Abstract
Brain metastasis (BM), classified as a secondary brain tumor, is the most common malignant central nervous system tumor whose median overall survival is approximately 6 months. However, the survival rate of patients with BMs has increased with recent advancements in immunotherapy and targeted therapy. This means that clinicians should take a more active position in the treatment paradigm that passively treats BMs. Because patients with BM are treated in a variety of clinical settings, treatment planning requires a more sophisticated decision-making process than that for other primary malignancies. Therefore, an accurate prognostic prediction is essential, for which a graded prognostic assessment that reflects next-generation sequencing can be helpful. It is also essential to understand the indications for various treatment modalities, such as surgical resection, stereotactic radiosurgery, and whole-brain radiotherapy and consider their advantages and disadvantages when choosing a treatment plan. Surgical resection serves a limited auxiliary function in BM, but it can be an essential therapeutic approach for increasing the survival rate of specific patients; therefore, this must be thoroughly recognized during the treatment process. The ultimate goal of surgical resection is maximal safe resection; to this end, neuronavigation, intraoperative neuro-electrophysiologic assessment including evoked potential, and the use of fluorescent materials could be helpful. In this review, we summarize the considerations for neurosurgical treatment in a rapidly changing treatment environment.
Metastatic brain tumors are the most common type of malignant brain tumors in adults [123]. The incidence of brain metastasis (BM) has recently increased [45]. This is because of the advancement of cancer therapies, prolonged patient survival, and strengthening of screening protocols [4]. Although the exact occurrence of BMs varies slightly in the literature, it has recently been reported as 10%–40% and up to 25% in autopsy studies [2367]. BM requires aggressive treatment because it not only causes convulsions, paralysis, and speech impairment but can also affect patient survival. Even in palliative settings, it is crucial to address these symptoms, and the role of neurosurgery in BM was first demonstrated in a randomized controlled study conducted by Patchell et al. [8]. For single BM lesions, a study randomized between a radiation-only group and the surgery with radiation group showed a median survival of 15 weeks in the former and 40 weeks in the latter, indicating a significant survival benefit [8]. Bindal et al. [6] showed a statistically significant survival benefit when all tumors were surgically removed in multiple BM cases compared to those with residual tumors with a median survival similar to that of patients with a single metastasis.
Owing to the nature of the disease, BM can be caused by diverse carcinomas and presents different clinical situations for each patient. Therefore, building a personalized treatment plan is crucial, and physicians must consider the individual aspects during this process. These factors are represented by clinical variables such as patient age, performance status, BM number and size, neurological symptoms, extracranial metastases, and genetic alterations [9]. Surgical resection, radiotherapy such as stereotactic radiosurgery (SRS) and whole-brain radiotherapy (WBRT), and systemic therapies including targeted therapy and immunotherapeutic agents, are used to treat BM [1011]. This review summarizes considerations for the surgical resection of BM, selection of treatment modalities, and surgical tips.
Predicting prognosis is among the most important aspects of BM treatment [9]. For neurosurgeons, the option of surgical resection includes general anesthesia; therefore, there must be a clear benefit. Surgical resection is generally recommended for patients with an expected survival period of at least three months and should be chosen according to diverse clinical situations [12]. Steady efforts have been made to develop a prognostic prediction system (Table 1).
The original work of the prognostic prediction system is a recursive partitioning analysis (RPA) of the Radiation Oncology Treatment Group (RTOG) published by Gaspar et al. [13], and 1,200 patients included in three clinical trials from 1979 to 1993 were analyzed. According to the RPA tree, age, Karnofsky performance score (KPS), systemic disease status, and the presence of extracranial metastasis (ECM) reportedly affect prognosis. In this study, patients with KPS of 70 or higher, controlled systemic disease, age <65 years, and no ECM were classified as Class I; those with a KPS <70 as Class III; and other applicable patients as Class II. This system can easily stratify patients using simple variables to predict prognosis, but it is not currently used and remains a legacy because it is not disease-specific, and current systemic therapies are not reflected [9].
