Abstract
Diabetic kidney disease is one of the most serious complications of diabetes. Although diabetic kidney disease can be effectively controlled through strict blood glucose management and corresponding symptomatic treatment, these therapies cannot reduce its incidence in diabetic patients. The sodium-glucose cotransporter 2 (SGLT2) inhibitors and the traditional Chinese herb “Gegen” have been widely used in diabetes-related therapy. However, it remains unclear whether the combined use of these two kinds of medicines contributes to an increased curative effect on diabetic kidney disease. In this study, we examined this issue by evaluating the efficacy of the combination of puerarin, an active ingredient of Gegen, and canagliflozin, an SGLT2 inhibitor for a 12-week intervention using a mouse model of diabetes. The results indicated that the combination of puerarin and canagliflozin was superior to canagliflozin alone in improving the metabolic and renal function parameters of diabetic mice. Our findings suggested that the renoprotective effect of combined puerarin and canagliflozin in diabetic mice was achieved by reducing renal lipid accumulation. This study provides a new strategy for the clinical prevention and treatment of diabetic kidney disease. The puerarin and SGLT2 inhibitor combination therapy at the initial stage of diabetes may effectively delay the occurrence of diabetic kidney injury, and significantly alleviate the burden of renal lipotoxicity.
The prevalence of diabetes has dramatically risen worldwide over the past few decades as people’s lifestyles have changed. This chronic metabolic disease, characterized by elevated blood glucose levels, can cause severe damage to various organs in the body over time. Diabetic kidney disease is one of the most common fatal complications of diabetes and the most common cause of chronic and end-stage renal disease [1]. Although the treatment methods for diabetic kidney disease have improved in recent years and disease progression can be well controlled through strict blood glucose management, the incidence of diabetic kidney disease did not reduce significantly [2,3].
Sodium-glucose cotransporter 2 (SGLT2) inhibitors are a relatively new class of diabetes drugs, which reduce blood glucose concentrations by inhibiting renal glucose reabsorption and increasing glucose excretion in urine. In addition to the traditional hypoglycemic effect, researchers have also observed renoprotective effects of SGLT2 inhibitors in patients with diabetes or other chronic metabolic diseases [4-7], such as improved renal outcomes, e.g., serum creatinine, development of macroalbuminuria, need for dialysis and/or transplantation, and kidney death. In 2019, the Food and Drug Administration of America approved the SGLT2 inhibitor canagliflozin for the treatment of diabetic kidney disease. Renoprotective effects of SGLT2 inhibition include improved renal cortical oxygenation, renal mitochondrial function, tubular autophagy, and other blood glucose-dependent and independent pathways [8]. Undoubtedly, the mystery of SGLT2 inhibitors for renoprotection still need to be further elucidated. Moreover, the pathophysiological mechanisms of diabetic kidney disease are complex, and the existing treatments do not completely prevent kidney injury in diabetic patients. Therefore, the development of a prevention and treatment intervention that can be applied at the initial diabetes diagnosis is urgently needed.
Diabetic renal injury is a complex result of glucotoxicity and lipotoxicity. Growing evidence indicates that renal lipid metabolism disorder, lipid accumulation and oxidative stress, inflammation, and fibrosis are the main factors underlying the pathogenesis of diabetic kidney disease. However, therapeutic methods targeting these pathways are still lacking [9]. Pueraria lobata (Willd.) Ohwi, a common “drug homologous” plant called Gegen in Chinese herbal medicine, is widely distributed in Asia, North America, and South America. In traditional Chinese medicine, Gegen is often combined with other herbs to treat cardiovascular diseases, diabetes, and other chronic metabolic diseases. The isoflavone puerarin is the main active ingredient of Gegen [10-12]. In this study, the combination of canagliflozin and puerarin intervention for diabetic mice surpassed effectiveness of any single drug treatment in alleviating renal lipotoxicity and maintaining stable blood glucose levels, thereby effectively delaying the occurrence of renal injury and reducing the degree of damage. These findings suggest the combination of canagliflozin and puerarin as potential therapy for the prevention and treatment of diabetic kidney disease.
Sixty-six 7-week-old male C57BL/6 mice were purchased from Chengdu Dashuo Experimental Animal Breeding Co., Ltd. After a week of adaptation, the mice were randomly divided into eleven groups and treated continuously for 12 weeks, as follows: (i) control group (normal mice with no treatment), (ii) diabetes group (diabetes mice treated with intragastric administration of saline), (iii) canagliflozin group (diabetes mice with intragastric administration of 1.5 mg/kg canagliflozin), (iv) puerarin groups (diabetes mice with intragastric administration of 4, or 10, or 40, or 100 mg/kg puerarin), (v) puerarin + canagliflozin groups (diabetes mice with intragastric administration of 1.5 mg/kg canagliflozin and 4, or 10, or 40, or 100 mg/kg puerarin). In order to explore the dose-response relationship between the combination of puerarin and canagliflozin, we designed four doses of puerarin for the study. Puerarin, chemical name is 4H-1-Benzopyran-4-one, 8-β-D-glucopyranosyl-7-hydroxy-3-(4-hydroxyphenyl)-, was purchased from Shanghai Yuanye Bio-Technology Co., Ltd., and canagliflozin was purchased from Janssen-Cilag International NV.
Diabetes modeling methods were conducted as follows. The mice were fed a high-fat diet (63.4% regular diet plus 20% lard, 15% sucrose, 1.5% cholesterol, 0.1% sodium cholate), and intraperitoneally administered the freshly prepared streptozotocin (S8050; Solarbio, dissolved in citrate buffer, pH 4.5) at 50 mg/kg after 4–6 h of food withdrawal for 5 days. Blood glucose was measured weekly using a blood glucometer (ACCU-CHEK Active; Roche). Two weeks after diabetes was confirmed (blood glucose > 15.8 mM), mice were treated with puerarin and/or canagliflozin for 12 weeks.
All animal procedures were approved (approval no. 2021DL-031) by the Ethics Committee of the Hospital of Chengdu University of Traditional Chinese Medicine Animal Policy and Welfare Committee. Mice were housed in an environmentally controlled room at 22°C ± 2.0°C, 50% ± 5% humidity, in a 12-h:12-h light/dark cycle, with food and water provided ad libitum.
