 55aa1451e2f54bc69fa9687a08cc3b69.
33. Kim SJ, Lee JS, Kim SM. 2012; 3,3'-Diindolylmethane suppresses growth of human esophageal squamous cancer cells by G1 cell cycle arrest. Oncol Rep. 27:1669–1673. DOI: 10.3892/or.2012.1662. PMID: 22293900.


34. Li XJ, Leem SH, Park MH, Kim SM. 2013; Regulation of YAP through an Akt-dependent process by 3, 3'-diindolylmethane in human colon cancer cells. Int J Oncol. 43:1992–1998. DOI: 10.3892/ijo.2013.2121. PMID: 24100865.


35. Kong D, Banerjee S, Huang W, Li Y, Wang Z, Kim HR, Sarkar FH. 2008; Mammalian target of rapamycin repression by 3,3'-diindolylmethane inhibits invasion and angiogenesis in platelet-derived growth factor-D-overexpressing PC3 cells. Cancer Res. 68:1927–1934. DOI: 10.1158/0008-5472.CAN-07-3241. PMID: 18339874. PMCID: PMC3757473.


36. Rahman KW, Sarkar FH. 2005; Inhibition of nuclear translocation of nuclear factor-{kappa}B contributes to 3,3'-diindolylmethane-induced apoptosis in breast cancer cells. Cancer Res. 65:364–371. DOI: 10.1158/0008-5472.364.65.1. PMID: 15665315.


37. Han Y. 2019; Analysis of the role of the Hippo pathway in cancer. J Transl Med. 17:116. DOI: 10.1186/s12967-019-1869-4. PMID: 30961610. PMCID: PMC6454697. PMID: 8a32b04a45634498900b8618a3e93fb5.


38. He Y, Sun MM, Zhang GG, Yang J, Chen KS, Xu WW, Li B. 2021; Targeting PI3K/Akt signal transduction for cancer therapy. Signal Transduct Target Ther. 6:425. DOI: 10.1038/s41392-021-00828-5. PMID: 34916492. PMCID: PMC8677728. PMID: 37774d4ae5334c1a9a597cb056b37cdb.


39. Luo Q, Du R, Liu W, Huang G, Dong Z, Li X. 2022; PI3K/Akt/mTOR signaling pathway: role in esophageal squamous cell carcinoma, regulatory mechanisms and opportunities for targeted therapy. Front Oncol. 12:852383. DOI: 10.3389/fonc.2022.852383. PMID: 35392233. PMCID: PMC8980269. PMID: 3457337e85a54ddeb424fb2bdb7d8419.


40. Javadinia SA, Shahidsales S, Fanipakdel A, Mostafapour A, Joudi-Mashhad M, Ferns GA, Avan A. 2018; The esophageal cancer and the PI3K/AKT/mTOR signaling regulatory microRNAs: a novel marker for prognosis, and a possible target for immunotherapy. Curr Pharm Des. 24:4646–4651. DOI: 10.2174/1381612825666190110143258. PMID: 30636576.


41. Zhu C, Li L, Zhao B. 2015; The regulation and function of YAP transcription co-activator. Acta Biochim Biophys Sin (Shanghai). 47:16–28. DOI: 10.1093/abbs/gmu110. PMID: 25487920.


42. Misra JR, Irvine KD. 2018; The Hippo signaling network and its biological functions. Annu Rev Genet. 52:65–87. DOI: 10.1146/annurev-genet-120417-031621. PMID: 30183404. PMCID: PMC6322405.


43. Yu FX, Zhao B, Guan KL. 2015; Hippo pathway in organ size control, tissue homeostasis, and cancer. Cell. 163:811–828. DOI: 10.1016/j.cell.2015.10.044. PMID: 26544935. PMCID: PMC4638384.


44. Tumaneng K, Schlegelmilch K, Russell RC, Yimlamai D, Basnet H, Mahadevan N, Fitamant J, Bardeesy N, Camargo FD, Guan KL. 2012; YAP mediates crosstalk between the Hippo and PI(3)K-TOR pathways by suppressing PTEN via miR-29. Nat Cell Biol. 14:1322–1329. DOI: 10.1038/ncb2615. PMID: 23143395. PMCID: PMC4019071.


45. Qian X, He L, Hao M, Li Y, Li X, Liu Y, Jiang H, Xu L, Li C, Wu W, Du L, Yin X, Lu Q. 2021; YAP mediates the interaction between the Hippo and PI3K/Akt pathways in mesangial cell proliferation in diabetic nephropathy. Acta Diabetol. 58:47–62. DOI: 10.1007/s00592-020-01582-w. PMID: 32816106.


46. Ye X, Deng Y, Lai ZC. 2012; Akt is negatively regulated by Hippo signaling for growth inhibition in Drosophila. Dev Biol. 369:115–123. DOI: 10.1016/j.ydbio.2012.06.014. PMID: 22732571.


47. Borreguero-Muñoz N, Fletcher GC, Aguilar-Aragon M, Elbediwy A, Vincent-Mistiaen ZI, Thompson BJ. 2019; The Hippo pathway integrates PI3K-Akt signals with mechanical and polarity cues to control tissue growth. PLoS Biol. 17:e3000509. DOI: 10.1371/journal.pbio.3000509. PMID: 31613895. PMCID: PMC6814241. PMID: 49700236fbce48088ee3945b33c7f241.


