Abstract
Osteoporosis is a systemic skeletal disorder that causes vulnerability of bones to fracture owing to reduction in bone density and deterioration of the bone tissue microstructure. The prevalence of osteoporosis is higher in patients with autoimmune inflammatory rheumatic diseases, including rheumatoid arthritis (RA), than in those of the general population. In this autoimmune inflammatory rheumatic disease, in addition to known risk factors for osteoporosis, various factors such as chronic inflammation, autoantibodies, metabolic disorders, drugs, and decreased physical activity contribute to additional risk. In RA, disease-related inflammation plays an important role in local or systemic bone loss, and active treatment for inflammation can help prevent osteoporosis. In addition to conventional synthetic disease-modifying anti-rheumatic drugs that have been traditionally used for treatment of RA, biologic DMARDs and targeted synthetic DMARDs have been widely used. These agents can be employed more selectively and precisely based on disease pathogenesis. It has been reported that these drugs can inhibit bone loss by not only reducing inflammation in RA, but also by inhibiting bone resorption and promoting bone formation. In this review, the pathogenesis and research results of the increase in osteoporosis in RA are reviewed, and the effects of biological agents on osteoporosis are discussed.
Osteoporosis is a systemic skeletal disorder that causes vulnerability of bones to fracture due to reduction in bone density and degradation of the microstructure of bone tissues [1]. Osteoporosis is the most common metabolic bone disease and affects half of female and one-third of male in their sixties and seventies. Prevention and treatment of osteoporosis are critical because the increased prevalence of osteoporosis and fragility fractures, along with aging of the global population, result in significant clinical, economic, and social burdens [2-4].
Bones in the human body maintain a healthy state through a remodeling process. Bone remodeling is a physiological process of maintaining the optimal bone state by removing aged and damaged bone cells and generating new ones. In this process, the receptor activator of NF-κB (RANK), receptor activator of NF-κB ligand (RANKL), and osteoprotegerin (OPG) pathways are important for osteoclastogenesis. In addition, the Wnt signaling pathway has an important role in osteoblastogenesis. The above pathways as well as immune cells and cytokines are linked with bone cells and are regulated precisely. Osteoporosis is induced by an imbalance between bone formation and bone resorption in the bone remodeling process. The risk of osteoporosis and fragility fractures is higher in patients having autoimmune rheumatic diseases than in the general population. In autoimmune rheumatoid diseases, in addition to the traditional risk factors, various factors such as chronic inflammation, autoantibodies, decreased physical activity due to arthritis, drugs, and vitamin D deficiency can contribute to bone loss (Figure 1) [5,6].
Rheumatoid arthritis (RA) is a common systemic autoimmune disease with an unknown cause and is characterized by chronic, symmetric, and progressive inflammatory polyarthritis, in which bone loss can be caused by chronic inflammation [7,8]. Osteoporosis is more common in RA than in the general population. The prevalence rates of osteoporosis and osteoporotic fracture in both males and females are 2 and 1.5 times higher than those of the general population, respectively [9,10]. In RA patients, an increase in fracture frequency was observed in patients with higher bone mineral density (BMD) than in the general population, suggesting bone quality deterioration in RA patients [11]. Pro-inflammatory cytokines, such as interleukin (IL)-1β, IL-6, IL-17, and tumor necrosis factor (TNF)-α, which play important roles in the pathogenesis of RA, not only induce chronic inflammation, but also promote bone loss and fragility through increased RANKL expression. They have been found to foster bone loss through processes such as resorption imbalance. These findings suggest that modulating inflammation in RA may be important in suppressing bone loss [12-14]. In support of this, biologic disease-modifying anti-rheumatic drugs (bDMARD) and targeted synthetic DMARDs (tsDMARDs), which have been widely used for treatment of RA, not only modulate disease activity, but also inhibit local and systemic bone loss [15].
In this review, we summarize the effects of inflammatory and autoimmune responses that play important roles in RA pathogenesis, and we identify the key pathways, cells, and mediators involved in bone resorption and bone formation in osteoporosis. Since relatively few studies have investigated the effects of tsDMARD on bone, here we discuss whether biologics have a positive effect on osteoporosis in RA.
1) Cells and osteoporosis
Bone is a dynamic organ that continually changes and undergoes continuous remodeling throughout life. Several factors are involved in bone remodeling, among which the interactions between bone cells (osteoclasts, osteoblasts, and osteocytes) and immune cells (macrophages, monocytes, dendritic cells, T cells, and B cells) are very important (Table 1, Figure 2) [16-21].
(1) Bone cells (osteoclast–osteoblast–osteocyte)
The osteoclast is a multinuclear cell in the monocyte/macrophage line derived from the hematopoietic stem cell. It has an important function in maintenance and remodeling by decomposing organic and inorganic parts of bone tissue. In the presence of inflammation, the influx of osteoclast precursors and differentiation and activation into osteoclasts can be enhanced by pro-inflammatory cytokines, such as macrophage colony-stimulating factor (M-CSF), IL-1β, IL-6, IL-17, and TNF-α [22]. The RANKL-RANK-OPG system plays a critical role in osteoclast regulation.
Osteoblasts, which are derived from mesenchymal stem cells, are responsible for bone synthesis and mineralization through the processes of bone modeling and remodeling. In addition, these cells produce various cell products, such as alkaline phosphatase (ALP) and collagenase, growth factors, osteocalcin, and collagen. The Wnt pathway is crucial to the differentiation and function of osteoblasts [23].
Osteoclast activity is regulated by the interaction of OPG and RANKL produced by osteoblasts [24]. RANKL is a key molecule involved in the regulation of osteoclast differentiation and is mainly produced by osteoblasts and osteocytes. In addition, RANKL can be generated from synovial fibroblasts and activated B cells and T cells (especially Th17 cells) under inflammation [25,26]. RANKL binds to RANK in osteoclast precursors and mature osteoclast to induce differentiation and activation. This process can be inhibited by OPG, which acts as a soluble decoy receptor for RANKL. Pro-inflammatory cytokines, such as TNF-α, which are increased in inflammatory rheumatic diseases, inhibit Wnt-induced osteoblastogenesis through increased expression of dickkopf-1 (DKK1), a Wnt pathway inhibitor [27-29], while promoting osteoclastogenesis by increasing RANKL expression.
Osteocytes compose 90%~95% of all bone cells of the adult skeleton, and their average half-life is approximately 25 years [30]. Osteocytes are derived from mesenchymal osteoprogenitor cells and osteoblasts. They are located in a space called the lacunae within a mineralized matrix. Osteocytes form networks with tiny canals called caniculi. Through this network, nutrients, excreta, and other materials are exchanged. In addition, it plays important roles as a sensor of mechanical load, regulator of bone remodeling through control of phosphate homeostasis and endocrine cell secretion of various factors, and regulator of osteoblast and osteoclast activity [31].
(2) T cells
The naive cluster of differentiation 4+ (CD4+) T cell is differentiated into Th1 (T helper 1), Th2, Th9, Th17, Th22, Treg (regulator T), and Tfh (follicular helper T) cells by various micro-environmental and cytokine stimuli. Each performs its own function [32]. The T cells that are critical in RA pathogenesis are Th1, Th2, Th17, and Treg. Their effects are outlined below.
Th1 cells: Naive CD4+ T cells differentiate into Th1 cells when stimulated by IL-12 to produce interferon gamma (IFN-γ), IL-2, lymphotoxin, TNF-α, and granulocyte-macrophage CSF (GM-CSF) [33]. Through this process, they generally induce cell-mediated immune responses by macrophage and cytotoxic T cells against intracellular bacteria and protozoa [34]. Th1 cells have also been implicated in the pathogenesis of various autoimmune and inflammatory diseases [34-36]. In bone metabolism, Th1 cells may inhibit osteoclastogenesis. IFN-γ, a Th1 cytokine, can reduce bone loss by inhibiting osteoclast formation through degradation of TNF Receptor Associated Factor 6 (TRAF6) molecules.
Th2 cells: The Th2 cells induce humoral immune responses and play an important role in host defense against parasites and allergic and atopic diseases [37]. These cells mainly regulate eosinophils, basophils, and mast cells. However, overactivation of Th2 cells can cause diseases such as type I hypersensitivity, allergic rhinitis, atopic dermatitis, and asthma [38]. IL-4 and IL-13, which are important cytokines of Th2 cells, are associated with inhibition of osteoclastogenesis via decreased RANKL and RANK and increased OPG [39]. Th2 cells maintain osteoblast function under various inflammatory conditions through increased parathyroid hormone (PTH) production and inhibit bone loss by decreasing the RANKL/OPG ratio [40]. Therefore, Th2 cells have an osteoprotective function in the pathophysiology of osteoporosis.