Body weight and blood glucose (ACCU-CHEK Active blood glucometer) from the tail vein were measured every week after an overnight fast. Water and food intake were measured every 4 weeks. At the end of the 12-week intervention, total cholesterol (TC) (A111-1-1, Nanjing JianCheng Bioengineering Institute), low-density lipoprotein cholesterol (LDL-C) (A113-1-1; Nanjing JianCheng Bioengineering Institute), high-density lipoprotein cholesterol (HDL-C) (A112-1-1; Nanjing JianCheng Bioengineering Institute), and triglyceride (TG) (A110-1-1; Nanjing JianCheng Bioengineering Institute) levels were determined by biochemical methods.
A metabolic cage was used to collect urine. Urinary volume, urinary glucose excretion (BC2505; Solarbio), urinary albumin (MM-44287M1; MEIMIAN), and urinary creatinine (C011-2-1; Nanjing JianCheng Bioengineering Institute) were measured every 2 weeks. At the end of the 12-week intervention, blood urea nitrogen (BUN) (BC1535; Solarbio) and creatinine (C011-2-1; Nanjing JianCheng Bioengineering Institute) were determined by biochemical methods, and the kidneys were weighed.
Paraffin-embedded kidney samples were cut into 4-μm-thick sections for hematoxylin and eosin (HE) staining and periodic acid-Schiff (PAS) staining to evaluate the incrassation of the glomerular mesangial matrix; the positive area was calculated as previously described [13,14]. Picrosirius red staining was used to detect renal fibrosis as previously reported [15]. Frozen kidney samples were cut into 10-μm-thick sections for oil red O staining to evaluate the lipid accumulation.
The expression of CD36, adipophilin, and PPARα was detected using Western blotting. Kidney proteins were obtained via cold radioimmunoprecipitation assay lysis buffer (BL504A; Biosharp) with the addition of phenylmethylsulfonyl fluoride (BL507A; Biosharp) on ice and quantified using a bicinchoninic acid assay kit (P0012S; Beyotime). Western blotting was performed according to standard protocols. The following antibodies were used: anti-CD36 (1:1,000, bs-1100R, Bioss), anti-adipophilin (1:300, bs-1164R, Bioss), anti-PPARα (1:600, 15540-1-AP, Proteintech) and anti-GAPDH (1:1,500, BA2913, Boster).
Kidney homogenates were centrifuged to obtain supernatants (3,500 rpm, 15 min, 4°C) to measure superoxide dismutase (SOD), catalase (CAT), glutathione peroxidase (GPx), glutathione (GSH), and malondialdehyde (MDA) levels by biochemical methods (BC0175, BC0205, BC1195, BC1175, BC0025; Solarbio).
Western blotting was used to estimate tumor necrosis factor α (TNFα), interferon γ (IFNγ), interleukin (IL) 6, and IL1β expression according to standard protocols described before. The following antibodies were used: anti-TNFα (1:800, 17590-1-AP, Proteintech), anti-IFNγ (1:1,000, 15365-1-AP, Proteintech), anti-IL6 (1:600, bs-0782R, Bioss), anti-IL1β (1:800, bs-0812R, Bioss), and anti-β-actin (1:1,000, bs-0061R, Bioss).
The body weight presented no significant difference before the treatment. At the end of the treatment, however, body weight was significantly higher in the diabetes group and puerarin groups compared to the control group; the canagliflozin, higher dose of puerarin (40 mg/kg and 100 mg/kg), and canagliflozin + puerarin groups were significantly lower than those of the diabetes group; the canagliflozin + puerarin groups with higher doses of puerarin (40 mg/kg and 100 mg/kg) were significantly lower than the canagliflozin group (Fig. 1A, C, Supplementary Table 1). Additionally, the blood glucose level was significantly higher in the diabetes group and the puerarin group than in the control group, and was lower in each treatment group than in the diabetes group. Blood glucose levels were also higher in the puerarin groups when compared to the canagliflozin group, while canagliflozin + puerarin groups were similar with the canagliflozin group (Fig. 1B, D, Supplementary Table 2).
In terms of lipid metabolism indicators at the end of treatment, the levels of TC, LDL-C, and TG levels were higher, and the level of HDL-C was lower in the diabetes group when compared with the control group (control group vs. diabetes group, Table 1). All parameters in all treated groups, i.e., canagliflozin, puerarin groups, and canagliflozin + puerarin groups, recovered to varying degrees (treated groups vs. diabetes group, Table 1). Combined use canagliflozin + puerarin groups with higher doses of puerarin (40 mg/kg and 100 mg/kg) showed better performance in restoring the parameters of lipid metabolism (canagliflozin group vs. canagliflozin + puerarin groups, Table 1). In terms of water intake, food intake, urine volume, and urinary glucose excretion, the combination therapies revealed more improvement in these parameters when compared with therapies using puerarin or canagliflozin alone (Supplementary Tables 3 and 4). Based on the above results, 40 mg/kg puerarin was chosen as an applicable dose in the following part of our study.
For the urinary albumin excretion, all groups increased to varying degrees over the control group within 22 weeks of treatment; all treated groups significantly decreased compared to the diabetes group (Fig. 2A). Notably, the puerarin + canagliflozin group showed a remarkable delay in the occurrence of urinary albumin excretion, compared to all other groups except the control group (Fig. 2A). At the end of the treatment, mice in the diabetes group had the highest levels of kidney weight, relative kidney weight, BUN, and serum creatinine (control group vs. diabetes group, Table 2). Treatment with puerarin and/or canagliflozin restored these values (treated groups vs. diabetes group, Table 2). The combined use of puerarin and canagliflozin showed a greater effect on kidney weight, BUN, and serum creatinine levels than using canagliflozin alone (canagliflozin groups vs. puerarin + canagliflozin group, Table 2).
To further verify the renoprotective effect of the combination of canagliflozin and puerarin in diabetic mice, we performed HE staining of kidney sections to observe morphology. PAS staining was used to evaluate the expansion of the glomerular basement membrane, and picrosirius red staining to observe the interstitial fibrosis. In the diabetes group, HE staining demonstrated a more pronounced tubular vacuolation (Fig. 2B upper panels), and PSA staining revealed considerable glomerular basement membrane expansion. The expansion was ameliorated by the puerarin + canagliflozin treatment (Fig. 2B middle panels and C). Furthermore, picrosirius red staining demonstrated that interstitial fibrosis was more severe in the diabetes group than in the control group. All treatments were able to suppress interstitial fibrosis, with the combined use of puerarin and canagliflozin being most effective (Fig. 2B lower panels and D).