Sperduto et al. [9] retrospectively analyzed 3,940 patients with newly diagnosed BM and published diagnosis-specific graded prognostic assessment (GPA) scores. Following this, specific GPAs for each cancer type were announced in the following order: breast cancer (breast-GPA) [14], lung cancer (lung-molGPA) [15], melanoma (melanoma-molGPA) [16], renal cell carcinoma (renal-GPA) [17], and gastrointestinal cancer (GI-GPA) [18]. Prognostic factors were evaluated using weights through multiple Cox regression analysis and RPA methods, and the largest change was that genetic alterations were included [14]. Genes associated with prognostic factors differ among cancer types and include non-small cell lung cancer (epidermal growth factor [EGFR] or anaplastic lymphoma kinase [ALK] alteration), breast cancer (estrogen receptor [ER], progesterone receptor [PR], human epidermal growth factor receptor 2 [HER2]), and melanoma (BRAF) [141516]. This shift is attributed to the development of effective targeted therapies for specific gene abnormalities for each carcinoma, which has a substantial impact on patient prognosis. Immunotherapy has recently become popular and is widely applied, especially for lung cancer and melanoma. Predictive factors in immunotherapy can also be included as prognostic factors in the future [1920]. This prognostic prediction system will be updated continuously. Therefore, neurosurgeons should apply these changes with sensitivity when treating patients or designing clinical studies.
As mentioned above, combined with systemic chemotherapy, localized therapies for BM include surgical resection, SRS, and WBRT [12]. The most important factors in determining the treatment method are the number of BM and performance status. In a single metastasis situation, if the tumor is accessible and the patient has RPA Class I or II, surgical treatment and adjuvant WBRT or adjuvant SRS are recommended [1221]. In cases of RPA Class III, SRS or WBRT without surgical treatment is recommended [122223]. If the tumor location is not accessible, SRS is recommended for RPA Class I or II, and WBRT is preferred for RPA Class III [12]. In cases of multiple BM, treatment methods are largely classified based on BM number (n>4), and surgical treatment is recommended only when the tumor causes a mass effect and is located in an accessible lesion [1221]. SRS or WBRT is recommended if the patient’s performance is unsatisfactory and the BM does not cause a mass effect. In some cases, SRS followed by WBRT can be considered [12]. Due to the similarity between clinical variables used to determine treatment modality and prognostic prediction systems such as GPA, it is easy to become confused. However, the decision flow of the two systems is entirely distinct, so it is essential to fully comprehend it.
Since surgical treatment can bring rapid resolution of the mass effect in tumors larger than 3–4 cm, it has the advantage of being able to drastically reduce the steroid requirement and minimize radiation necrosis that may occur after radiation [5]. However, SRS can be applied to relatively small tumors and has the advantage of being delivered on an outpatient basis or during a short hospital stay without requiring general anesthesia [5]. In addition, although WBRT appears effective in almost all tumors, the neurocognitive decline that may occur after treatment has recently attracted attention [2425]. A large meta-analysis by Tallet et al. [26] reported decreases in neurocognitive function of 31%–57% within 3 months and 48%–89% in 1 year. This study reported that radiation exposure to hippocampal lesions and the temporal lobe could reduce a patient’s intelligence quotient and capacity to generate new memories. To avoid this cognitive decline, hippocampal preservation or prophylactic administration of memantine may be used [2728].
Although the role of surgical treatment in BM treatment is limited, it appears to have a clear survival benefit in patients with good performance status and single or oligometastases [12]. In addition, since performance status is one of the most important prognostic factors in patients with BM, the most important goal of surgical treatment is maximal safe resection without neurological deficits. To achieve this goal, various recently developed neurosurgical modalities can be used.
First, the positional relationship between metastatic brain tumors and the functional tract should be investigated using neuronavigation and diffusion tensor imaging tractography [29]. The corticospinal tract (CST), which can affect motor function, is a representative tract requiring preservation (Fig. 1). In addition, recent language tracts are related to paraphasia, word recognition, and conditional associative tasks such as the superior longitudinal fasciculus I, II, III, and uncinate fasciculus, which can also affect language function [30]. It is recommended that we fully understand the three-dimensional anatomical relationship of the language tract during surgery and carefully remove the tumor. Tumors invading the temporal stem could affect the visual pathway and cause symptoms such as hemianopia and quadrantanopia, so this should be sufficiently discussed with patients before surgery. One consideration of neuronavigation during surgery is that accuracy can change intraoperatively due to brain shifting, shallow anesthesia, or brain swelling [31]. Therefore, it is necessary to compare the depth of the cerebral vein or the brain’s surface, which can be landmarks, to evaluate and maintain accuracy during surgery. Furthermore, by setting a rescue point during the surgery, when shaking occurs, accuracy can be increased through re-registration to protect the surgeon from navigation errors [32].