Th17 cells: When naive CD4+ T cells are stimulated by IL-1β, IL-6, IL-23, and transforming growth factor-β (TGF-β), they differentiate into the Th17 cells IL-17, IL-21, IL-22. GM-CSF is the main effector cytokine [41,42]. Th17 cells protect the body from bacterial infection and induce various immune responses through generation and recruitment of neutrophils [43,44]. In addition, Th17 cells play an important role in various inflammatory diseases, including osteoporosis, psoriasis, periodontal disease, RA, and inflammatory bowel diseases [45,46]. Th17 cells are involved in osteoclastogenesis through various mechanisms. Secretion of IL-17 promotes RANKL production in fibroblasts and osteoblasts, stimulates M-CSF and RANKL production by osteoblasts and stromal cells, and increases RANK expression in osteoclast precursors [47,48]. Although IL-17 appears to have a key function in the pathogenesis of RA, treatment with IL-17 inhibitors does not achieve the primary endpoint of American College of Rheumatology 20 (ACR20), unlike in psoriasis, psoriatic arthritis, and ankylosing spondylitis [48]. In this regard, further research is needed.
Treg cells: Treg cells (CD4+CD25+Foxp3+ T cells) play an important role in controlling and preventing various inflammatory and autoimmune diseases by inhibiting activation of the immune system [49]. Treg cells regulate inflammation and immune responses by secreting anti-inflammatory cytokines, such as IL-10 and TGF-β [50,51]. They directly inhibit osteoclasts by suppression of RANKL and M-CSF production [4]. They are also known to promote activation of osteoblasts [52,53].
In summary, it is assumed that the balance among Th1, Th2, and Treg cells, which suppress osteoclastogenesis, and Th17 cells, which promote osteoclastogenesis, is critical in the pathogenesis of osteoporosis. Meanwhile, in inflammatory conditions such as RA, depending on the environment, Th1 cells can act differently.
(3) B cells
The B lymphocyte is responsible for humoral immunity among adaptive immunities. When naive B cells are activated by antigens, they differentiate into plasma cells and produce antibodies to show an immune response. Under physiological conditions, B cells produce approximately 40%~60% of OPG [54]. However, B cells activated by inflammation increase osteoclast precursors through granulocyte CSF (G-CSF) secretion [55], and they activate osteoclast formation by producing RANKL. In addition, B cells can stimulate osteoclasts through production of immune complexes and autoantibodies [56-58]. In conclusion, activated B cells in inflammatory conditions contribute to osteoclastogenesis by secreting G-CSF and RANKL while contributing to antibody production.
(4) Innate immune cells
Macrophages are inflammatory cells that contribute to the host defense system by inducing phagocytosis and inflammatory responses against pathogen infection. Macrophages act as precursors to osteoclasts and induce bone loss by producing pro-inflammatory cytokines, such as IL-1β, IL-6, and TNF-α to promote osteoclast formation [59]. They also play an important role in the pathogenesis of RA [60].
Monocytes migrate from blood vessels to inflammatory sites in a C-C chemokine receptor type 2 (CCR2)-dependent manner and differentiate into macrophages or dendritic cells, contributing to inflammatory and repair processes [61,62]. Meanwhile, monocytes act as osteoclast precursors and can be involved in inflammatory cell recruitment and osteoclastogenesis through production of cytokines and chemokines [63,64].
Neutrophils are involved in the pathogenesis of various diseases, including inflammation-mediated bone loss, through cytokine and chemokine production [65-67]. Neutrophils can also differentiate into osteoclast precursors when activated. In addition, neutrophils express membrane-bound RANKL (mRANKL) and RANK and may contribute to osteoclastogenesis by acting on osteoclasts and their precursors [67,68].
Several other innate immune cells may also be directly/indirectly involved in osteoclastogenesis [20].
In summary, some innate immune cells can be differentiated into osteoclasts by serving as osteoclast precursors in the microenvironment of RANKL, M-CSF, and pro-inflammatory cytokines. These cells can also contribute to osteoclastogenesis by directly producing pro-inflammatory cytokines.
2) Cytokines and osteoporosis
TNF-α plays an important role in the pathogenesis of osteoporosis as well as in inflammatory diseases such as RA [69-72]. TNF-α increases RANKL expression in T cells, B cells, and osteoblasts, and it increases RANK expression in osteoclast precursors. Eventually, it promotes differentiation into osteoclasts and causes bone resorption [73]. Meanwhile, TNF-α inhibits osteoblast differentiation by increasing DKK1 and sclerostin expression to reduce bone formation [74,75]. As a result, TNF-α exhibits an overall osteoclastogenic effect.
IFN-γ exhibits a dual effect on bone. It can inhibit osteoclast formation by inducing degradation of the RANK adapter protein TRAF6, delaying osteoclast formation by acting directly on osteoclast precursors, and promoting osteoclast apoptosis by inducing superoxide production. On the other hand, IFN-γ acts as an inducer of major histocompatibility complex (MHC) class II expression and antigen presentation, and it can promote osteoclastogenesis by activating T cells and promoting RANKL and TNF-α secretion from T cells. It contributes to the formation of functional multinuclear osteoclasts by fusion of osteoclasts [76]. The mechanism of these conflicting reactions is not clear; nevertheless, IFN-γ is presumed to exhibit pro- or anti-resorptive activity depending on the state of estrogen or inflammation in the body.
IL-1β promotes osteoclastogenesis by increasing RANKL production in osteoblasts [77], and it inhibits osteoclast apoptosis through M-CSF production [78]. It also promotes RANKL-dependent osteoclast differentiation through activation of NF-κB and AP-1, while contributing to osteoclast migration and activation through increased C-C chemokine receptor type 7 (CCR7) [79]. In addition, it is possible to reduce osteoblast activity by inhibiting the production of ALP [77].
IL-6 can directly stimulate bone loss in RA and promotes bone resorption through increased expression of RANKL in osteoblasts, fibroblasts, and T cells. In addition, it can indirectly contribute to bone loss by stimulating the differentiation of Th17 cells [80,81]. IL-6 plays an important mediator role in the inhibition of osteoblast differentiation by TNF-α, inhibiting the Wnt pathway [82].
IL-17 is involved in RANKL-RANK-OPG pathway-dependent osteoclastogenesis. By increasing RANK expression in osteoclast precursors, it increases the sensitivity of osteoclast precursors to RANKL [83]. This encourages osteoclastogenesis by increasing RANKL expression in various cells, such as osteoblasts, synovial cells, and mesenchymal cells, and by increasing the RANKL/OPG ratio [84]. IL-17 also indirectly contributes to bone loss by inducing the expression and secretion of pro-inflammatory cytokines such as IL-6, G-CSF, GM-CSF, and TNF-α from other cells [85,86]. It has been reported that IL-17, unlike TNF-α, can promote the Wnt pathway by inhibiting DKK1 [87,88]. This may explain some of the differences between the TNF inhibitor and IL-17 inhibitor for new bone formation in spondyloarthritis.
Under the inflammatory state of RA, pro-inflammatory cytokines such as IL-1β, IL-6, IL-17, and TNF-α are increased. An increase of pro-inflammatory cytokines induces promotion of osteoclastogenesis and inhibition of osteoblastogenesis through interaction with immune cells and bone cells, contributing to local and systemic bone loss in RA (Table 2, Figure 2) [25,89].
3) Autoantibodies and osteoporosis
Autoantibodies such as rheumatoid factor (RF) or anti-citrullinated protein antibodies (ACPA) in RA are related to extra-articular involvement and poor prognosis. Their importance in osteoporosis has been highlighted in several studies. In an experimental study, citrullinated vimentin, which is a target antigen of ACPA, was expressed in monocyte/macrophage line cells and osteoclast precursors. When ACPA was bound, differentiation into osteoclast occurred [90]. In addition, IL-8 production is increased in ACPA-stimulated osteoclasts, and circulating ACPA promotes osteoclastogenesis through an IL-8-dependent autocrine loop [91]. ACPA can directly/indirectly activate osteoclasts through several mechanisms such as stimulation of TNF-α production and synovial fibroblast migration [91,92].
Autoantibody-positive cases in asymptomatic healthy individuals increase the future risk of RA in these individuals [90,93]. In a preclinical stage, an ACPA-positive group had decreased cortical bone thickness and increased porosity compared to the negative control group, as assessed by high-resolution peripheral quantitative computed tomography [94].