48. Hu LL, Su T, Luo RC, Zheng YH, Huang J, Zhong ZS, Nie J, Zheng LP. 2019; Hippo pathway functions as a downstream effector of AKT signaling to regulate the activation of primordial follicles in mice. J Cell Physiol. 234:1578–1587. DOI: 10.1002/jcp.27024. PMID: 30078193.


49. Lin Z, Zhou P, von Gise A, Gu F, Ma Q, Chen J, Guo H, van Gorp PR, Wang DZ, Pu WT. 2015; Pi3kcb links Hippo-YAP and PI3K-AKT signaling pathways to promote cardiomyocyte proliferation and survival. Circ Res. 116:35–45. DOI: 10.1161/CIRCRESAHA.115.304457. PMID: 25249570. PMCID: PMC4282610.


50. Xu W, Yang Z, Xie C, Zhu Y, Shu X, Zhang Z, Li N, Chai N, Zhang S, Wu K, Nie Y, Lu N. 2018; PTEN lipid phosphatase inactivation links the hippo and PI3K/Akt pathways to induce gastric tumorigenesis. J Exp Clin Cancer Res. 37:198. DOI: 10.1186/s13046-018-0795-2. PMID: 30134988. PMCID: PMC6104022. PMID: fa829ec5bd6b4945a08ad9b0211c1d69.


51. Worby CA, Dixon JE. 2014; PTEN. Annu Rev Biochem. 83:641–669. DOI: 10.1146/annurev-biochem-082411-113907. PMID: 24905788.


52. Zou R, Xu Y, Feng Y, Shen M, Yuan F, Yuan Y. 2020; YAP nuclear-cytoplasmic translocation is regulated by mechanical signaling, protein modification, and metabolism. Cell Biol Int. 44:1416–1425. DOI: 10.1002/cbin.11345. PMID: 32190949.


53. Kitagawa Y, Uno T, Oyama T, Kato K, Kato H, Kawakubo H, Kawamura O, Kusano M, Kuwano H, Takeuchi H, Toh Y, Doki Y, Naomoto Y, Nemoto K, Booka E, Matsubara H, Miyazaki T, Muto M, Yanagisawa A, Yoshida M. 2019; Esophageal cancer practice guidelines 2017 edited by the Japan Esophageal Society: part 1. Esophagus. 16:1–24. Erratum in: Esophagus. 2022;19:726. DOI: 10.1007/s10388-018-0641-9. PMID: 30171413. PMCID: PMC6510883.


54. Meng RY, Jin H, Nguyen TV, Chai OH, Park BH, Kim SM. 2021; Ursolic acid accelerates paclitaxel-induced cell death in esophageal cancer cells by suppressing Akt/FOXM1 signaling cascade. Int J Mol Sci. 22:11486. DOI: 10.3390/ijms222111486. PMID: 34768915. PMCID: PMC8584129. PMID: d28bd70cecc64f66bf6cb2ef10fc4235.


55. Biersack B. 2020; 3,3'-Diindolylmethane and its derivatives: nature-inspired strategies tackling drug resistant tumors by regulation of signal transduction, transcription factors and microRNAs. Cancer Drug Resist. 3:867–878. DOI: 10.20517/cdr.2020.53. PMID: 35582221. PMCID: PMC8992569.


56. Lin W, Ye H. 2020; Anticancer activity of ursolic acid on human ovarian cancer cells via ROS and MMP mediated apoptosis, cell cycle arrest and downregulation of PI3K/AKT pathway. J BUON. 25:750–756. PMID: 32521863.
57. Meng Y, Lin ZM, Ge N, Zhang DL, Huang J, Kong F. 2015; Ursolic acid induces apoptosis of prostate cancer cells via the PI3K/Akt/mTOR pathway. Am J Chin Med. 43:1471–1486. DOI: 10.1142/S0192415X15500834. PMID: 26503559.


58. Wong RS. 2011; Apoptosis in cancer: from pathogenesis to treatment. J Exp Clin Cancer Res. 30:87. DOI: 10.1186/1756-9966-30-87. PMID: 21943236. PMCID: PMC3197541. PMID: 14037097dac24b9dae7629da9c125275.


59. Tahtamouni L, Ahram M, Koblinski J, Rolfo C. 2019; Molecular regulation of cancer cell migration, invasion, and metastasis. Anal Cell Pathol (Amst). 2019:1356508. DOI: 10.1155/2019/1356508. PMID: 31218208. PMCID: PMC6536998. PMID: a0407114b4874fdb92140eab9fe3e998.


60. Shin JY, Kim JO, Lee SK, Chae HS, Kang JH. 2010; LY294002 may overcome 5-FU resistance via down-regulation of activated p-AKT in Epstein-Barr virus-positive gastric cancer cells. BMC Cancer. 10:425. DOI: 10.1186/1471-2407-10-425. PMID: 20704765. PMCID: PMC3087326. PMID: e37d2abf32d94d3ca2610f5c88c54213.