Since the occurrence of neurological symptoms after surgery could impair the performance status of patients with BM, particular attention is needed. Numerous types of surveillance devices can preserve patient functionality, including motor evoked potentials (EPs), somatosensory EPs, visual EPs, and brainstem auditory EPs [33343536]. EPs can be used to compare neurologic deficits after surgery versus before tumor removal; however, it has disadvantages in that it is difficult to predict the functional tract before tumor removal and difficult to measure the change in EP in real-time [33]. For simultaneous monitoring, subcortical stimulation (SCS) mapping can also be used in asleep and awake surgery [3738]. Since the use of SCS during brain surgery was reported by Duffau et al. [3940], it has been widely applied to various patients, such as glioma patients, and the intraoperative procedure used for SCS has been described in detail elsewhere. SCS is a simple method of depolarizing the CST using a monopolar stimulator to detect muscle movement with proven safety [4041]. Therefore, SCS-assisted BM resection could help preserve function postoperatively (Fig. 2). Multichannel electromyography recordings can increase the sensitivity of detecting muscle movements [42]. Recent studies suggested that the current strength (mA) and the distance between the CST (mm) correspond almost 1:1 [4344]. For example, if stimulation is sensed at 5 mA before tumor removal, the predicted distance between the monopolar stimulator and the CST is approximately 5 mm. If muscle movement is detected at a lower than expected current, the CST may be located closer to that observed during navigation. This method will further reduce postoperative complications and contribute to maintaining patient performance status.
In addition to the above methods, to increase resection extent, staining materials that can differentiate between tumor and normal tissue, called fluorescence-guided surgery, are used (Fig. 3A and B). In particular, sodium fluorescein is highly accumulated in enhancing lesions where the blood-brain barrier is disrupted, distinguishing it from the normal parenchyma under a yellow 560 nm surgical microscope filter, which enables tumor visualization [4546]. It is usually used at a concentration of 5 mg/kg to stain tumors and is administered through an intravenous injection after completion of the procedure under general anesthesia [46]. During resection, the operator can view the tumor in real-time at high resolution using a microscope. In the course of BM surgery using sodium fluorescein, there was a strong correlation between the stained area and enhancement on magnetic resonance imaging with a reported sensitivity of 91% and specificity of 100% [46]. In fact, 5-aminolevulinic acid, which is widely used in glioma surgery, is also not widely used because it is expensive and has distinct detection rates for different primary cancer types (Fig. 3C and D). In a prospective study of 157 BMs, fluorescence was observed in only 104 patients (66%), and the fluorescence pattern was inconsistent. In particular, fluorescence was observed in 73% of breast cancer cases, but fluorescence was observed in only 33% of melanoma cases, and there was a statistically significant difference between carcinomas, resulting in inconsistency [47]. Additionally, in approximately two-thirds of cases, histological analysis of biopsies obtained from residual fluorescent areas during BM resection revealed false-positive results [48].
The BM was previously thought to be distinct from the surrounding tissues. However, most BMs do not have clear boundaries with the surrounding normal parenchyma, and some cases show an invasive growth pattern [484950]. Therefore, after gross total resection (GTR) has been achieved, the extent of resection can be increased by additional removal of approximately 5 mm; this method has been named microscopic GTR (MTR) or supramarginal resection [5152]. This method is applicable to BM that occurs in non-eloquent areas and can be implemented with methods such as functional brain mapping using SCS [52]. Yoo et al. [51] observed a local recurrence rate of 23.3% in the MTR group and 43.1% in the GTR group and reported a clear local control effect. The use of this strategy for BM in the eloquent area and its contribution to overall survival are unclear; therefore, this should be investigated in greater depth in future research.
A novel technique called laser interstitial thermal therapy (LITT) was recently reported for its clinical utility since it was first applied to BM in 2010 [535455]. Briefly, LITT is a localized minimally invasive method that attempts to kill cancer cells by placing a stereotactically placed laser probe on the tumor and delivering high thermal energy [55]. It is widely applied in BM, with a short recovery term and a relatively simple procedure [54]. This is preferred for patients with deeply seated tumors, patients with expected high morbidity, a thin scalp, and low-performance status, meaning that they are not surgical candidates, and it can be applied as an adjuvant therapy in radioresistant tumors [54].