Oil red O staining revealed that lipid accumulation was aggravated in the diabetes group compared with the control group and improved in the puerarin and puerarin + canagliflozin groups compared with the diabetes group. Notably, the lipid droplets were ameliorated more effectively by puerarin + canagliflozin treatment than by canagliflozin treatment (Fig. 3A, B). To investigate the anti-lipid accumulation effect of puerarin + canagliflozin therapy, we detected the level of fatty acid synthesis and oxidation in the kidney, marked by the protein expression of CD36, adipophilin, and PPARα using Western blotting. The levels of CD36 and adipophilin were decreased in the puerarin + canagliflozin group compared with the groups treated with either drug alone, and the protein level of PPARα was increased in the puerarin and puerarin + canagliflozin groups compared with the diabetes group (Fig. 3C–F). Together, these results suggest that the combination of canagliflozin and puerarin may prevent lipid accumulation by inhibiting fatty acid synthesis and promoting fatty acid oxidation.
It is well known that lipotoxicity causes damage via oxidative stress and inflammatory responses [9]. In this study, we verified the increased levels of oxidative stress and inflammatory response in diabetic kidneys by measuring the levels of MDA, GSH, TNFα, IFNγ, IL6, and IL1β, and the activities of GPx, SOD, and CAT. No significant differences in these parameters were detected between the puerarin + canagliflozin group and the control group, suggesting the changes observed in the diabetes group were restored by the combination of puerarin and canagliflozin (Fig. 4). These results confirm the renoprotective effect of canagliflozin and demonstrate that canagliflozin with puerarin treatment results in the remission of lipotoxicity-induced oxidative stress and the inflammatory response in the kidneys.
Glucose and lipid metabolism disorders play a key role in the occurrence and development of diseases. Furthermore, dyslipidemia induced by hyperglycemia contributes to the occurrence and progression of diabetic nephropathy [9,16]. A previous study found that the incidence of chronic kidney disease was significantly reduced in patients with dyslipidemia after hypolipidemic treatment [17], indicating that dyslipidemia may induce renal injury by mechanisms dependent on or independent of hyperglycemia. Lipotoxicity caused by abnormal lipid metabolism in the body is a destructive process caused by lipid accumulation in nonadipose tissue, which leads to cellular oxidative stress and inflammatory responses. These changes are closely related to the pathogenesis of diabetic kidney disease, explaining why the incidence of chronic kidney disease is still high in diabetic patients with well-controlled blood glucose. Our study confirmed the abnormal serum lipid metabolism and lipid accumulation in the kidneys of mice with diabetes, providing strong evidence that lipotoxicity is involved in the occurrence and development of diabetic kidney disease and thus represents a new intervention target for treating diabetic kidney disease.
In this study, we treated diabetic mice with 12 weeks of canagliflozin and/or puerarin and found that all interventions were effective in reducing blood glucose and alleviating diabetes symptoms in mice, but puerarin alone could not achieve complete remission of diabetic symptoms. Moreover, our experimental results showed that the puerarin intervention ameliorated lipid metabolism in both the serum and kidney in diabetic mice, which is consistent with previous research results on puerarin [18,19], and verified the improved clinical outcome of Gegen regarding chronic metabolic diseases. In addition, studies have shown that puerarin can inhibit Guanine nucleotide-binding protein Gi alpha-1, activate the cAMP/PKA/CREB pathway in renal podocytes, and reduce podocyte apoptosis induced by high glucose [20]. Puerarin can also inhibit the oxidative stress process of the endoplasmic reticulum in podocytes to play a renal protective role [21]. Combining above studies with our results, it is suggested that the mechanism of puerarin is possibly achieved by regulating various physiological functions of podocytes. In our results, the combination of canagliflozin and puerarin showed a significant anti-lipotoxicity effect, which indicates that the puerarin in combination with canagliflozin treatment may improve the prognosis and enhance the quality of life of patients with diabetes.
In this study, a preclinical mouse model was used to verify the positive effect of SGLT2 inhibitors on kidney protection in clinical practice. Our research revealed a new renoprotective pathway of SGLT2 inhibitors, in which the mechanisms are possibly via the reduction of renal filtration and reabsorption pressure by well-controlled blood glucose [22] and the alleviation of glomerular basement membrane thickening. We also found that the parameters of serum lipid metabolism and renal lipid accumulation improved after canagliflozin treatment, as indicated by the amelioration of glucose metabolism. When combined with puerarin, the renoprotective effect was enhanced. The results showed changes in the serum renal function parameters of occurred later, and the degree of the damage was reduced in the combination of canagliflozin and puerarin group, compared with the canagliflozin group, or the puerarin group, or the diabetes group. Glomerular basement membrane thickening and renal fibrosis were milder, and the levels of oxidative stress and inflammation were significantly decreased in the puerarin + canagliflozin group, compared with the untreated diabetes group and other treatment groups. Such results are consistent with the findings of previous studies on the renoprotection of SGLT2 inhibitors [23-26] and the antioxidant and anti-inflammatory effects of puerarin [27-30].
The current clinical treatment of diabetic kidney disease most frequently focuses on symptomatic treatment, while prevention of its occurrence has received much less attention. In this research, we showed that administering puerarin with the SGLT2 inhibitor canagliflozin at the initial diagnosis stage of diabetes can effectively delay the occurrence of diabetic kidney disease and significantly reduce the degree of damage by reducing renal lipotoxicity in a mouse model. This study demonstrates prevention of diabetic kidney disease is on the horizon. However, more research is essential to replicate and strengthen these results. These findings also offer strong evidence for the need of future research to explore safety and synergy assessments.
Notes
SUPPLEMENTARY MATERIALS
Supplementary data including four tables can be found with this article online at https://doi.org/10.4196/kjpp.2023.27.3.221.
REFERENCES
1. Koye DN, Magliano DJ, Nelson RG, Pavkov ME. 2018; The global epidemiology of diabetes and kidney disease. Adv Chronic Kidney Dis. 25:121–132. DOI: 10.1053/j.ackd.2017.10.011. PMID: 29580576.