61. Datta S, Cano M, Satyanarayana G, Liu T, Wang L, Wang J, Cheng J, Itoh K, Sharma A, Bhutto I, Kannan R, Qian J, Sinha D, Handa JT. 2023; Mitophagy initiates retrograde mitochondrial-nuclear signaling to guide retinal pigment cell heterogeneity. Autophagy. 19:966–983. DOI: 10.1080/15548627.2022.2109286. PMID: 35921555. PMCID: PMC9980637.


62. Domoto T, Pyko IV, Furuta T, Miyashita K, Uehara M, Shimasaki T, Nakada M, Minamoto T. 2016; Glycogen synthase kinase-3β is a pivotal mediator of cancer invasion and resistance to therapy. Cancer Sci. 107:1363–1372. DOI: 10.1111/cas.13028. PMID: 27486911. PMCID: PMC5084660.


63. Duda P, Akula SM, Abrams SL, Steelman LS, Martelli AM, Cocco L, Ratti S, Candido S, Libra M, Montalto G, Cervello M, Gizak A, Rakus D, McCubrey JA. 2020; Targeting GSK3 and associated signaling pathways involved in cancer. Cells. 9:1110. DOI: 10.3390/cells9051110. PMID: 32365809. PMCID: PMC7290852. PMID: 4013e82b8ba443de968a8ae058a04c3d.


64. Fu Y, Sun S, Sun H, Peng J, Ma X, Bao L, Ji R, Luo C, Gao C, Zhang X, Jin Y. 2019; Scutellarin exerts protective effects against atherosclerosis in rats by regulating the Hippo-FOXO3A and PI3K/AKT signaling pathways. J Cell Physiol. 234:18131–18145. DOI: 10.1002/jcp.28446. PMID: 30891776.


Fig. 1
Ursolic acid (UA) plus 3,3’-diindolylmethane (DIM) inhibits cell growth and promotes apoptosis in ESCC.
(A) UA and DIM combination therapy inhibited cell viability in TE-8 and TE-12 cells. Cells were treated with UA (20 μM), DIM (50 μM), and combination treatment for 48 h. An EZ-CYTOX assay kit was used to assess the cell viability of esophageal cancer cells and to generate growth curves. (B) UA plus DIM combination reduced colony number and size in TE-8 and TE-12 cells. Colonies were cultured with UA (20 μM), DIM (50 μM), and the combination treatment of UA and DIM for 4 weeks in soft agar gel. The quantification of colony numbers was calculated by ImageJ. (C) UA plus DIM increased early-stage and late-stage apoptosis in TE-8 and TE-12 cells. Annexin V/PI staining was performed to detect cell apoptosis by flow cytometry. (D) UA plus DIM combination induced subG1 phase cell accumulation in TE-8 and TE-12 cells. Cells were treated with UA (20 μM), DIM (50 μM), and combination treatment for 48 h. SubG1 phase cells were indicated by apoptosis markers and detected by flow cytometry. (E) UA plus DIM enhanced apoptosis-related protein levels. Expression of apoptosis-regulatory factor proteins caspase-9/cleaved-caspase-9 and PRAP/cleaved-PARP were examined by Western blot analysis. The quantification of protein bands was estimated by ImageJ software. GAPDH was used as the internal control. Data are expressed as the mean ± standard error of the mean. CONT, control; DMSO, dimethyl sulfoxide; ESCC, esophageal squamous cell carcinoma; PI, propidium iodide; n.s., no significant. *, compared to the control; #, compare with UA plus DIM combination treatment. * or #, p < 0.05; ** or ##, p < 0.01.