In addition, some groups are trying neoadjuvant SRS, noting that WBRT or adjuvant SRS, which is performed after surgical resection, reports rates of about 7.5%–14% leptomeningeal seeding (LMS) in BM [56]. Conceptually, neoadjuvant GKS before surgery is used to reduce viable tumor cells, so that even microtumor cells that are invisible during surgery overflowing into the surrounding arachnoid space are not expected to lead to colonization. Asher et al. [56] reported a local control rate of 86%, a distant control rate of 38%, and LMS of 0% in a study of 47 patients. Thus, it may be an alternative to adjuvant GKS or adjuvant WBRT in radio-resistant tumors such as melanoma and renal cell carcinoma, but caution is required for application as no survival benefit has been observed in large-scale clinical studies.
BM is clearly a desperate situation for cancer patients; however, with the development of systemic treatment options, more aggressive treatment is recommended. In particular, the surgical treatment of BM remains important because it is expected to alleviate the patient’s symptoms by immediately eliminating the mass effect. For surgical success, neurosurgeons must decide which patients are appropriate candidates. In the rapidly developing neurosurgical armamentarium, the application of fluorescein, neuro-electrophysiological surveillance, and neuronavigation systems is expected to make a significant contribution to maximal safe resection. Consequently, successful surgical resection may prolong cancer patient survival and provide opportunities for them to benefit from novel therapies.
Acknowledgments
The authors would like to thank Editage (www.editage.co.kr) for English language editing.
Availability of Data and Material
Data sharing not applicable to this article as no datasets were generated during the study.
References
1. Artzi M, Bressler I, Ben Bashat D. Differentiation between glioblastoma, brain metastasis and subtypes using radiomics analysis. J Magn Reson Imaging. 2019; 50:519–528. PMID: 30635952.
2. Nayak L, Lee EQ, Wen PY. Epidemiology of brain metastases. Curr Oncol Rep. 2012; 14:48–54. PMID: 22012633.
3. Stelzer KJ. Epidemiology and prognosis of brain metastases. Surg Neurol Int. 2013; 4(Suppl 4):S192–S202. PMID: 23717790.
4. Fecci PE, Champion CD, Hoj J, McKernan CM, Goodwin CR, Kirkpatrick JP, et al. The evolving modern management of brain metastasis. Clin Cancer Res. 2019; 25:6570–6580. PMID: 31213459.
5. Suh JH. Stereotactic radiosurgery for the management of brain metastases. N Engl J Med. 2010; 362:1119–1127. PMID: 20335588.
6. Bindal RK, Sawaya R, Leavens ME, Lee JJ. Surgical treatment of multiple brain metastases. J Neurosurg. 1993; 79:210–216. PMID: 8331402.
7. Walker AE, Robins M, Weinfeld FD. Epidemiology of brain tumors: the national survey of intracranial neoplasms. Neurology. 1985; 35:219–226. PMID: 3969210.
8. Patchell RA, Tibbs PA, Walsh JW, Dempsey RJ, Maruyama Y, Kryscio RJ, et al. A randomized trial of surgery in the treatment of single metastases to the brain. N Engl J Med. 1990; 322:494–500. PMID: 2405271.
9. Sperduto PW, Kased N, Roberge D, Xu Z, Shanley R, Luo X, et al. Summary report on the graded prognostic assessment: an accurate and facile diagnosis-specific tool to estimate survival for patients with brain metastases. J Clin Oncol. 2012; 30:419–425. PMID: 22203767.
10. Narita Y, Sato S, Kayama T. Review of the diagnosis and treatment of brain metastases. Jpn J Clin Oncol. 2022; 52:3–7. PMID: 34865060.
11. Valiente M, Ahluwalia MS, Boire A, Brastianos PK, Goldberg SB, Lee EQ, et al. The evolving landscape of brain metastasis. Trends Cancer. 2018; 4:176–196. PMID: 29506669.
12. Hatiboglu MA, Akdur K, Sawaya R. Neurosurgical management of patients with brain metastasis. Neurosurg Rev. 2020; 43:483–495. PMID: 30058049.
13. Gaspar L, Scott C, Rotman M, Asbell S, Phillips T, Wasserman T, et al. Recursive partitioning analysis (RPA) of prognostic factors in three Radiation Therapy Oncology Group (RTOG) brain metastases trials. Int J Radiat Oncol Biol Phys. 1997; 37:745–751. PMID: 9128946.