2. Li H, Lu W, Wang A, Jiang H, Lyu J. 2021; Changing epidemiology of chronic kidney disease as a result of type 2 diabetes mellitus from 1990 to 2017: estimates from Global Burden of Disease 2017. J Diabetes Investig. 12:346–356. DOI: 10.1111/jdi.13355. PMID: 32654341. PMCID: PMC7926234. PMID: 731ea2cc6b88450b88b4ce87998d2520.


3. Gregg EW, Williams DE, Geiss L. 2014; Changes in diabetes-related complications in the United States. N Engl J Med. 371:286–287. DOI: 10.1056/NEJMc1406009. PMID: 25014698.


4. Zinman B, Wanner C, Lachin JM, Fitchett D, Bluhmki E, Hantel S, Mattheus M, Devins T, Johansen OE, Woerle HJ, Broedl UC, Inzucchi SE. EMPA-REG OUTCOME Investigators. 2015; Empagliflozin, cardiovascular outcomes, and mortality in type 2 diabetes. N Engl J Med. 373:2117–2128. DOI: 10.1056/NEJMoa1504720. PMID: 26378978.


5. Neal B, Perkovic V, Matthews DR. 2017; Canagliflozin and cardiovascular and renal events in type 2 diabetes. N Engl J Med. 377:2099. DOI: 10.1056/NEJMc1712572. PMID: 29166232.


6. Wiviott SD, Raz I, Bonaca MP, Mosenzon O, Kato ET, Cahn A, Silverman MG, Zelniker TA, Kuder JF, Murphy SA, Bhatt DL, Leiter LA, McGuire DK, Wilding JPH, Ruff CT, Gause-Nilsson IAM, Fredriksson M, Johansson PA, Langkilde AM, Sabatine MS. DECLARE-TIMI 58 Investigators. 2019; Dapagliflozin and cardiovascular outcomes in type 2 diabetes. N Engl J Med. 380:347–357. DOI: 10.1056/NEJMoa1812389. PMID: 30415602.


7. Perkovic V, Jardine MJ, Neal B, Bompoint S, Heerspink HJL, Charytan DM, Edwards R, Agarwal R, Bakris G, Bull S, Cannon CP, Capuano G, Chu PL, de Zeeuw D, Greene T, Levin A, Pollock C, Wheeler DC, Yavin Y, Zhang H, et al. CREDENCE Trial Investigators. 2019; Canagliflozin and renal outcomes in type 2 diabetes and nephropathy. N Engl J Med. 380:2295–2306. DOI: 10.1056/NEJMoa1811744. PMID: 30990260.


8. Nespoux J, Vallon V. 2020; Renal effects of SGLT2 inhibitors: an update. Curr Opin Nephrol Hypertens. 29:190–198. DOI: 10.1097/MNH.0000000000000584. PMID: 31815757. PMCID: PMC7224333.
9. Thongnak L, Pongchaidecha A, Lungkaphin A. 2020; Renal lipid metabolism and lipotoxicity in diabetes. Am J Med Sci. 359:84–99. DOI: 10.1016/j.amjms.2019.11.004. PMID: 32039770.