In addition, in a study of 155 treatment-naive patients with early-stage RA, decreased systemic BMD was observed in association with ACPA positivity and high RF level [95]. These results suggest that autoantibodies can act directly on bone. Moreover, seropositive RA patients have more severe bone erosion and osteopenia than seronegative RA patients [96-100]. A recent study also found that ACPA-positive RA patients had a higher 10-year risk of major hip fracture at fracture risk assessment tool (FRAX), and they had a lower BMD of the femoral neck than ACPA-negative RA patients [101].
Autoantibodies in RA have been reported to be associated with osteoporosis not only in experimental studies, but also in clinical studies. In particular, association with decreased BMD was reported in the preclinical period of RA without inflammation, suggesting that autoantibodies directly affect bone metabolism (Figure 2).
4) Increased imbalance between bone resorption and bone formation in RA
The RANKL-RANK-OPG pathway plays an important role in bone loss by promoting the differentiation of osteoclast precursors to osteoclasts, whereas the Wnt signaling pathway plays an important role in bone formation through osteoblast maturation. In normal bone remodeling, these pathways are properly balanced through precise coordination with cells and cytokines. However, when this balance is disrupted by various causes, osteoporosis can occur [102,103].
In RA patients, compared with the control group, the serum RANKL level was higher in the patient group, and the OPG level and OPG/RANKL ratio were significantly higher in the control group. This suggests that osteoclastogenesis is further activated through the RANKL-RANK-OPG pathway in RA patients [10]. DKK1 and sclerostin, which are inhibitors of the Wnt pathway, are stimulated in RA by inflammatory cytokines such as TNF-α, which ultimately inhibits bone formation [74,104]. Elevation of DKK1 was observed in the synovial membrane and serum of RA patients and was found to increase the risk of bone erosion [105].
Increased pro-inflammatory cytokines, such as IL-1β, IL-6, and TNF-α, in the inflammatory state of RA contribute to the imbalance of bone formation and bone resorption through activation of the RANKL/RANK/OPG pathway and inhibition of the Wnt pathway. Immune cells, such as macrophages, synovial fibroblasts, T cells, and B cells, which play an important role in the inflammatory process, as well as autoantibodies such as RF and ACPA can also contribute to bone loss through this imbalance [106].
Most conventional synthetic DMARDs (csDMARDs) used in RA can be expected to show a positive effect on bone density and metabolism by reducing inflammation. Nonetheless, evidence of their effectiveness in preventing bone loss is lacking, and results in this area remain controversial. Methotrexate (MTX) showed a non-deleterious effect on BMD, and leflunomide was observed to significantly increase lumbar spine BMD [107,108].
On the other hand, it has been reported that most bDMARDs effectively inhibit osteoclast-mediated bone loss and exert a positive effect on osteoblasts, contributing to stabilization of bone metabolism [15,25,109].
1) TNF-α inhibitors
As mentioned above, TNF-α was found to play an important role in local and systemic bone loss by promoting bone resorption in RA patients.
TNF-α inhibitors are the primary biologics used for RA patients and include monoclonal antibody agents such as infliximab, adalimumab, golimumab, and certolizumab and soluble receptor agents such as etanercept. It has been reported that TNF-α inhibitors have superior effects to csDMARDs in controlling disease activity and inflammation and prevention of joint damage. In animal models, TNF-α inhibition has been shown to improve the negative balance of bone turnover, decrease osteoclasts, and increase osteoblasts [110,111].
Serum osteocalcin, a marker of bone formation, was shown to be lower in premenopausal female RA patients than in the control group, while N-telopeptide and deoxypyridinoline, markers of bone resorption, were high. Changes in these bone markers were correlated with disease activity [112]. It was reported that TNF-α inhibitors increased bone formation markers and reduced bone resorption markers in several clinical studies (Table 3) [113-116]. In addition, TNF-α inhibitors promoted osteoblastogenesis by significantly reducing DKK1, and they inhibited osteoclastogenesis by increasing OPG, decreasing RANKL, and decreasing the RANKL/OPG ratio (Table 3) [105,117-121].
In additional exploratory analyses of the two-year premier study in RA patients, hand bone loss was evaluated with digital X-ray radiogrammetry. The results showed that adalimumab could reduce metacarpal cortical bone loss [122]. In a cohort study of 184 RA patients receiving adalimumab, after one year of treatment, the BMD of the hip and lumbar spine remained stable, while the BMD of the hand decreased by 1.41% (p<0.0001) [123]. This suggests that TNF-α inhibitors are effective in blocking systemic bone loss; however, they may have limitations in inhibiting local bone loss. In a case-control study comparing RA patients treated with infliximab and RA patients treated with MTX, a significant decrease in spinal and femoral neck BMD was observed in the control group; however, both spine and femoral neck BMD were preserved in the infliximab-treated group. The protective effect of infliximab on bone was observed regardless of disease activity [124]. Other studies using TNF-α inhibitors showed similar results [125,126].
2) Abatacept
Abatacept (a cytotoxic T-lymphocyte-associated protein 4 [CTLA4]–immunoglobulin fusion protein) inhibits T cell activation and differentiation by blocking signal transmission between T cells and antigen presentation cells by binding with CD80 or CD86. It plays an important role in pathogenesis-based treatment of RA [127,128].
In an experimental study on murine peripheral blood mononuclear cells, binding of CTLA4 to osteoclast precursor cells directly inhibited osteoclast differentiation and maturation [129]. In CD80/86-deficient mice, increased osteoclast differentiation induced osteopenia, suggesting that CD80/86-targeting abatacept may inhibit osteoclastogenesis [130]. Moreover, it was reported that abatacept directly suppresses osteoclastogenesis by interfering with intracellular calcium oscillations in bone marrow macrophages [131], and it promotes osteoblastogenesis through increased production of Wnt protein in T cells [132,133]. Meanwhile, in a mouse model, abatacept inhibited PTH-induced bone loss [134]. In a clinical study, abatacept significantly reduced serum RANKL [135]. In addition, abatacept significantly elevated serum OPG and significantly decreased serum DKK1 [136]. These results suggest that abatacept may be involved in both inhibition of osteoclastogenesis and promotion of osteoblastogenesis (Table 3).
In the AIRTIGHT study, a prospective, comparative, and non-randomized study, the effects of abatacept and other bDMARDs on bone metabolism were investigated. Abatacept treatment was significantly associated with an increase in femoral neck BMD. The efficacy of abatacept on the increase of femoral neck BMD was superior to that of other bDMARDs. Although the mechanism by which abatacept increases BMD in the femoral neck remains unclear, CTLA4 immunoglobulin is known to promote Wnt-10b production and bone formation [137]. In an interim analysis of a three-year longitudinal cohort study, the protective effects of various biologics and csDMARDs on systemic bone loss were investigated. Compared with csDMARDs and TNF-α inhibitors, abatacept showed a better BMD preservation effect in RA [138]. These differences suggest that T cells may play a key role in the pathogenesis of RA-associated osteoporosis. Autoantibodies are also crucial in the pathogenesis of osteoporosis. Abatacept can inhibit the production of autoantibodies by inhibiting the differentiation of T cell-dependent B cells into plasma cells. On the other hand, bDMARDs targeting pro-inflammatory cytokines were found to have a smaller effect on ACPA level [139,140]. The ability of abatacept to inhibit autoantibody production may also have induced differences between these drugs.
3) Rituximab
Rituximab is a monoclonal antibody against the molecule CD20 on the surface of B cells. Since the CD20 molecule is involved in complement activation, rituximab induces B cell apoptosis through complement-mediated cytotoxicity after binding to CD20. Currently, it is being used in clinical settings with approval as a secondary biologic treatment for RA patients [141,142]. In an RA mouse model, B cells inhibit bone formation through suppression of osteoblast differentiation and functions [143].
In a study analyzing the cytokine mRNA profile of inflammatory cells isolated from the synovial fluid of 12 RA patients, high expression of mRNA for RANKL was observed in B cells. This finding suggests that B cells are the main source of RANKL [144]. In addition, anti-CD20-mediated B cell depletion was related to increased bone mass in a mouse model [145]. In a study using the synovial membranes of 28 RA patients treated with rituximab, RANK-positive osteoclast precursors and synovial RANKL expression were significantly reduced. In serum, OPG and RANKL were significantly decreased, and the OPG/RANKL ratio was significantly increased. These results partially explain the protective effect of rituximab on the progression of bone loss in RA [146].
In an exploratory and prospective study examining changes in bone density and bone turnover in RA patients treated with rituximab, lumbar spine and forearm BMD were effectively maintained, whereas femur BMD was significantly decreased. Furthermore, significant elevations of procollagen type 1 amino-terminal propeptide (P1NP), and bone-specific ALP, all biomarkers of bone formation, were observed (Table 3) [147].