14. Sperduto PW, Kased N, Roberge D, Xu Z, Shanley R, Luo X, et al. Effect of tumor subtype on survival and the graded prognostic assessment for patients with breast cancer and brain metastases. Int J Radiat Oncol Biol Phys. 2012; 82:2111–2117. PMID: 21497451.
15. Sperduto PW, Yang TJ, Beal K, Pan H, Brown PD, Bangdiwala A, et al. Estimating survival in patients with lung cancer and brain metastases: an update of the graded prognostic assessment for lung cancer using molecular markers (Lung-molGPA). JAMA Oncol. 2017; 3:827–831. PMID: 27892978.
16. Sperduto PW, Jiang W, Brown PD, Braunstein S, Sneed P, Wattson DA, et al. Estimating survival in melanoma patients with brain metastases: an update of the graded prognostic assessment for melanoma using molecular markers (Melanoma-molGPA). Int J Radiat Oncol Biol Phys. 2017; 99:812–816. PMID: 29063850.
17. Sperduto PW, Deegan BJ, Li J, Jethwa KR, Brown PD, Lockney N, et al. Estimating survival for renal cell carcinoma patients with brain metastases: an update of the Renal Graded Prognostic Assessment tool. Neuro Oncol. 2018; 20:1652–1660. PMID: 30418657.
18. Sperduto PW, Fang P, Li J, Breen W, Brown PD, Cagney D, et al. Estimating survival in patients with gastrointestinal cancers and brain metastases: an update of the graded prognostic assessment for gastrointestinal cancers (GI-GPA). Clin Transl Radiat Oncol. 2019; 18:39–45. PMID: 31341974.
19. To KKW, Fong W, Cho WCS. Immunotherapy in treating EGFR-mutant lung cancer: current challenges and new strategies. Front Oncol. 2021; 11:635007. PMID: 34113560.
20. Dimitriou F, Long GV, Menzies AM. Novel adjuvant options for cutaneous melanoma. Ann Oncol. 2021; 32:854–865. PMID: 33771664.
21. Carapella CM, Gorgoglione N, Oppido PA. The role of surgical resection in patients with brain metastases. Curr Opin Oncol. 2018; 30:390–395. PMID: 30142093.
22. Hatiboglu MA, Chang EL, Suki D, Sawaya R, Wildrick DM, Weinberg JS. Outcomes and prognostic factors for patients with brainstem metastases undergoing stereotactic radiosurgery. Neurosurgery. 2011; 69:796–806. PMID: 21508879.
23. Li B, Yu J, Suntharalingam M, Kennedy AS, Amin PP, Chen Z, et al. Comparison of three treatment options for single brain metastasis from lung cancer. Int J Cancer. 2000; 90:37–45. PMID: 10725856.
24. Crossen JR, Garwood D, Glatstein E, Neuwelt EA. Neurobehavioral sequelae of cranial irradiation in adults: a review of radiation-induced encephalopathy. J Clin Oncol. 1994; 12:627–642. PMID: 8120563.
25. Meyers CA, Brown PD. Role and relevance of neurocognitive assessment in clinical trials of patients with CNS tumors. J Clin Oncol. 2006; 24:1305–1309. PMID: 16525186.
26. Tallet AV, Azria D, Barlesi F, Spano JP, Carpentier AF, Gonçalves A, et al. Neurocognitive function impairment after whole brain radiotherapy for brain metastases: actual assessment. Radiat Oncol. 2012; 7:77. PMID: 22640600.
27. Gondi V, Deshmukh S, Brown PD, Wefel JS, Tome W, Armstrong T, et al. NRG Oncology CC001: A phase III trial of hippocampal avoidance (HA) in addition to whole-brain radiotherapy (WBRT) plus memantine to preserve neurocognitive function (NCF) in patients with brain metastases (BM) [abstract]. J Clin Oncol. 2019; 37(15 Suppl):2009.
28. Brown PD, Gondi V, Pugh S, Tome WA, Wefel JS, Armstrong TS, et al. Hippocampal avoidance during whole-brain radiotherapy plus memantine for patients with brain metastases: phase III trial NRG oncology CC001. J Clin Oncol. 2020; 38:1019–1029. PMID: 32058845.