10. Yuan G, Shi S, Jia Q, Shi J, Shi S, Zhang X, Shou X, Zhu X, Hu Y. 2021; Use of network pharmacology to explore the mechanism of gegen (Puerariae lobatae Radix) in the treatment of type 2 diabetes mellitus associated with hyperlipidemia. Evid Based Complement Alternat Med. 2021:6633402. DOI: 10.1155/2021/6633402. PMID: 33953784. PMCID: PMC8068526.
11. Wang S, Zhang S, Wang S, Gao P, Dai L. 2020; A comprehensive review on Pueraria: insights on its chemistry and medicinal value. Biomed Pharmacother. 131:110734. DOI: 10.1016/j.biopha.2020.110734. PMID: 32942158.
12. Jiang Z, Cui X, Qu P, Shang C, Xiang M, Wang J. 2022; Roles and mechanisms of puerarin on cardiovascular disease: a review. Biomed Pharmacother. 147:112655. DOI: 10.1016/j.biopha.2022.112655. PMID: 35066299.
13. Cansby E, Caputo M, Gao L, Kulkarni NM, Nerstedt A, Ståhlman M, Borén J, Porosk R, Soomets U, Pedrelli M, Parini P, Marschall HU, Nyström J, Howell BW, Mahlapuu M. 2020; Depletion of protein kinase STK25 ameliorates renal lipotoxicity and protects against diabetic kidney disease. JCI Insight. 5:e140483. DOI: 10.1172/jci.insight.140483. PMID: 33170807. PMCID: PMC7819747.


14. Terami N, Ogawa D, Tachibana H, Hatanaka T, Wada J, Nakatsuka A, Eguchi J, Horiguchi CS, Nishii N, Yamada H, Takei K, Makino H. 2014; Long-term treatment with the sodium glucose cotransporter 2 inhibitor, dapagliflozin, ameliorates glucose homeostasis and diabetic nephropathy in db/db mice. PLoS One. 9:e100777. DOI: 10.1371/journal.pone.0100777. PMID: 24960177. PMCID: PMC4069074. PMID: 95f390caebe0425d811aad5116700659.


15. Hatanaka T, Ogawa D, Tachibana H, Eguchi J, Inoue T, Yamada H, Takei K, Makino H, Wada J. 2016; Inhibition of SGLT2 alleviates diabetic nephropathy by suppressing high glucose-induced oxidative stress in type 1 diabetic mice. Pharmacol Res Perspect. 4:e00239. DOI: 10.1002/prp2.239. PMID: 28116093. PMCID: PMC5242174.


16. Nakamichi R, Hayashi K, Itoh H. 2021; Effects of high glucose and lipotoxicity on diabetic podocytes. Nutrients. 13:241. DOI: 10.3390/nu13010241. PMID: 33467659. PMCID: PMC7830342. PMID: d0ed4289173d4bc1a268eac1cb9cb57f.


17. You HS, Shin SJ, Kim J, Kang HT. 2021; Statin use and incidence of chronic kidney disease in hypercholesterolemia patients with normal renal function. Am J Nephrol. 52:940–948. DOI: 10.1159/000520532. PMID: 34864729.


18. Wan XM, Chen J, Wang M, Zheng C, Zhou XL. 2021; Puerarin attenuates cadmium-induced hepatic lipid metabolism disorder by inhibiting oxidative stress and inflammation in mice. J Inorg Biochem. 222:111521. DOI: 10.1016/j.jinorgbio.2021.111521. PMID: 34171769.


19. Noh JW, Yang HK, Jun MS, Lee BC. 2022; Puerarin attenuates obesity-induced inflammation and dyslipidemia by regulating macrophages and TNF-alpha in obese mice. Biomedicines. 10:175. DOI: 10.3390/biomedicines10010175. PMID: 35052852. PMCID: PMC8773888. PMID: 0c68260fe5e9449287a0ad18973b6c98.


20. Zhu Q, Yang S, Wei C, Lu G, Lee K, He JC, Liu R, Zhong Y. 2022; Puerarin attenuates diabetic kidney injury through interaction with Guanidine nucleotide-binding protein Gi subunit alpha-1 (Gnai1) subunit. J Cell Mol Med. 26:3816–3827. DOI: 10.1111/jcmm.17414. PMID: 35678269. PMCID: PMC9279604.


21. Xu X, Chen B, Huang Q, Wu Y, Liang T. 2020; The effects of puerarin on autophagy through regulating of the PERK/eIF2α/ATF4 signaling pathway influences renal function in diabetic nephropathy. Diabetes Metab Syndr Obes. 13:2583–2592. DOI: 10.2147/DMSO.S256457. PMID: 32765037. PMCID: PMC7381766.
22. Tauber P, Sinha F, Berger RS, Gronwald W, Dettmer K, Kuhn M, Trum M, Maier LS, Wagner S, Schweda F. 2021; Empagliflozin reduces renal hyperfiltration in response to uninephrectomy, but is not nephroprotective in UNx/DOCA/Salt mouse models. Front Pharmacol. 12:761855. DOI: 10.3389/fphar.2021.761855. PMID: 34992532. PMCID: PMC8724563. PMID: 425b5cc5bd5d407aabe01f2b4f8ecc5e.


23. Locatelli M, Zoja C, Conti S, Cerullo D, Corna D, Rottoli D, Zanchi C, Tomasoni S, Remuzzi G, Benigni A. 2022; Empagliflozin protects glomerular endothelial cell architecture in experimental diabetes through the VEGF-A/caveolin-1/PV-1 signaling pathway. J Pathol. 256:468–479. DOI: 10.1002/path.5862. PMID: 35000230.