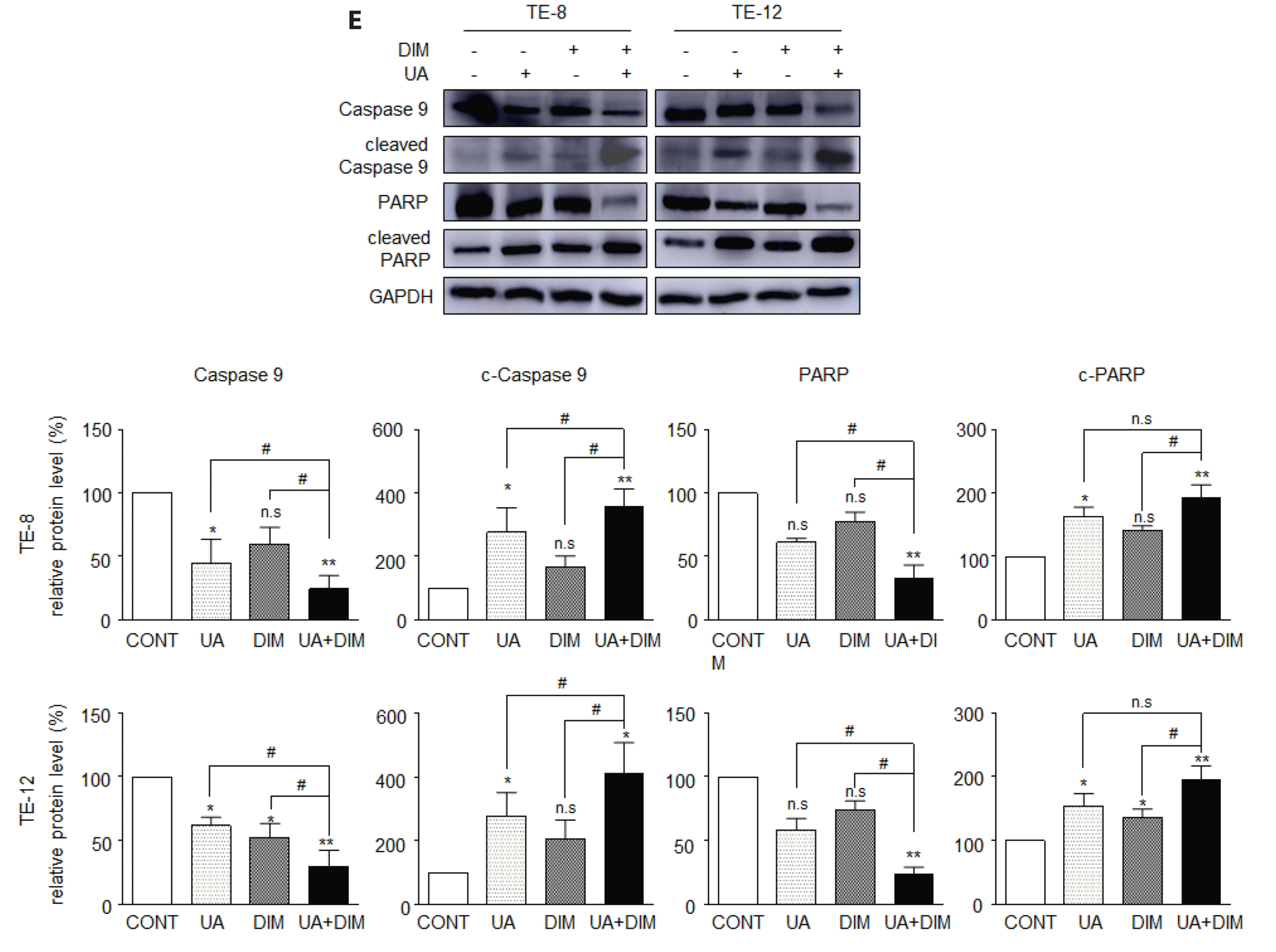
Fig. 2
Ursolic acid (UA) plus 3,3’-diindolylmethane (DIM) induces G1 phase arrest in ESCC.
(A) UA plus DIM induced G1 phase arrest in TE-8 and TE-12 cells. Cells were treated with UA (20 μM), DIM (50 μM), and a combination of both for 48 h. PI staining-based flow cytometry assay was designed to study the cell cycle in esophageal cancer cells. (B) UA plus DIM combination suppressed G1 phase-related protein levels. Western blot analysis was performed to measure G1 phase-related protein CDK4, CDK6, and cyclin D1. GAPDH was used as the internal control. Data are expressed as the mean ± standard error of the mean. CONT, control; ESCC, esophageal squamous cell carcinoma; PI, propidium iodide; n.s., no significant. *, compared to the control; #, compare with UA plus DIM combination treatment. * or #, p < 0.05; ** or ##, p < 0.01.
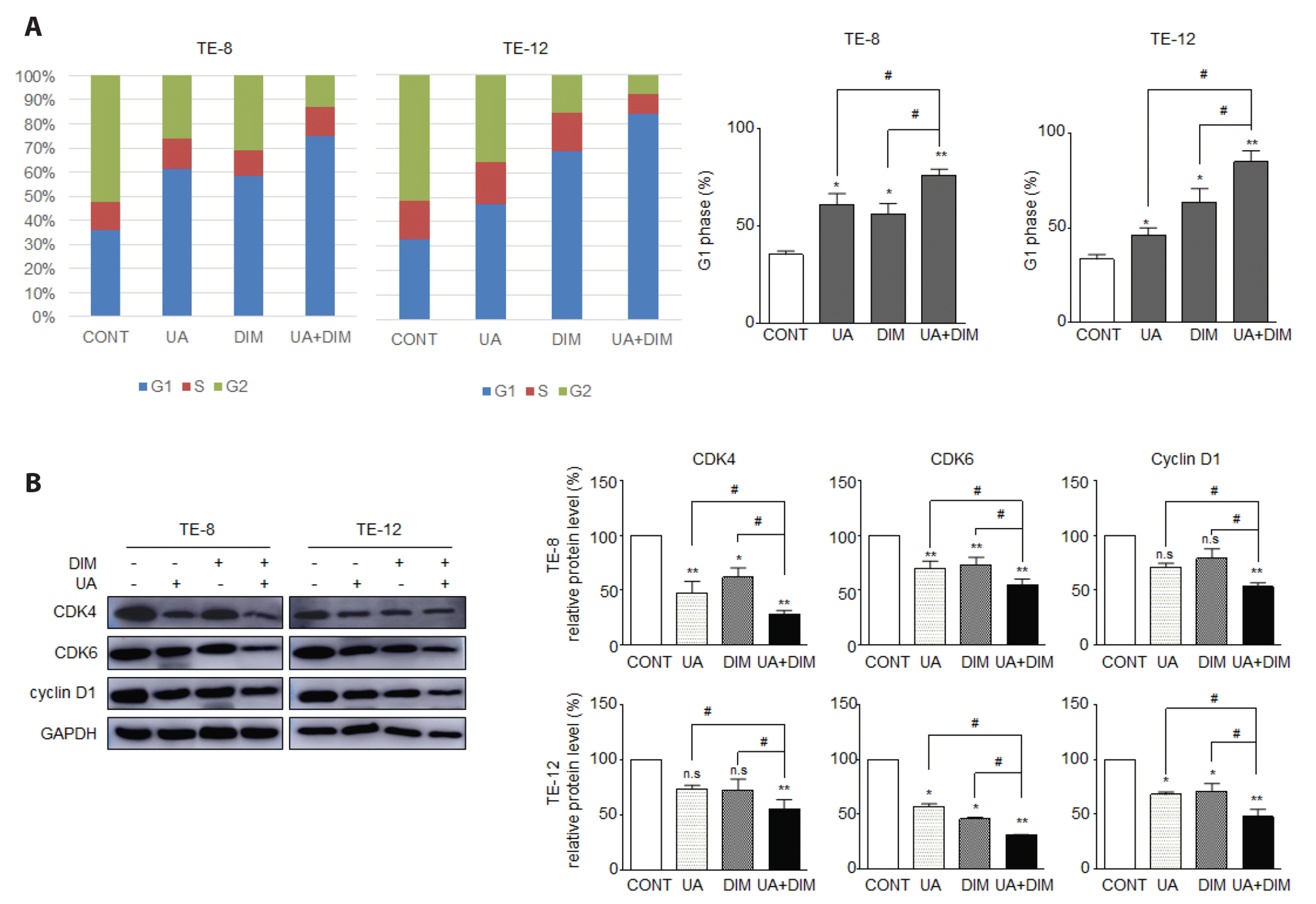
Fig. 3
Ursolic acid (UA) plus 3,3’-diindolylmethane (DIM) suppresses cell migration in ESCC.
(A) UA (20 μM) and DIM (50 μM) combination treatment inhibited cell migration in the presence of 5 µg/ml mitomycin-C. Wound healing assays were used to analyze cell migration. TE-8 and TE-12 cell migration rates were measured at 12 h and 24 h after UA or DIM single treatment or a combination and calculated by ImageJ software. (B) UA plus DIM inhibited migration-related protein expression. Migration-related proteins E-cadherin, MMP-13, and MMP-9 were examined by Western blot analysis. GAPDH was used as the internal control. Data are expressed as the mean ± standard error of the mean. CONT, control; ESCC, esophageal squamous cell carcinoma; n.s., no significant. *, compared to the control; #, compare with UA plus DIM combination treatment. * or #, p < 0.05; ** or ##, p < 0.01.