4) IL-6 inhibitor
Blockade of IL-6 receptors directly inhibited osteoclast formation in vitro and in vivo, and this effect was observed regardless of the anti-inflammatory effect of anti-IL-6R therapy [148]. Tocilizumab is a monoclonal antibody that binds to IL-6 receptor, a pro-inflammatory cytokine, and inhibits inflammation-related IL-6 signaling [149]. In a one-year prospective open study, RA patients treated with tocilizumab showed a decrease in serum DKK1 concentration and an increase in serum P1NP, an osteogenic marker, without significant change in BMD [150]. In the radiate study, which was a randomized, double-blind, placebo-controlled, parallel-group phase 3 trial, the effects of tocilizumab on bone metabolism markers in 299 anti-TNF refractory RA patients were investigated. In that study, the tocilizumab group showed significantly reduced C-terminal telopeptides of type 1 collagen (CTX), CTX/osteocalcin ratio, and matrix metalloproteinase 3 compared to the MTX group [151]. A study comparing changes in bone marrow tissue obtained from 10 RA patients treated with tocilizumab and 10 other RA patients treated with MTX monotherapy demonstrated a significant increase in OPG expression in tocilizumab-treated patients compared with the control [152]. In a preliminary study comparing the effects of tocilizumab on bone homeostasis in 22 patients with active RA and 22 healthy patients, the serum level of DKK1 was decreased in the tocilizumab group compared with baseline after two months of treatment. The ratio of OPG/RANKL was significantly increased. These results suggest that the positive effect of tocilizumab on bone remodeling was induced by reduction of the effect of DKK1 on the Wnt pathway and rapid and effective inhibition of inflammation [153].
In a multicenter single-arm study, tocilizumab maintained stable BMD for two years [154]. Another study showed a significant increase of lumbar and femoral neck BMD in patients with osteopenia at baseline [155]. In ACPA-positive RA patients, two years of tocilizumab treatment significantly decreased CTX level and significantly increased femoral neck BMD [156]. In a retrospective study investigating bone metabolism and BMD when denosumab was used in combination with anti-TNF inhibitors, tocilizumab, or abatacept in osteoporotic patients with RA, tocilizumab was more beneficial than the other drugs in increasing BMD in the hip joint [157].
In summary, biologics used for RA induce positive changes in the bone turnover marker profile and show good results in improving BMD (Table 3). Theoretically, it is estimated that abatacept, which is more effective in reducing autoantibody production along with T cell inhibition, would be more advantageous in inhibiting bone loss. However, there does not appear to be a clear difference between the drugs. Abatacept and tocilizumab have been reported to improve BMD better than other biologics in some studies [137,157]; further research is needed in this regard.
In this review, we investigated the pathogenesis of osteoporosis associated with RA and the effects of biologics on osteoporosis. In addition to the traditional risk factors for osteoporosis, RA increases the risk of osteoporosis compared to the general population owing to several additional factors, such as chronic inflammation, autoantibodies, drugs, and decreased physical activity. In particular, in RA, immune cells, pro-inflammatory cytokines, and autoantibodies related to autoimmune reactions interact with RANKL-RANK-OPG and Wnt pathways to promote bone resorption and inhibit bone formation. Through these mechanisms, bone loss can be further aggravated in RA. Under this consideration, control of inflammation using biologics in RA may not only inhibit disease progression and improve the quality of life, but also may be important in preventing and treating osteoporosis. In agreement, several studies have reported positive results of biologics in improving BMD with changes in the bone turnover marker profile. However, the effects of these treatments on bone remain largely unknown. Further studies should be conducted on the pathophysiology of osteoporosis associated with RA and the clinical effects of various treatments including biologics.
REFERENCES
1. NIH Consensus Development Panel on Osteoporosis Prevention. Diagnosis. and Therapy. 2001; Osteoporosis prevention, diagnosis, and therapy. JAMA. 285:785–95. DOI: 10.1001/jama.285.6.785. PMID: 11176917.
2. Melton LJ 3rd. 2001; The prevalence of osteoporosis: gender and racial comparison. Calcif Tissue Int. 69:179–81. DOI: 10.1007/s00223-001-1043-9. PMID: 11730244.
3. Wright NC, Looker AC, Saag KG, Curtis JR, Delzell ES, Randall S, et al. 2014; The recent prevalence of osteoporosis and low bone mass in the United States based on bone mineral density at the femoral neck or lumbar spine. J Bone Miner Res. 29:2520–6. DOI: 10.1002/jbmr.2269. PMID: 24771492. PMCID: PMC4757905.
4. Wade SW, Strader C, Fitzpatrick LA, Anthony MS, O'Malley CD. 2014; Estimating prevalence of osteoporosis: examples from industrialized countries. Arch Osteoporos. 9:182. DOI: 10.1007/s11657-014-0182-3. PMID: 24847682.
5. Keller JJ, Kang JH, Lin HC. 2013; Association between osteoporosis and psoriasis: results from the Longitudinal Health Insurance Database in Taiwan. Osteoporos Int. 24:1835–41. DOI: 10.1007/s00198-012-2185-5. PMID: 23052942.
6. Oo WM, Naganathan V, Bo MT, Hunter DJ. 2018; Clinical utilities of quantitative ultrasound in osteoporosis associated with inflammatory rheumatic diseases. Quant Imaging Med Surg. 8:100–13. DOI: 10.21037/qims.2018.02.02. PMID: 29541626. PMCID: PMC5835660.
7. McInnes IB, Schett G. 2011; The pathogenesis of rheumatoid arthritis. N Engl J Med. 365:2205–19. DOI: 10.1056/NEJMra1004965. PMID: 22150039.
8. Firestein GS. 2003; Evolving concepts of rheumatoid arthritis. Nature. 423:356–61. DOI: 10.1038/nature01661. PMID: 12748655.
9. Haugeberg G, Uhlig T, Falch JA, Halse JI, Kvien TK. 2000; Bone mineral density and frequency of osteoporosis in female patients with rheumatoid arthritis: results from 394 patients in the Oslo County Rheumatoid Arthritis register. Arthritis Rheum. 43:522–30. DOI: 10.1002/1529-0131(200003)43:3<522::AID-ANR7>3.0.CO;2-Y. PMID: 10728744.
10. Xu S, Wang Y, Lu J, Xu J. 2012; Osteoprotegerin and RANKL in the pathogenesis of rheumatoid arthritis-induced osteoporosis. Rheumatol Int. 32:3397–403. DOI: 10.1007/s00296-011-2175-5. PMID: 22057136.
11. Raterman HG, Lems WF. 2019; Pharmacological management of osteoporosis in rheumatoid arthritis patients: a review of the literature and practical guide. Drugs Aging. 36:1061–72. DOI: 10.1007/s40266-019-00714-4. PMID: 31541358. PMCID: PMC6884430.
12. Schett G. 2009; Osteoimmunology in rheumatic diseases. Arthritis Res Ther. 11:210. DOI: 10.1186/ar2571. PMID: 19232069. PMCID: PMC2688223.
13. Schett G, Saag KG, Bijlsma JW. 2010; From bone biology to clinical outcome: state of the art and future perspectives. Ann Rheum Dis. 69:1415–9. DOI: 10.1136/ard.2010.135061. PMID: 20650876.
14. Geusens P, Lems WF. 2011; Osteoimmunology and osteoporosis. Arthritis Res Ther. 13:242. DOI: 10.1186/ar3375. PMID: 21996023. PMCID: PMC3308061.
15. Soós B, Szentpétery Á, Raterman HG, Lems WF, Bhattoa HP, Szekanecz Z. 2022; Effects of targeted therapies on bone in rheumatic and musculoskeletal diseases. Nat Rev Rheumatol. 18:249–57. DOI: 10.1038/s41584-022-00764-w. PMID: 35273387.
16. Fischer V, Haffner-Luntzer M. 2022; Interaction between bone and immune cells: implications for postmenopausal osteoporosis. Semin Cell Dev Biol. 123:14–21. DOI: 10.1016/j.semcdb.2021.05.014. PMID: 34024716.
17. Takayanagi H. 2009; Osteoimmunology and the effects of the immune system on bone. Nat Rev Rheumatol. 5:667–76. DOI: 10.1038/nrrheum.2009.217. PMID: 19884898.
18. Srivastava RK, Dar HY, Mishra PK. 2018; Immunoporosis: immunology of osteoporosis-role of T cells. Front Immunol. 9:657. DOI: 10.3389/fimmu.2018.00657. PMID: 29675022. PMCID: PMC5895643.