24. Alkabbani W, Zongo A, Minhas-Sandhu JK, Eurich DT, Shah BR, Alsabbagh MW, Gamble JM. 2021; Renal effectiveness and safety of the sodium-glucose cotransporter-2 inhibitors: a population-based cohort study. BMJ Open Diabetes Res Care. 9:e002496. DOI: 10.1136/bmjdrc-2021-002496. PMID: 34906925. PMCID: PMC8671915.


25. Yang L, Liang B, Li J, Zhang X, Chen H, Sun J, Zhang Z. 2022; Dapagliflozin alleviates advanced glycation end product induced podocyte injury through AMPK/mTOR mediated autophagy pathway. Cell Signal. 90:110206. DOI: 10.1016/j.cellsig.2021.110206. PMID: 34856357.


26. Chun KJ, Jung HH. 2021; SGLT2 inhibitors and kidney and cardiac outcomes according to estimated GFR and albuminuria levels: a meta-analysis of randomized controlled trials. Kidney Med. 3:732–744.e1. DOI: 10.1016/j.xkme.2021.04.009. PMID: 34746739. PMCID: PMC8551546.


27. Jeon YD, Lee JH, Lee YM, Kim DK. 2020; Puerarin inhibits inflammation and oxidative stress in dextran sulfate sodium-induced colitis mice model. Biomed Pharmacother. 124:109847. DOI: 10.1016/j.biopha.2020.109847. PMID: 31981944.


28. Li X, Cai W, Lee K, Liu B, Deng Y, Chen Y, Zhang X, He JC, Zhong Y. 2017; Puerarin attenuates diabetic kidney injury through the suppression of NOX4 expression in podocytes. Sci Rep. 7:14603. DOI: 10.1038/s41598-017-14906-8. PMID: 29097815. PMCID: PMC5668268.


29. Zhang Y, Yang X, Ge X, Zhang F. 2019; Puerarin attenuates neurological deficits via Bcl-2/Bax/cleaved caspase-3 and Sirt3/SOD2 apoptotic pathways in subarachnoid hemorrhage mice. Biomed Pharmacother. 109:726–733. DOI: 10.1016/j.biopha.2018.10.161. PMID: 30551525.


30. Xiao L, Zhong M, Huang Y, Zhu J, Tang W, Li D, Shi J, Lu A, Yang H, Geng D, Li H, Wang Z. 2020; Puerarin alleviates osteoporosis in the ovariectomy-induced mice by suppressing osteoclastogenesis via inhibition of TRAF6/ROS-dependent MAPK/NF-κB signaling pathways. Aging (Albany NY). 12:21706–21729. DOI: 10.18632/aging.103976. PMID: 33176281. PMCID: PMC7695364.


Fig. 1
Effect of canagliflozin and puerarin on body weight and hyperglycemia.
(A) Body weight in control and diabetic mice with different treatments at the end of the 22nd week. (B) Blood glucose in control mice and diabetic mice with different treatments at the end of the 22nd week. (C) Body weight time course in control mice and diabetic mice with different treatments. (D) Blood glucose time course in control mice and diabetic mice with different treatments. Data are shown as mean ± SD (n = 6). #p < 0.05, ##p < 0.01 vs. control group; *p < 0.05, **p < 0.01 vs. diabetes group; ^p < 0.05, ^^p < 0.01 vs. canagliflozin group.
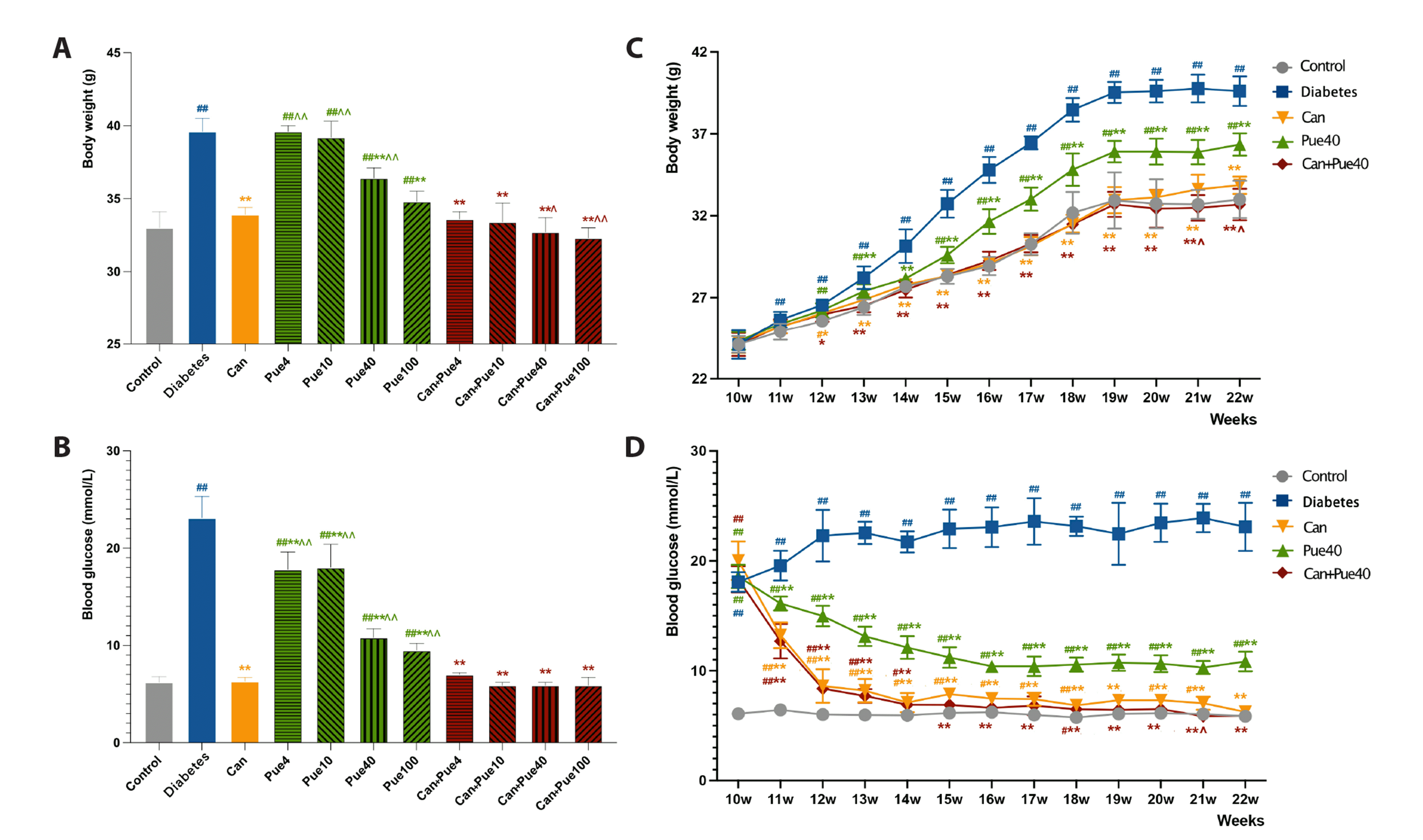
Fig. 2
Effect of canagliflozin and puerarin on functional and structural parameters of kidneys.
(A) Effect of canagliflozin and puerarin on urinary albumin excretion. (B) Representative image of HE (100×), PAS (400×), and picrosirius red (100×) staining of kidney sections. (C) PAS-positive area in glomeruli. (D) Percentages of picrosirius red-positive areas. Data are shown as mean ± SD (n = 6). HE, hematoxylin and eosin; PAS, periodic acid-Schiff. ##p < 0.01 vs. control group; **p < 0.01 vs. diabetes group; ^^p < 0.01 vs. canagliflozin group.