29. Costabile JD, Alaswad E, D’Souza S, Thompson JA, Ormond DR. Current applications of diffusion tensor imaging and tractography in intracranial tumor resection. Front Oncol. 2019; 9:426. PMID: 31192130.
30. Hervey-Jumper SL, Berger MS. Insular glioma surgery: an evolution of thought and practice. J Neurosurg. 2019; 130:9–16. PMID: 30611160.
31. Nimsky C, Ganslandt O, Hastreiter P, Fahlbusch R. Intraoperative compensation for brain shift. Surg Neurol. 2001; 56:357–364. discussion 364-5. PMID: 11755962.
32. Catapano G, Sgulò FG, Acurio Padilla PE, Spennato P, Di Nuzzo G, Boniello V, et al. Palatal position of patient tracker for magnetic neuronavigation system: technical note. World Neurosurg. 2018; 116:105–109. PMID: 29753080.
33. Krieg SM, Schäffner M, Shiban E, Droese D, Obermüller T, Gempt J, et al. Reliability of intraoperative neurophysiological monitoring using motor evoked potentials during resection of metastases in motor-eloquent brain regions: clinical article. J Neurosurg. 2013; 118:1269–1278. PMID: 23521547.
34. Zuo F, Hu K, Kong J, Zhang Y, Wan J. Surgical management of brain metastases in the perirolandic region. Front Oncol. 2020; 10:572644. PMID: 33194673.
35. Gutzwiller EM, Cabrilo I, Radovanovic I, Schaller K, Boëx C. Intraoperative monitoring with visual evoked potentials for brain surgeries. J Neurosurg. 2019; 130:654–660.
36. Zileli M, Idiman F, Hiçdönmez T, Ovül I, Tunçbay E. A comparative study of brain-stem auditory evoked potentials and blink reflexes in posterior fossa tumor patients. J Neurosurg. 1988; 69:660–668. PMID: 3183728.
37. Sayyahmelli S, Aydin I, Wheeler B, Baskaya MK. Mapping of the internal capsule with subcortical stimulation for gross-total resection of a thalamic metastatic tumor. Neurosurg Focus. 2018; 45(VideoSuppl2):V7. PMID: 30269558.
38. Han SJ, Morshed RA, Troncon I, Jordan KM, Henry RG, Hervey-Jumper SL, et al. Subcortical stimulation mapping of descending motor pathways for perirolandic gliomas: assessment of morbidity and functional outcome in 702 cases. J Neurosurg. 2018; 131:201–208. PMID: 30117770.
39. Duffau H, Peggy Gatignol ST, Mandonnet E, Capelle L, Taillandier L. Intraoperative subcortical stimulation mapping of language pathways in a consecutive series of 115 patients with Grade II glioma in the left dominant hemisphere. J Neurosurg. 2008; 109:461–471. PMID: 18759577.
40. Duffau H. Intraoperative direct subcortical stimulation for identification of the internal capsule, combined with an image-guided stereotactic system during surgery for basal ganglia lesions. Surg Neurol. 2000; 53:250–254. PMID: 10773257.
41. Raabe A, Beck J, Schucht P, Seidel K. Continuous dynamic mapping of the corticospinal tract during surgery of motor eloquent brain tumors: evaluation of a new method. J Neurosurg. 2014; 120:1015–1024. PMID: 24628613.
42. Yingling CD, Ojemann S, Dodson B, Harrington MJ, Berger MS. Identification of motor pathways during tumor surgery facilitated by multichannel electromyographic recording. J Neurosurg. 1999; 91:922–927. PMID: 10584836.
43. Nossek E, Korn A, Shahar T, Kanner AA, Yaffe H, Marcovici D, et al. Intraoperative mapping and monitoring of the corticospinal tracts with neurophysiological assessment and 3-dimensional ultrasonography-based navigation. J Neurosurg. 2011; 114:738–746. PMID: 20799862.
44. Shiban E, Krieg SM, Haller B, Buchmann N, Obermueller T, Boeckh-Behrens T, et al. Intraoperative subcortical motor evoked potential stimulation: how close is the corticospinal tract? J Neurosurg. 2015; 123:711–720. PMID: 26047412.
45. Okuda T, Kataoka K, Yabuuchi T, Yugami H, Kato A. Fluorescence-guided surgery of metastatic brain tumors using fluorescein sodium. J Clin Neurosci. 2010; 17:118–121. PMID: 19969462.