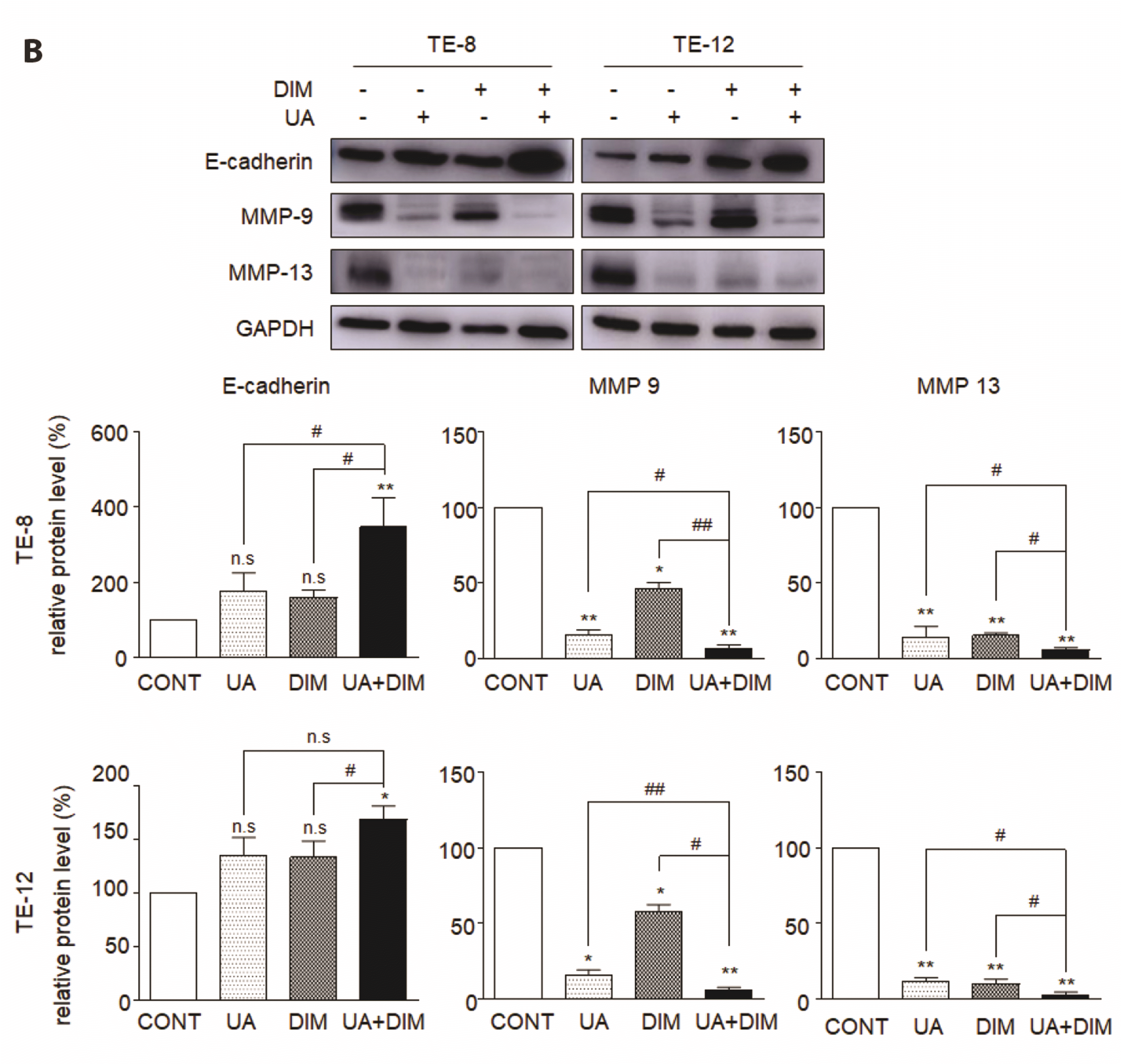
Fig. 4
Ursolic acid (UA) plus 3,3’-diindolylmethane (DIM) inactivates the PI3K/Akt/GSK-3β signaling pathway in ESCC.
After TE-8 and TE-12 cells were treated with UA (20 μM), DIM (50 μM), and a combination for 48 h. Akt/p-Akt and Gsk-3β/p-GSK 3β protein levels were examined by Western blot analysis. The quantification of protein bands was estimated by ImageJ software. GAPDH was used as the internal control. Data are expressed as the mean ± standard error of the mean. CONT, control; ESCC, esophageal squamous cell carcinoma; n.s., no significant. *, compared to the control; #, compare with UA plus DIM combination treatment. * or #, p < 0.05; ** or ##, p < 0.01.
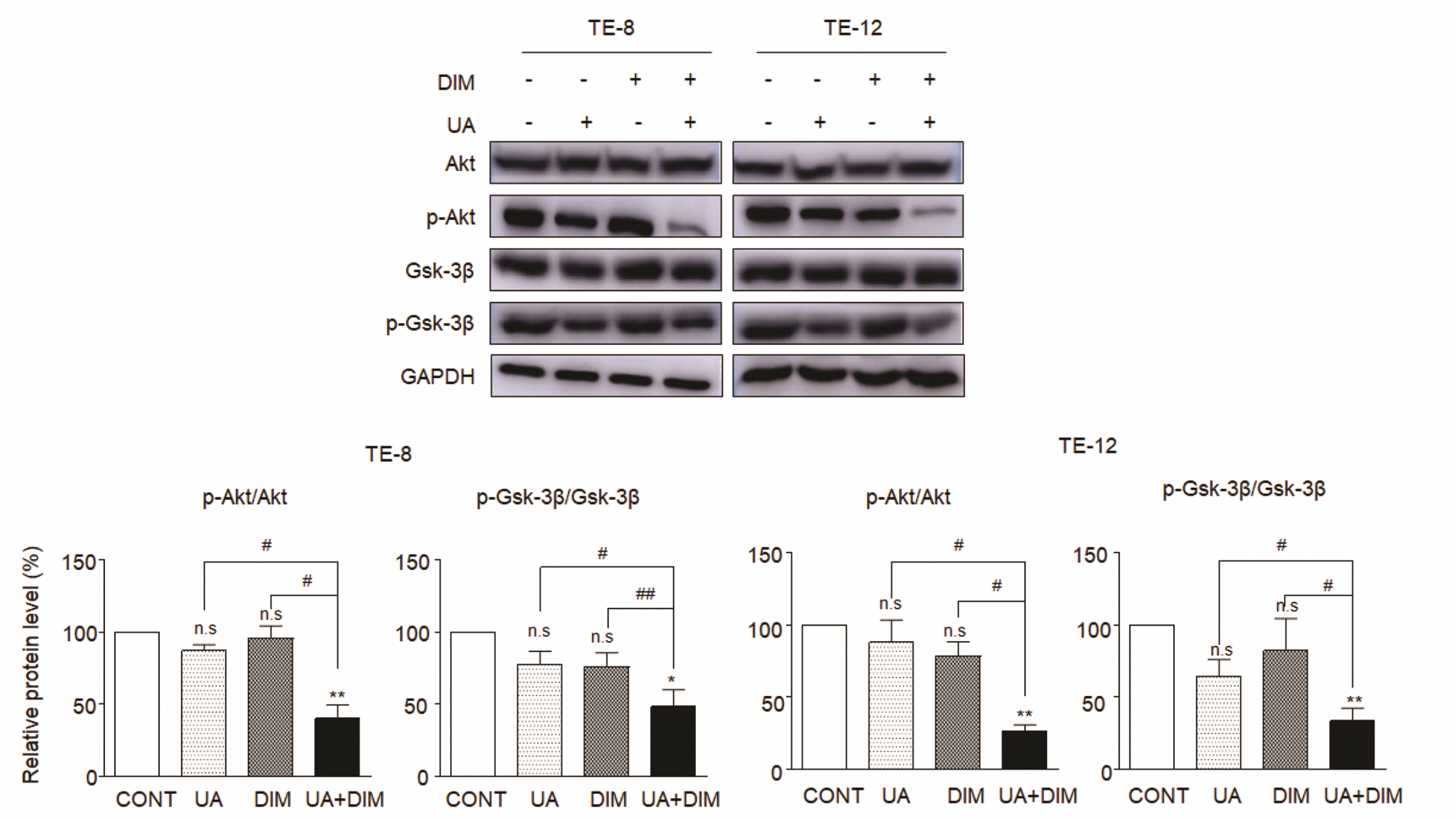
Fig. 5
Ursolic acid (UA) plus 3,3’-diindolylmethane (DIM) actives the Hippo signaling pathway and down-regulates the target gene CTGF in ESCC.
TE-8 and TE-12 cells were treated with UA (20 μM), DIM (50 μM), or combination treatment for 48 h. Western blot analysis was used to determine the Hippo signaling pathway: (A) Rassf1, Mst1, Mst2, Sav1, Mob-1, and p-Mob1, (B) YAP and p-YAP. (C) The Hippo signaling pathway CTGF mRNA level was detected by real-time PCR and (D) protein levels were examined by Western blot analysis. GAPDH was used as the internal control. Data are expressed as the mean ± standard error of the mean. CONT, control; ESCC, esophageal squamous cell carcinoma; n.s., no significant. *, compared to the control; #, compare with UA plus DIM combination treatment. * or #, p < 0.05; ** or ##, p < 0.01.