Fig. 3
Effect of canagliflozin and puerarin on lipid homeostasis of kidneys.
(A) Representative image of oil red O staining (100×) of kidney sections. (B) Oil red O-positive area in the kidney. (C) Western blot of CD36, adipophilin, and PPARα in the kidney. (D) Relative expression of CD36. (E) Relative expression of adipophilin. (F) Relative expression of PPARα. Data are shown as mean ± SD (n = 6). #p < 0.05, ##p < 0.01 vs. control group; **p < 0.01 vs. diabetes group; ^^p < 0.01 vs. canagliflozin group.

Fig. 4
Effect of puerarin in combination with canagliflozin on oxidative stress and the inflammatory response of kidneys.
(A) MDA level in the kidney. (B) GPx activity in the kidney. (C) SOD activity in the kidney. (D) CAT activity in the kidney. (E) GSH levels in the kidney. (F) Western blot of TNFα, IFNγ, IL6, and IL1β in the kidney. (G) Relative expression of TNFα. (H) Relative expression of IFNγ. (I) Relative expression of IL6. (J) Relative expression of IL1β. Data are shown as mean ± SD (n = 6). MDA, malondialdehyde; GPx, glutathione peroxidase; SOD, superoxide dismutase; CAT, catalase; GSH, glutathione; TNFα, tumor necrosis factor α; IFNγ, interferon γ; IL, interleukin. #p < 0.05, ##p < 0.01 vs. control group; *p < 0.05, **p < 0.01 vs. diabetes group.
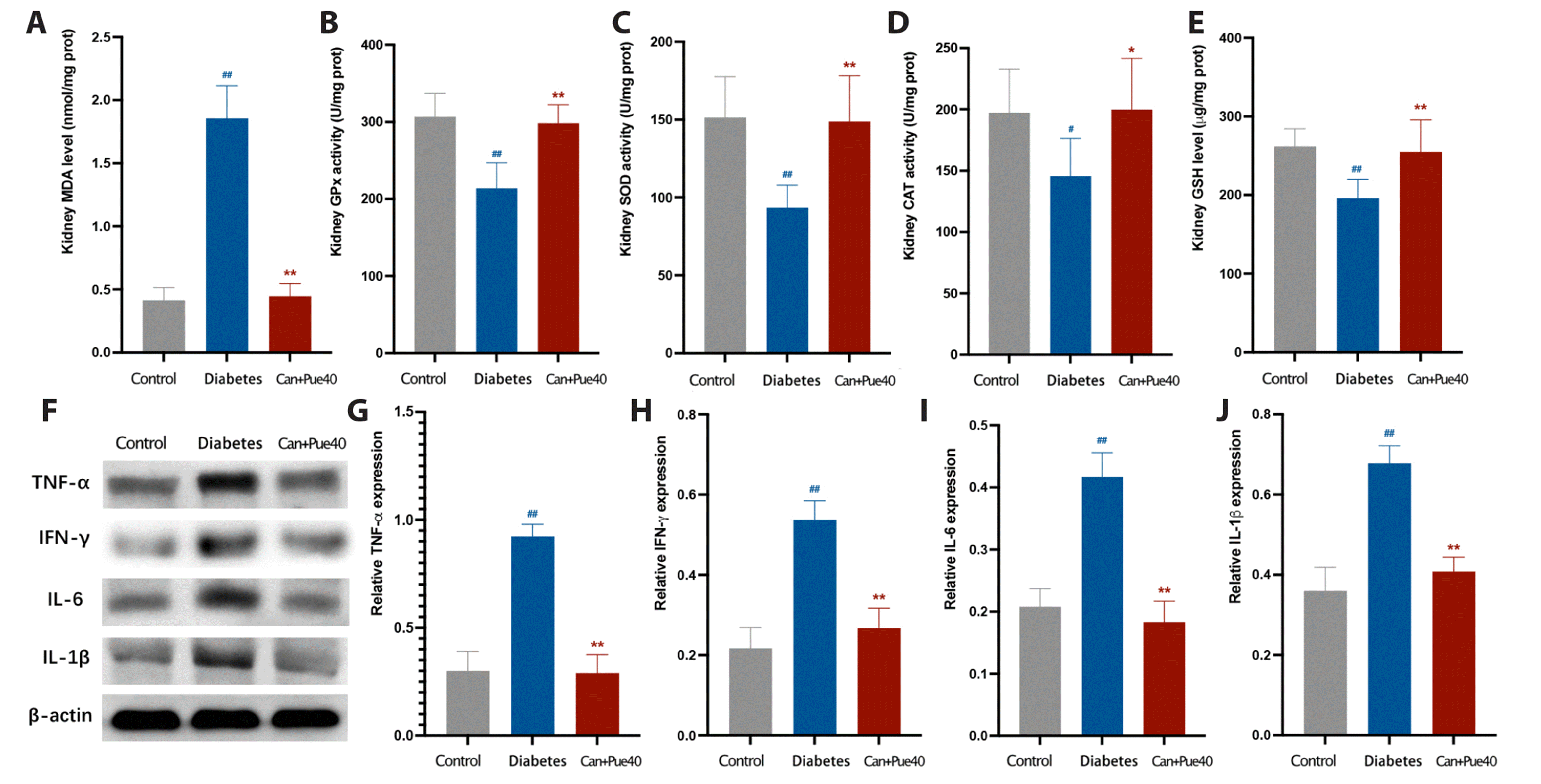
Table 1
Influence of canagliflozin and puerarin on lipid metabolic parameters in diabetes mice at the end of treatment (22 weeks of age)
Parameter | Control | Diabetes | Can | Pue (mg/kg) | Can + Pue (mg/kg of Pue) | |||||||
---|---|---|---|---|---|---|---|---|---|---|---|---|