19. Raphael I, Nalawade S, Eagar TN, Forsthuber TG. 2015; T cell subsets and their signature cytokines in autoimmune and inflammatory diseases. Cytokine. 74:5–17. DOI: 10.1016/j.cyto.2014.09.011. PMID: 25458968. PMCID: PMC4416069.
20. Saxena Y, Routh S, Mukhopadhaya A. 2021; Immunoporosis: role of innate immune cells in osteoporosis. Front Immunol. 12:687037. DOI: 10.3389/fimmu.2021.687037. PMID: 34421899. PMCID: PMC8374941.
21. Ming J, Cronin SJF, Penninger JM. 2020; Targeting the RANKL/RANK/OPG axis for cancer therapy. Front Oncol. 10:1283. DOI: 10.3389/fonc.2020.01283. PMID: 32850393. PMCID: PMC7426519.
22. Hodge JM, Collier FM, Pavlos NJ, Kirkland MA, Nicholson GC. 2011; M-CSF potently augments RANKL-induced resorption activation in mature human osteoclasts. PLoS One. 6:e21462. DOI: 10.1371/journal.pone.0021462. PMID: 21738673. PMCID: PMC3126821.
23. Chen X, Wang Z, Duan N, Zhu G, Schwarz EM, Xie C. 2018; Osteoblast-osteoclast interactions. Connect Tissue Res. 59:99–107. DOI: 10.1080/03008207.2017.1290085. PMID: 28324674. PMCID: PMC5612831.
24. Schoppet M, Preissner KT, Hofbauer LC. 2002; RANK ligand and osteoprotegerin: paracrine regulators of bone metabolism and vascular function. Arterioscler Thromb Vasc Biol. 22:549–53. DOI: 10.1161/01.ATV.0000012303.37971.DA. PMID: 11950689.
25. Zerbini CAF, Clark P, Mendez-Sanchez L, Pereira RMR, Messina OD, Uña CR, et al. 2017; Biologic therapies and bone loss in rheumatoid arthritis. Osteoporos Int. 28:429–46. DOI: 10.1007/s00198-016-3769-2. PMID: 27796445.
26. Ono T, Hayashi M, Sasaki F, Nakashima T. 2020; RANKL biology: bone metabolism, the immune system, and beyond. Inflamm Regen. 40:2. DOI: 10.1186/s41232-019-0111-3. PMID: 32047573. PMCID: PMC7006158.
27. Lam J, Takeshita S, Barker JE, Kanagawa O, Ross FP, Teitelbaum SL. 2000; TNF-alpha induces osteoclastogenesis by direct stimulation of macrophages exposed to permissive levels of RANK ligand. J Clin Invest. 106:1481–8. DOI: 10.1172/JCI11176. PMID: 11120755. PMCID: PMC387259.
28. Wang SY, Liu YY, Ye H, Guo JP, Li R, Liu X, et al. 2011; Circulating Dickkopf-1 is correlated with bone erosion and inflammation in rheumatoid arthritis. J Rheumatol. 38:821–7. DOI: 10.3899/jrheum.100089. PMID: 21362762.
29. Liu YY, Long L, Wang SY, Guo JP, Ye H, Cui LF, et al. 2010; Circulating Dickkopf-1 and osteoprotegerin in patients with early and longstanding rheumatoid arthritis. Chin Med J (Engl). 123:1407–12.
30. Bonewald LF. 2013; In: Rosen CJ, ed. Primer on the metabolic bone diseases and disorders of mineral metabolism. 8th ed. Ames. Wiley-Blackwell. 34–41. DOI: 10.1002/9781118453926.ch4.
31. Knothe Tate ML, Adamson JR, Tami AE, Bauer TW. 2004; The osteocyte. Int J Biochem Cell Biol. 36:1–8. DOI: 10.1016/S1357-2725(03)00241-3. PMID: 14592527.
32. Luckheeram RV, Zhou R, Verma AD, Xia B. 2012; CD4+T cells: differentiation and functions. Clin Dev Immunol. 2012:925135. DOI: 10.1155/2012/925135. PMID: 22474485. PMCID: PMC3312336.
33. Ren W, Liu G, Chen S, Yin J, Wang J, Tan B, et al. 2017; Melatonin signaling in T cells: functions and applications. J Pineal Res. 62:e12394. DOI: 10.1111/jpi.12394. PMID: 28152213.
34. Cosmi L, Maggi L, Santarlasci V, Liotta F, Annunziato F. 2014; T helper cells plasticity in inflammation. Cytometry A. 85:36–42. DOI: 10.1002/cyto.a.22348. PMID: 24009159.
35. Hirahara K, Poholek A, Vahedi G, Laurence A, Kanno Y, Milner JD, et al. 2013; Mechanisms underlying helper T-cell plasticity: implications for immune-mediated disease. J Allergy Clin Immunol. 131:1276–87. DOI: 10.1016/j.jaci.2013.03.015. PMID: 23622118. PMCID: PMC3677748.
36. Takayanagi H, Ogasawara K, Hida S, Chiba T, Murata S, Sato K, et al. 2000; T-cell-mediated regulation of osteoclastogenesis by signalling cross-talk between RANKL and IFN-gamma. Nature. 408:600–5. DOI: 10.1038/35046102. PMID: 11117749.
37. Belizário JE, Brandão W, Rossato C, Peron JP. 2016; Thymic and postthymic regulation of naïve CD4(+) T-cell lineage fates in humans and mice models. Mediators Inflamm. 2016:9523628. DOI: 10.1155/2016/9523628. PMID: 27313405. PMCID: PMC4904118.
38. Zhu J, Paul WE. 2008; CD4 T cells: fates, functions, and faults. Blood. 112:1557–69. DOI: 10.1182/blood-2008-05-078154. PMID: 18725574. PMCID: PMC2518872.
39. Palmqvist P, Lundberg P, Persson E, Johansson A, Lundgren I, Lie A, et al. 2006; Inhibition of hormone and cytokine-stimulated osteoclastogenesis and bone resorption by interleukin-4 and interleukin-13 is associated with increased osteoprotegerin and decreased RANKL and RANK in a STAT6-dependent pathway. J Biol Chem. 281:2414–29. DOI: 10.1074/jbc.M510160200. PMID: 16251181.
40. Pacifici R. 2010; T cells: critical bone regulators in health and disease. Bone. 47:461–71. DOI: 10.1016/j.bone.2010.04.611. PMID: 20452473. PMCID: PMC2926258.
41. Vautier S, Sousa M, Brown GD. 2010; C-type lectins, fungi and Th17 responses. Cytokine Growth Factor Rev. 21:405–12. DOI: 10.1016/j.cytogfr.2010.10.001. PMID: 21075040. PMCID: PMC3001956.
42. Zambrano-Zaragoza JF, Romo-Martínez EJ, Durán-Avelar Mde J, García-Magallanes N, Vibanco-Pérez N. 2014; Th17 cells in autoimmune and infectious diseases. Int J Inflam. 2014:651503. DOI: 10.1155/2014/651503. PMID: 25152827. PMCID: PMC4137509.
43. Yago T, Nanke Y, Ichikawa N, Kobashigawa T, Mogi M, Kamatani N, et al. 2009; IL-17 induces osteoclastogenesis from human monocytes alone in the absence of osteoblasts, which is potently inhibited by anti-TNF-alpha antibody: a novel mechanism of osteoclastogenesis by IL-17. J Cell Biochem. 108:947–55. DOI: 10.1002/jcb.22326. PMID: 19728295.
44. Oelzner P, Franke S, Lehmann G, Eidner T, Hein G, Wolf G. 2012; The balance between soluble receptors regulating IL-6 trans-signaling is predictive for the RANKL/osteoprotegerin ratio in postmenopausal women with rheumatoid arthritis. Rheumatol Int. 32:199–206. DOI: 10.1007/s00296-010-1606-z. PMID: 20821212.
45. Miossec P, Korn T, Kuchroo VK. 2009; Interleukin-17 and type 17 helper T cells. N Engl J Med. 361:888–98. DOI: 10.1056/NEJMra0707449. PMID: 19710487.
46. Martinez GJ, Nurieva RI, Yang XO, Dong C. 2008; Regulation and function of proinflammatory TH17 cells. Ann N Y Acad Sci. 1143:188–211. DOI: 10.1196/annals.1443.021. PMID: 19076351. PMCID: PMC5793850.
47. Sato K, Suematsu A, Okamoto K, Yamaguchi A, Morishita Y, Kadono Y, et al. 2006; Th17 functions as an osteoclastogenic helper T cell subset that links T cell activation and bone destruction. J Exp Med. 203:2673–82. DOI: 10.1084/jem.20061775. PMID: 17088434. PMCID: PMC2118166.