Fig. 6
Inhibition of the PI3K/Akt pathway stimulated by ursolic acid (UA) plus 3,3’-diindolylmethane (DIM) combination treatment induces Hippo signaling pathway activation in ESCC.
TE-8 and TE-12 cells were pre-treated with LY294002 (50 μM) or 3-MA (5 mM) for 2 h and further treated with UA (20 μM) plus DIM (50 μM) for 48 h. The expression of Hippo pathway-related proteins: (A) Rassf1, Mst1, Mst2, Sav1, Mob-1, p-Mob1, (B) YAP, and p-YAP and Hippo signaling pathway downstream proteins CTGF were examined by Western blotting. (C) Cytoplasm and nuclear proteins were separated to detect YAP distribution by Western blotting. GAPDH was used as the internal control and cytoplasm marker. Lamin B was used as a nuclear marker. (D) TE-8 and TE-12 cells were stained with anti-YAP antibody (green) and DAPI (blue) and visualized under fluorescence microscopy. Nuclei were stained by DAPI. The value of immunofluorescence was measured and calculated by ImageJ software. Data are expressed as the mean ± standard error of the mean. CONT, control; LY, LY294002; Mix, UA plus DIM; ESCC, esophageal squamous cell carcinoma; n.s., no significant. *, compared to the control; a, combined therapy (mix) compared with mix plus LY294002; #, mix compared with mix plus 3-MA. * or #, p < 0.05; **, ## or aa, p < 0.01.