46. Hamamcıoğlu MK, Akçakaya MO, Göker B, Kasımcan MÖ, Kırış T. The use of the YELLOW 560 nm surgical microscope filter for sodium fluorescein-guided resection of brain tumors: our preliminary results in a series of 28 patients. Clin Neurol Neurosurg. 2016; 143:39–45. PMID: 26895208.
47. Marhold F, Mercea PA, Scheichel F, Berghoff AS, Heicappell P, Kiesel B, et al. Detailed analysis of 5-aminolevulinic acid induced fluorescence in different brain metastases at two specialized neurosurgical centers: experience in 157 cases. J Neurosurg. 2020; 133:1032–1043.
48. Kamp MA, Grosser P, Felsberg J, Slotty PJ, Steiger HJ, Reifenberger G, et al. 5-Aminolevulinic acid (5-ALA)-induced fluorescence in intracerebral metastases: a retrospective study. Acta Neurochir (Wien). 2012; 154:223–228. PMID: 22080159.
49. Baumert BG, Rutten I, Dehing-Oberije C, Twijnstra A, Dirx MJ, Debougnoux-Huppertz RM, et al. A pathology-based substrate for target definition in radiosurgery of brain metastases. Int J Radiat Oncol Biol Phys. 2006; 66:187–194. PMID: 16814946.
50. Neves S, Mazal PR, Wanschitz J, Rudnay AC, Drlicek M, Czech T, et al. Pseudogliomatous growth pattern of anaplastic small cell carcinomas metastatic to the brain. Clin Neuropathol. 2001; 20:38–42. PMID: 11220694.
51. Yoo H, Kim YZ, Nam BH, Shin SH, Yang HS, Lee JS, et al. Reduced local recurrence of a single brain metastasis through microscopic total resection. J Neurosurg. 2009; 110:730–736. PMID: 19072310.
52. Kamp MA, Dibué M, Niemann L, Reichelt DC, Felsberg J, Steiger HJ, et al. Proof of principle: supramarginal resection of cerebral metastases in eloquent brain areas. Acta Neurochir (Wien). 2012; 154:1981–1986. PMID: 22875595.
53. Patel P, Patel NV, Danish SF. Intracranial MR-guided laser-induced thermal therapy: single-center experience with the Visualase thermal therapy system. J Neurosurg. 2016; 125:853–860. PMID: 26722845.
54. Kamath AA, Friedman DD, Hacker CD, Smyth MD, Limbrick DD Jr, Kim AH, et al. MRI-guided interstitial laser ablation for intracranial lesions: a large single-institution experience of 133 cases. Stereotact Funct Neurosurg. 2017; 95:417–428. PMID: 29339639.
55. Missios S, Bekelis K, Barnett GH. Renaissance of laser interstitial thermal ablation. Neurosurg Focus. 2015; 38:E13.
56. Asher AL, Burri SH, Wiggins WF, Kelly RP, Boltes MO, Mehrlich M, et al. A new treatment paradigm: neoadjuvant radiosurgery before surgical resection of brain metastases with analysis of local tumor recurrence. Int J Radiat Oncol Biol Phys. 2014; 88:899–906. PMID: 24606851.
Fig. 1
Example of application of neuronavigation and diffusion tensor imaging (DTI) tractography. A: Neuronavigation showing the anatomical relationship between the tumor and the corticospinal tract (CST). B: Three-dimensional DTI tractography showing an intuitive perspective of the CST.
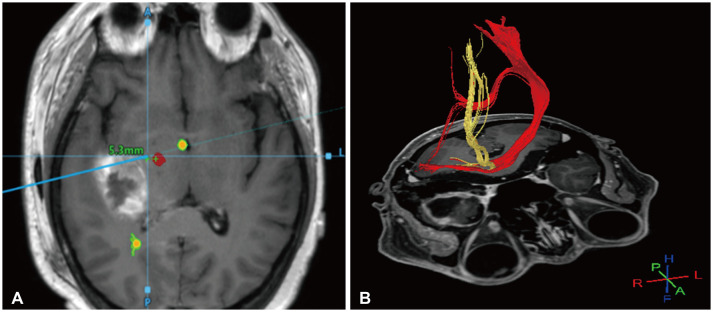
Fig. 2
Subcortical stimulation (SCS) during tumor resection. A: Sample monopolar stimulator for SCS. B: Application of SCS after tumor resection to estimate the distance to the corticospinal tract. C: Recording of muscle depolarization caused by SCS.