48. Dokoupilová E, Aelion J, Takeuchi T, Malavolta N, Sfikakis PP, Wang Y, et al. 2018; Secukinumab after anti-tumour necrosis factor-α therapy: a phase III study in active rheumatoid arthritis. Scand J Rheumatol. 47:276–81. DOI: 10.1080/03009742.2017.1390605. PMID: 29458278.
49. de Rezende LC, Silva IV, Rangel LB, Guimarães MC. 2010; Regulatory T cell as a target for cancer therapy. Arch Immunol Ther Exp (Warsz). 58:179–90. DOI: 10.1007/s00005-010-0075-0. PMID: 20373146.
50. Miyara M, Sakaguchi S. 2007; Natural regulatory T cells: mechanisms of suppression. Trends Mol Med. 13:108–16. DOI: 10.1016/j.molmed.2007.01.003. PMID: 17257897.
51. Wang H, Ying H, Wang S, Gu X, Weng Y, Peng W, et al. 2015; Imbalance of peripheral blood Th17 and Treg responses in patients with chronic obstructive pulmonary disease. Clin Respir J. 9:330–41. DOI: 10.1111/crj.12147. PMID: 24720797.
52. Okamoto K, Nakashima T, Shinohara M, Negishi-Koga T, Komatsu N, Terashima A, et al. 2017; Osteoimmunology: the conceptual framework unifying the immune and skeletal systems. Physiol Rev. 97:1295–349. DOI: 10.1152/physrev.00036.2016. PMID: 28814613.
53. Zaiss MM, Frey B, Hess A, Zwerina J, Luther J, Nimmerjahn F, et al. 2010; Regulatory T cells protect from local and systemic bone destruction in arthritis. J Immunol. 184:7238–46. DOI: 10.4049/jimmunol.0903841. PMID: 20483756.
54. Walsh MC, Choi Y. 2014; Biology of the RANKL-RANK-OPG system in immunity, bone, and beyond. Front Immunol. 5:511. DOI: 10.3389/fimmu.2014.00511. PMID: 25368616. PMCID: PMC4202272.
55. Zhang Z, Yuan W, Deng J, Wang D, Zhang T, Peng L, et al. 2020; Granulocyte colony stimulating factor (G-CSF) regulates neutrophils infiltration and periodontal tissue destruction in an experimental periodontitis. Mol Immunol. 117:110–21. DOI: 10.1016/j.molimm.2019.11.003. PMID: 31765840.
56. Weitzmann MN. 2013; The role of inflammatory cytokines, the RANKL/OPG axis, and the immunoskeletal interface in physiological bone turnover and osteoporosis. Scientifica (Cairo). 2013:125705. DOI: 10.1155/2013/125705. PMID: 24278766. PMCID: PMC3820310.
57. Li Y, Terauchi M, Vikulina T, Roser-Page S, Weitzmann MN. 2014; B cell production of both OPG and RANKL is significantly increased in aged mice. Open Bone J. 6:8–17. DOI: 10.2174/1876525401406010008. PMID: 25984250. PMCID: PMC4429037.
58. Meednu N, Zhang H, Owen T, Sun W, Wang V, Cistrone C, et al. 2016; Production of RANKL by memory B cells: a link between B Cells and bone erosion in rheumatoid arthritis. Arthritis Rheumatol. 68:805–16. DOI: 10.1002/art.39489. PMID: 26554541. PMCID: PMC4956406.
59. Ponzetti M, Rucci N. 2019; Updates on osteoimmunology: what's new on the cross-talk between bone and immune system. Front Endocrinol (Lausanne). 10:236. DOI: 10.3389/fendo.2019.00236. PMID: 31057482. PMCID: PMC6482259.
60. Chang MK, Raggatt LJ, Alexander KA, Kuliwaba JS, Fazzalari NL, Schroder K, et al. 2008; Osteal tissue macrophages are intercalated throughout human and mouse bone lining tissues and regulate osteoblast function in vitro and in vivo. J Immunol. 181:1232–44. DOI: 10.4049/jimmunol.181.2.1232. PMID: 18606677.
61. Tsou CL, Peters W, Si Y, Slaymaker S, Aslanian AM, Weisberg SP, et al. 2007; Critical roles for CCR2 and MCP-3 in monocyte mobilization from bone marrow and recruitment to inflammatory sites. J Clin Invest. 117:902–9. DOI: 10.1172/JCI29919. PMID: 17364026. PMCID: PMC1810572.
62. Auffray C, Sieweke MH, Geissmann F. 2009; Blood monocytes: development, heterogeneity, and relationship with dendritic cells. Annu Rev Immunol. 27:669–92. DOI: 10.1146/annurev.immunol.021908.132557. PMID: 19132917.
63. Sprangers S, de Vries TJ, Everts V. 2016; Monocyte heterogeneity: consequences for monocyte-derived immune cells. J Immunol Res. 2016:1475435. DOI: 10.1155/2016/1475435. PMID: 27478854. PMCID: PMC4958468.
64. Gebraad A, Kornilov R, Kaur S, Miettinen S, Haimi S, Peltoniemi H, et al. 2018; Monocyte-derived extracellular vesicles stimulate cytokine secretion and gene expression of matrix metalloproteinases by mesenchymal stem/stromal cells. FEBS J. 285:2337–59. DOI: 10.1111/febs.14485. PMID: 29732732.
65. Nauseef WM, Borregaard N. 2014; Neutrophils at work. Nat Immunol. 15:602–11. DOI: 10.1038/ni.2921. PMID: 24940954.
66. Scapini P, Cassatella MA. 2014; Social networking of human neutrophils within the immune system. Blood. 124:710–9. DOI: 10.1182/blood-2014-03-453217. PMID: 24923297.
67. Hajishengallis G, Moutsopoulos NM, Hajishengallis E, Chavakis T. 2016; Immune and regulatory functions of neutrophils in inflammatory bone loss. Semin Immunol. 28:146–58. DOI: 10.1016/j.smim.2016.02.002. PMID: 26936034. PMCID: PMC4867283.
68. Poubelle PE, Chakravarti A, Fernandes MJ, Doiron K, Marceau AA. 2007; Differential expression of RANK, RANK-L, and osteoprotegerin by synovial fluid neutrophils from patients with rheumatoid arthritis and by healthy human blood neutrophils. Arthritis Res Ther. 9:R25. DOI: 10.1186/ar2137. PMID: 17341304. PMCID: PMC1906801.
69. Fu SC, Wang P, Qi MX, Peng JP, Lin XQ, Zhang CY, et al. 2019; The associations of TNF-α gene polymorphisms with bone mineral density and risk of osteoporosis: a meta-analysis. Int J Rheum Dis. 22:1619–29. DOI: 10.1111/1756-185X.13647. PMID: 31273943.
70. Kotrych D, Dziedziejko V, Safranow K, Sroczynski T, Staniszewska M, Juzyszyn Z, et al. 2016; TNF-α and IL10 gene polymorphisms in women with postmenopausal osteoporosis. Eur J Obstet Gynecol Reprod Biol. 199:92–5. DOI: 10.1016/j.ejogrb.2016.01.037. PMID: 26914399.
71. Weitzmann MN. 2017; Bone and the immune system. Toxicol Pathol. 45:911–24. DOI: 10.1177/0192623317735316. PMID: 29046115. PMCID: PMC5749254.
72. Zha L, He L, Liang Y, Qin H, Yu B, Chang L, et al. 2018; TNF-α contributes to postmenopausal osteoporosis by synergistically promoting RANKL-induced osteoclast formation. Biomed Pharmacother. 102:369–74. DOI: 10.1016/j.biopha.2018.03.080. PMID: 29571022.
73. Osta B, Benedetti G, Miossec P. 2014; Classical and paradoxical effects of TNF-α on bone homeostasis. Front Immunol. 5:48. DOI: 10.3389/fimmu.2014.00048. PMID: 24592264. PMCID: PMC3923157.
74. Diarra D, Stolina M, Polzer K, Zwerina J, Ominsky MS, Dwyer D, et al. 2007; Dickkopf-1 is a master regulator of joint remodeling. Nat Med. 13:156–63. DOI: 10.1038/nm1538. PMID: 17237793.
75. Ohori F, Kitaura H, Marahleh A, Kishikawa A, Ogawa S, Qi J, et al. 2019; Effect of TNF-α-induced sclerostin on osteocytes during orthodontic tooth movement. J Immunol Res. 2019:9716758. DOI: 10.1155/2019/9716758. PMID: 31341915. PMCID: PMC6612957.