Fig. 7
Knockdown of YAP accelerates ursolic acid (UA) plus 3,3’-diindolylmethane (DIM) combination treatment induced down-regulation of the PI3K/Akt pathway by targeting PTEN protein expression in ESCC.
Protein levels of Akt, p-Akt, PTEN, and p-PTEN were measured by Western blot analysis after YAP knockdown by siRNA 24 h following treatment with UA (20 μM) and DIM (50 μM) for 48 h. GAPDH was used as the internal control. Data are expressed as the mean ± standard error of the mean. CONT, control; KD, YAP siRNA knockdown; Mix, UA plus DIM; ESCC, esophageal squamous cell carcinoma; n.s., no significant. *, compared to the control; #, compare with mix plus YAP siRNA knockdown. * or #, p < 0.05; **p < 0.01; ***p < 0.001.

Fig. 8
Ursolic acid (UA) plus 3,3’-diindolylmethane (DIM) safely and effectively inhibits tumorigenesis through the PI3K/Akt signaling pathway and Hippo signaling pathway in a xenograft mouse model.
TE-8 cells were subcutaneously injected into nude mice to create the xenograft mouse model. After tumors were established, mice were injected with UA, DIM, or combination treatment for 3 weeks. (A) Serum was extracted from mouse blood at 4°C. ALT, AST, BUN, and creatine were used as serum biochemical indices for drug safety evaluation. (B) Body weight and (C) tumor volume was measured every 3 days. (D) Tumor weights were measured after mice were sacrificed. (E) Tumor images were obtained after they were removed from mice. (F) H&E staining (left) and Ki67 staining (right) of tumor tissue (50 uM). Akt-related proteins (G) and Hippo pathway-related proteins (H) are analyzed by Western blotting. Data are expressed as the mean ± standard error of the mean. GAPDH was used as the internal control. CONT, control; ALT, alanine aminotransferase; AST, aspartate aminotransferase; BUN, blood urea nitrogen; n.s., no significant. *, compared to the control; #, compare with UA plus DIM combination treatment. * or #, p < 0.05; ** or ##, p < 0.01.