4 | 10 | 40 | 100 | 4 | 10 | 40 | 100 | |||||
TC (mM) | 5.15 ± 0.29 | 7.34 ± 0.34## | 6.80 ± 0.15##** | 6.88 ± 0.21##** | 6.46 ± 0.26##**^ | 6.14 ± 0.23##**^^ | 5.47 ± 0.20#**^^ | 6.54 ± 0.27##** | 6.13 ± 0.22##**^^ | 5.25 ± 0.29**^^ | 5.18 ± 0.25**^^ | |
LDL-C (mM) |
2.57 ± 0.15 | 3.97 ± 0.08## | 3.54 ± 0.12##** | 3.75 ± 0.07##*^ | 3.69 ± 0.08##** | 3.12 ± 0.10##**^^ | 3.02 ± 0.12##**^^ | 3.42 ± 0.09##** | 3.22 ± 0.12##**^^ | 2.58 ± 0.22**^^ | 2.67 ± 0.29**^^ | |
HDL-C (mM) |
2.90 ± 0.08 | 1.97 ± 0.14## | 2.22 ± 0.11##** | 2.43 ± 0.19##**^^ | 2.49 ± 0.13##**^^ | 2.66 ± 0.08##**^^ | 2.53 ± 0.08##**^^ | 2.56 ± 0.18##**^^ | 2.57 ± 0.17##**^^ | 2.83 ± 0.08**^^ | 2.86 ± 0.12**^^ | |
TG (mM) | 2.33 ± 0.12 | 3.23 ± 0.04## | 3.02 ± 0.08##* | 3.03 ± 0.14##* | 2.61 ± 0.11##**^^ | 2.89 ± 0.08##** | 2.19 ± 0.10**^^ | 2.84 ± 0.16##**^ | 2.48 ± 0.14**^^ | 2.36 ± 0.17**^^ | 2.18 ± 0.26**^^ |
Table 2
Influence of canagliflozin and puerarin on functional and structural parameters of kidneys in diabetes mice at the end of treatment (22 weeks of age)
Parameter | Control | Diabetes | Can | Pue40 | Can + Pue40 |
---|---|---|---|---|---|
Kidney weight (mg) | 291.3 ± 9.4 | 462.0 ± 10.3## | 342.1 ± 12.0##** | 346.7 ± 45.36##** | 311.6 ± 4.8**^ |
Relative kidney weight (mg/g body weight) |
8.8 ± 0.3 | 11.7 ± 0.3## | 10.1 ± 0.4##** | 9.5 ± 1.3** | 9.5 ± 0.4** |
BUN (mg/dl) | 8.6 ± 1.0 | 20.2 ± 1.3## | 15.3 ± 0.5##** | 14.6 ± 0.9##** | 9.5 ± 0.9**^^ |
Serum creatinine (mg/dl) | 0.40 ± 0.04 | 0.93 ± 0.08## | 0.70 ± 0.03##** | 0.58 ± 0.10##** | 0.47 ± 0.04**^^ |