76. Tang M, Tian L, Luo G, Yu X. 2018; Interferon-gamma-mediated osteoimmunology. Front Immunol. 9:1508. DOI: 10.3389/fimmu.2018.01508. PMID: 30008722. PMCID: PMC6033972.
77. Ruscitti P, Cipriani P, Carubbi F, Liakouli V, Zazzeroni F, Di Benedetto P, et al. 2015; The role of IL-1β in the bone loss during rheumatic diseases. Mediators Inflamm. 2015:782382. DOI: 10.1155/2015/782382. PMID: 25954061. PMCID: PMC4410538.
78. Lacativa PG, Farias ML. 2010; Osteoporosis and inflammation. Arq Bras Endocrinol Metabol. 54:123–32. DOI: 10.1590/S0004-27302010000200007. PMID: 20485900.
79. Lee J, Park C, Kim HJ, Lee YD, Lee ZH, Song YW, et al. 2017; Stimulation of osteoclast migration and bone resorption by C-C chemokine ligands 19 and 21. Exp Mol Med. 49:e358. DOI: 10.1038/emm.2017.100. PMID: 28729639. PMCID: PMC5565950.
80. Abdel Meguid MH, Hamad YH, Swilam RS, Barakat MS. 2013; Relation of interleukin-6 in rheumatoid arthritis patients to systemic bone loss and structural bone damage. Rheumatol Int. 33:697–703. DOI: 10.1007/s00296-012-2375-7. PMID: 22531887.
81. Bettelli E, Carrier Y, Gao W, Korn T, Strom TB, Oukka M, et al. 2006; Reciprocal developmental pathways for the generation of pathogenic effector TH17 and regulatory T cells. Nature. 441:235–8. DOI: 10.1038/nature04753. PMID: 16648838.
82. Malysheva K, de Rooij K, Lowik CW, Baeten DL, Rose-John S, Stoika R, et al. 2016; Interleukin 6/Wnt interactions in rheumatoid arthritis: interleukin 6 inhibits Wnt signaling in synovial fibroblasts and osteoblasts. Croat Med J. 57:89–98. DOI: 10.3325/cmj.2016.57.89. PMID: 27106351. PMCID: PMC4856197.
83. Hirota K, Hashimoto M, Ito Y, Matsuura M, Ito H, Tanaka M, et al. 2018; Autoimmune Th17 cells induced synovial stromal and innate lymphoid cell secretion of the cytokine GM-CSF to initiate and augment autoimmune arthritis. Immunity. 48:1220–32.e5. DOI: 10.1016/j.immuni.2018.04.009. PMID: 29802020. PMCID: PMC6024031.
84. Kindstedt E, Koskinen Holm C, Palmqvist P, Sjöström M, Lejon K, Lundberg P. 2019; Innate lymphoid cells are present in gingivitis and periodontitis. J Periodontol. 90:200–7. DOI: 10.1002/JPER.17-0750. PMID: 30070705.
85. Wang T, He C. 2020; TNF-α and IL-6: the link between immune and bone system. Curr Drug Targets. 21:213–27. DOI: 10.2174/1389450120666190821161259. PMID: 31433756.
86. Xu S, Cao X. 2010; Interleukin-17 and its expanding biological functions. Cell Mol Immunol. 7:164–74. DOI: 10.1038/cmi.2010.21. PMID: 20383173. PMCID: PMC4002915.
87. Osta B, Lavocat F, Eljaafari A, Miossec P. 2014; Effects of interleukin-17A on osteogenic differentiation of isolated human mesenchymal stem cells. Front Immunol. 5:425. DOI: 10.3389/fimmu.2014.00425. PMID: 25228904. PMCID: PMC4151036.
88. Klavdianou K, Kanellou A, Daoussis D. 2022; Molecular mechanisms of new bone formation in axial spondyloarthritis. Mediterr J Rheumatol. 33(Suppl 1):115–25. DOI: 10.31138/mjr.33.1.115. PMID: 36127924. PMCID: PMC9450187.
89. Seong S, Kim JH, Kim N. 2016; Pro-inflammatory cytokines modulating osteoclast differentiation and function. J Rheum Dis. 23:148–53. DOI: 10.4078/jrd.2016.23.3.148.
90. Harre U, Georgess D, Bang H, Bozec A, Axmann R, Ossipova E, et al. 2012; Induction of osteoclastogenesis and bone loss by human autoantibodies against citrullinated vimentin. J Clin Invest. 122:1791–802. DOI: 10.1172/JCI60975. PMID: 22505457. PMCID: PMC3336988.
91. Sun M, Rethi B, Krishnamurthy A, Joshua V, Circiumaru A, Hensvold AH, et al. 2019; Anticitrullinated protein antibodies facilitate migration of synovial tissue-derived fibroblasts. Ann Rheum Dis. 78:1621–31. DOI: 10.1136/annrheumdis-2018-214967. PMID: 31481351. PMCID: PMC6900251.
92. Kleyer A, Schett G. 2014; Arthritis and bone loss: a hen and egg story. Curr Opin Rheumatol. 26:80–4. DOI: 10.1097/BOR.0000000000000007. PMID: 24276089.
93. Li S, Yu Y, Yue Y, Liao H, Xie W, Thai J, et al. 2016; Autoantibodies from single circulating plasmablasts react with citrullinated antigens and Porphyromonas gingivalis in rheumatoid arthritis. Arthritis Rheumatol. 68:614–26. DOI: 10.1002/art.39455. PMID: 26474325. PMCID: PMC5770231.
94. Kleyer A, Finzel S, Rech J, Manger B, Krieter M, Faustini F, et al. 2014; Bone loss before the clinical onset of rheumatoid arthritis in subjects with anticitrullinated protein antibodies. Ann Rheum Dis. 73:854–60. DOI: 10.1136/annrheumdis-2012-202958. PMID: 23520034.
95. Bugatti S, Bogliolo L, Vitolo B, Manzo A, Montecucco C, Caporali R. 2016; Anti-citrullinated protein antibodies and high levels of rheumatoid factor are associated with systemic bone loss in patients with early untreated rheumatoid arthritis. Arthritis Res Ther. 18:226. DOI: 10.1186/s13075-016-1116-9. PMID: 27716332. PMCID: PMC5052789.
96. de Vries-Bouwstra JK, Goekoop-Ruiterman YP, Verpoort KN, Schreuder GM, Ewals JA, Terwiel JP, et al. 2008; Progression of joint damage in early rheumatoid arthritis: association with HLA-DRB1, rheumatoid factor, and anti-citrullinated protein antibodies in relation to different treatment strategies. Arthritis Rheum. 58:1293–8. DOI: 10.1002/art.23439. PMID: 18438829.
97. Bukhari M, Thomson W, Naseem H, Bunn D, Silman A, Symmons D, et al. 2007; The performance of anti-cyclic citrullinated peptide antibodies in predicting the severity of radiologic damage in inflammatory polyarthritis: results from the Norfolk Arthritis Register. Arthritis Rheum. 56:2929–35. DOI: 10.1002/art.22868. PMID: 17763407. PMCID: PMC2435419.
98. Syversen SW, Goll GL, van der Heijde D, Landewé R, Lie BA, Odegård S, et al. 2010; Prediction of radiographic progression in rheumatoid arthritis and the role of antibodies against mutated citrullinated vimentin: results from a 10-year prospective study. Ann Rheum Dis. 69:345–51. DOI: 10.1136/ard.2009.113092. PMID: 19648126.
99. Martin-Mola E, Balsa A, García-Vicuna R, Gómez-Reino J, González-Gay MA, Sanmartí R, et al. 2016; Anti-citrullinated peptide antibodies and their value for predicting responses to biologic agents: a review. Rheumatol Int. 36:1043–63. DOI: 10.1007/s00296-016-3506-3. PMID: 27271502.
100. Sakkas LI, Bogdanos DP, Katsiari C, Platsoucas CD. 2014; Anti-citrullinated peptides as autoantigens in rheumatoid arthritis-relevance to treatment. Autoimmun Rev. 13:1114–20. DOI: 10.1016/j.autrev.2014.08.012. PMID: 25182207.
101. Cheng TT, Yu SF, Su FM, Chen YC, Su BY, Chiu WC, et al. 2018; Anti-CCP-positive patients with RA have a higher 10-year probability of fracture evaluated by FRAX®: a registry study of RA with osteoporosis/fracture. Arthritis Res Ther. 20:16. DOI: 10.1186/s13075-018-1515-1. PMID: 29382355. PMCID: PMC5791167.