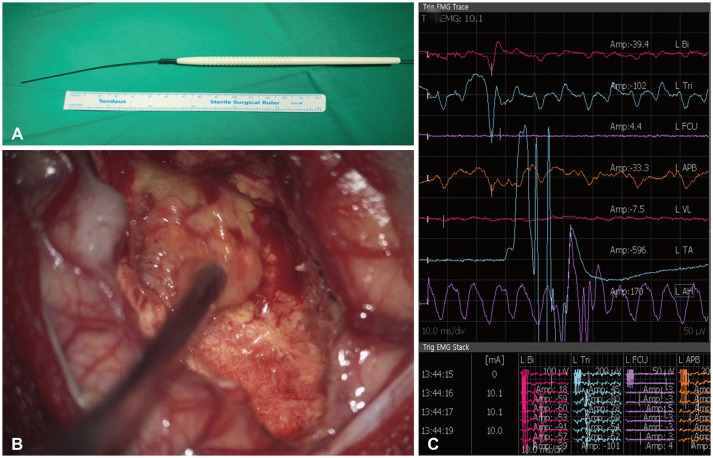
Fig. 3
Tumor resection using sodium fluorescein (A and B). A: Tumor and normal parenchyma under white light. B: Tumor and normal parenchyma under a yellow 560 nm filter. The tumor, where the blood-brain barrier was disrupted, is well stained by sodium fluorescein. Tumor resection using 5-aminolevulinic acid (5-ALA) (C and D). C: Tumor and normal parenchyma under white light. D: Tumor and normal parenchyma under a blue 400 nm filter. The tumor is well-stained with 5-ALA showing a strong red wavelength. CSF, cerebrospinal fluid.
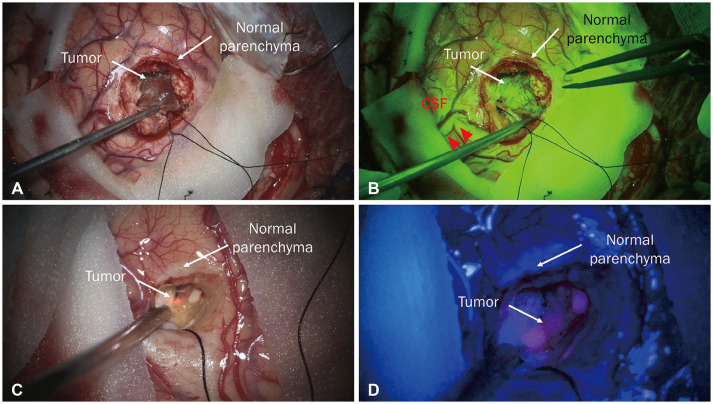
Table 1
Graded prognostic assessments by cancer type
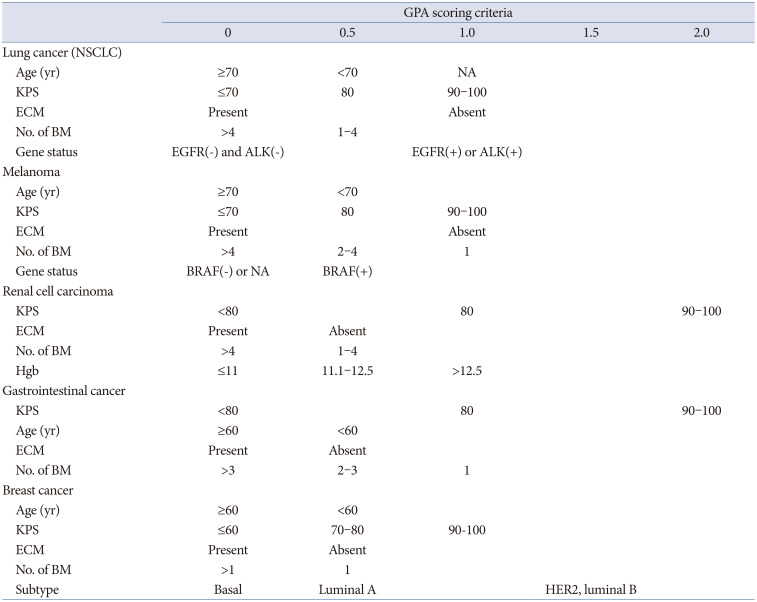