Fig. 9
Schematic diagram.
Ursolic acid (UA) plus 3,3’-diindolylmethane (DIM) combination treatment can induce apoptosis by promoting cleaved-PARP and caspase-9, increasing the G1 phase by upregulating CDK4, CDK6, and Cyclin D1 protein expression, and inhibiting migration by regulating E-cadherin, MMP-9, and MMP-13 in esophageal squamous cell carcinoma (ESCC). UA plus DIM combination treatment lowered p-Akt and p-Gsk-3β expression in ESCC. UA plus DIM combination activated the Hippo signaling pathway leading to the inhibition of YAP de-phosphorylation and nuclear translocation and suppression of its downstream gene CTGF further restrained cell growth in ESCC. UA and DIM combination treatment induced the Hippo pathway activation by inhibiting the PI3K/Akt pathway in ESCC. UA plus DIM combination treatment can induce YAP inhibition by conversely inhibiting the PI3K/Akt pathway through up-regulated PTEN. UA plus DIM stimulated a feedback loop between the PI3K/Akt signaling pathway and Hippo signaling pathways in ESCC.
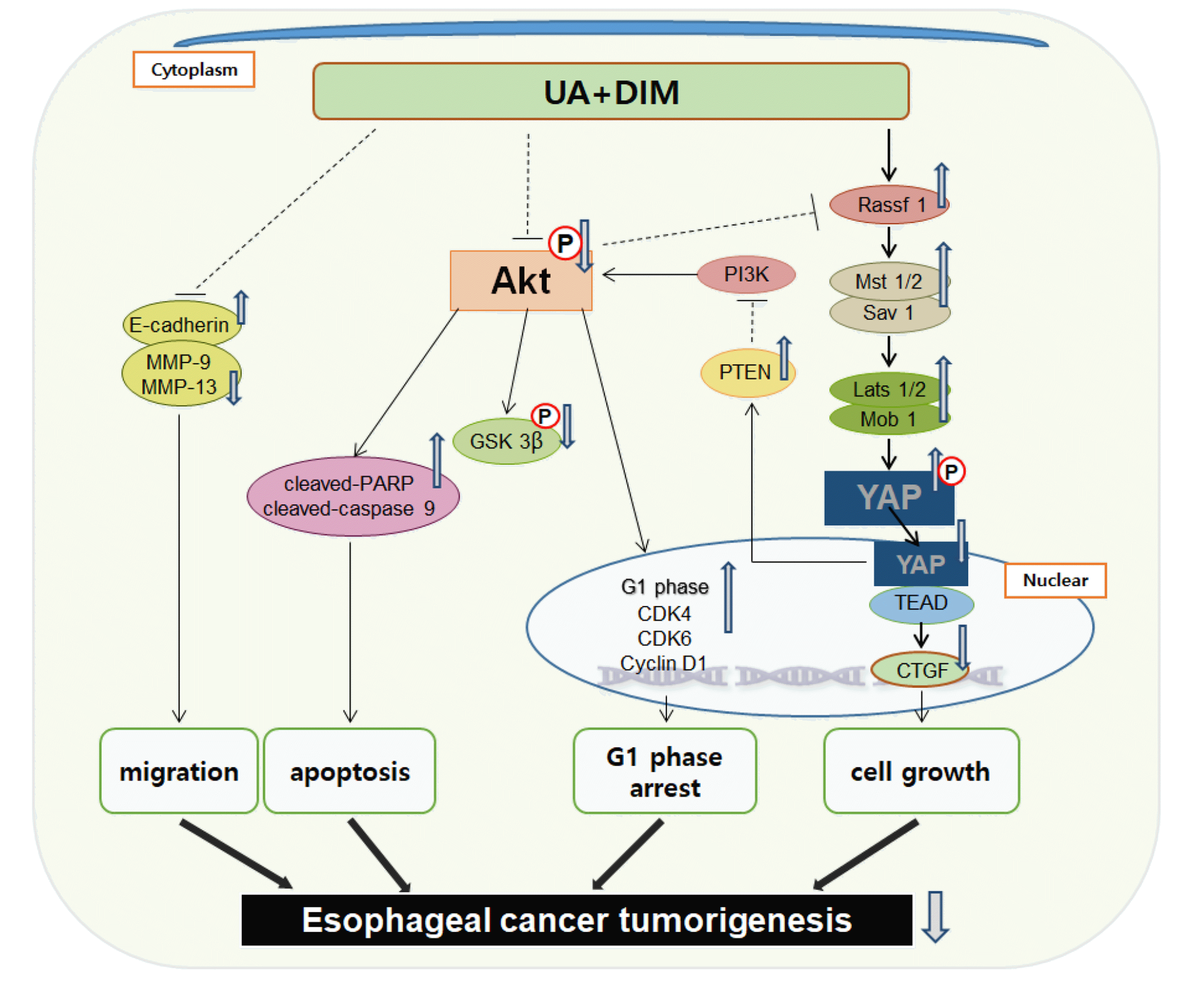