102. Wu D, Cline-Smith A, Shashkova E, Perla A, Katyal A, Aurora R. 2021; T-cell mediated inflammation in postmenopausal osteoporosis. Front Immunol. 12:687551. DOI: 10.3389/fimmu.2021.687551. PMID: 34276675. PMCID: PMC8278518.
103. Chen TL, Chang KH, Su KY. 2022; Effects of biological/targeted therapies on bone mineral density in inflammatory arthritis. Int J Mol Sci. 23:4111. DOI: 10.3390/ijms23084111. PMID: 35456929. PMCID: PMC9029148.
104. Miao CG, Yang YY, He X, Li XF, Huang C, Huang Y, et al. 2013; Wnt signaling pathway in rheumatoid arthritis, with special emphasis on the different roles in synovial inflammation and bone remodeling. Cell Signal. 25:2069–78. DOI: 10.1016/j.cellsig.2013.04.002. PMID: 23602936.
105. Garnero P, Tabassi NC, Voorzanger-Rousselot N. 2008; Circulating dickkopf-1 and radiological progression in patients with early rheumatoid arthritis treated with etanercept. J Rheumatol. 35:2313–5. DOI: 10.3899/jrheum.080356. PMID: 18843784.
106. Llorente I, García-Castañeda N, Valero C, González-Álvaro I, Castañeda S. 2020; Osteoporosis in rheumatoid arthritis: dangerous liaisons. Front Med (Lausanne). 7:601618. DOI: 10.3389/fmed.2020.601618. PMID: 33330566. PMCID: PMC7719815.
107. Tascioglu F, Oner C, Armagan O. 2003; The effect of low-dose methotrexate on bone mineral density in patients with early rheumatoid arthritis. Rheumatol Int. 23:231–5. DOI: 10.1007/s00296-003-0298-z. PMID: 14504915.
108. Kwon OC, Oh JS, Hong S, Lee CK, Yoo B, Kim YG. 2019; Conventional synthetic disease-modifying antirheumatic drugs and bone mineral density in rheumatoid arthritis patients with osteoporosis: possible beneficial effect of leflunomide. Clin Exp Rheumatol. 37:813–9.
109. Confavreux CB, Chapurlat RD. 2011; Systemic bone effects of biologic therapies in rheumatoid arthritis and ankylosing spondylitis. Osteoporos Int. 22:1023–36. DOI: 10.1007/s00198-010-1462-4. PMID: 20959960.
110. Cohen SB, Dore RK, Lane NE, Ory PA, Peterfy CG, Sharp JT, et al. 2008; Denosumab treatment effects on structural damage, bone mineral density, and bone turnover in rheumatoid arthritis: a twelve-month, multicenter, randomized, double-blind, placebo-controlled, phase II clinical trial. Arthritis Rheum. 58:1299–309. DOI: 10.1002/art.23417. PMID: 18438830.
111. Saidenberg-Kermanac'h N, Corrado A, Lemeiter D, deVernejoul MC, Boissier MC, Cohen-Solal ME. 2004; TNF-alpha antibodies and osteoprotegerin decrease systemic bone loss associated with inflammation through distinct mechanisms in collagen-induced arthritis. Bone. 35:1200–7. DOI: 10.1016/j.bone.2004.07.004. PMID: 15542046.
112. Seriolo B, Ferretti V, Sulli A, Caratto E, Fasciolo D, Cutolo M. 2002; Serum osteocalcin levels in premenopausal rheumatoid arthritis patients. Ann N Y Acad Sci. 966:502–7. DOI: 10.1111/j.1749-6632.2002.tb04254.x. PMID: 12114311.
113. Lange U, Teichmann J, Müller-Ladner U, Strunk J. 2005; Increase in bone mineral density of patients with rheumatoid arthritis treated with anti-TNF-alpha antibody: a prospective open-label pilot study. Rheumatology (Oxford). 44:1546–8. DOI: 10.1093/rheumatology/kei082. PMID: 16263785.
114. Orsolini G, Fassio A, Rossini M, Adami G, Giollo A, Caimmi C, et al. 2019; Effects of biological and targeted synthetic DMARDs on bone loss in rheumatoid arthritis. Pharmacol Res. 147:104354. DOI: 10.1016/j.phrs.2019.104354. PMID: 31306774.
115. Seriolo B, Paolino S, Sulli A, Ferretti V, Cutolo M. 2006; Bone metabolism changes during anti-TNF-alpha therapy in patients with active rheumatoid arthritis. Ann N Y Acad Sci. 1069:420–7. DOI: 10.1196/annals.1351.040. PMID: 16855169.
116. Fassio A, Adami G, Gatti D, Orsolini G, Giollo A, Idolazzi L, et al. 2019; Inhibition of tumor necrosis factor-alpha (TNF-alpha) in patients with early rheumatoid arthritis results in acute changes of bone modulators. Int Immunopharmacol. 67:487–9. DOI: 10.1016/j.intimp.2018.12.050. PMID: 30599401.
117. Lories RJ, de Vlam K, Luyten FP. 2010; Are current available therapies disease-modifying in spondyloarthritis? Best Pract Res Clin Rheumatol. 24:625–35. DOI: 10.1016/j.berh.2010.05.005. PMID: 21035084.
118. Lories RJ, Haroon N. 2014; Bone formation in axial spondyloarthritis. Best Pract Res Clin Rheumatol. 28:765–77. DOI: 10.1016/j.berh.2014.10.008. PMID: 25488783.
119. Yasunori K, Masaaki T, Tetsuyuki N, Hayato K, Akira N. 2008; Reduction of urinary levels of pyridinoline and deoxypyridinoline and serum levels of soluble receptor activator of NF-kappaB ligand by etanercept in patients with rheumatoid arthritis. Clin Rheumatol. 27:1093–101. DOI: 10.1007/s10067-008-0870-8. PMID: 18338203.
120. Ziolkowska M, Kurowska M, Radzikowska A, Luszczykiewicz G, Wiland P, Dziewczopolski W, et al. 2002; High levels of osteoprotegerin and soluble receptor activator of nuclear factor kappa B ligand in serum of rheumatoid arthritis patients and their normalization after anti-tumor necrosis factor alpha treatment. Arthritis Rheum. 46:1744–53. DOI: 10.1002/art.10388. PMID: 12124857.
121. Jura-Półtorak A, Szeremeta A, Olczyk K, Zoń-Giebel A, Komosińska-Vassev K. 2021; Bone metabolism and RANKL/OPG ratio in rheumatoid arthritis women treated with TNF-α inhibitors. J Clin Med. 10:2905. DOI: 10.3390/jcm10132905. PMID: 34209821. PMCID: PMC8267676.
122. Hoff M, Kvien TK, Kälvesten J, Elden A, Haugeberg G. 2009; Adalimumab therapy reduces hand bone loss in early rheumatoid arthritis: explorative analyses from the PREMIER study. Ann Rheum Dis. 68:1171–6. DOI: 10.1136/ard.2008.091264. PMID: 18801760. PMCID: PMC2689520.
123. Krieckaert CL, Nurmohamed MT, Wolbink G, Lems WF. 2013; Changes in bone mineral density during long-term treatment with adalimumab in patients with rheumatoid arthritis: a cohort study. Rheumatology (Oxford). 52:547–53. DOI: 10.1093/rheumatology/kes320. PMID: 23221326.
124. Marotte H, Pallot-Prades B, Grange L, Gaudin P, Alexandre C, Miossec P. 2007; A 1-year case-control study in patients with rheumatoid arthritis indicates prevention of loss of bone mineral density in both responders and nonresponders to infliximab. Arthritis Res Ther. 9:R61. DOI: 10.1186/ar2219. PMID: 17597527. PMCID: PMC2206336.
125. Vis M, Voskuyl AE, Wolbink GJ, Dijkmans BA, Lems WF. OSTRA Study Group. 2005; Bone mineral density in patients with rheumatoid arthritis treated with infliximab. Ann Rheum Dis. 64:336–7. DOI: 10.1136/ard.2003.017780. PMID: 15647447. PMCID: PMC1755334.
126. Wijbrandts CA, Klaasen R, Dijkgraaf MG, Gerlag DM, van Eck-Smit BL, Tak PP. 2009; Bone mineral density in rheumatoid arthritis patients 1 year after adalimumab therapy: arrest of bone loss. Ann Rheum Dis. 68:373–6. DOI: 10.1136/ard.2008.091611. PMID: 18408246. PMCID: PMC2945478.
127. Alenazy MF, Saheb Sharif-Askari F, Omair MA, El-Wetidy MS, Omair MA, Mitwalli H, et al. 2021; Abatacept enhances blood regulatory B cells of rheumatoid arthritis patients to a level that associates with disease remittance. Sci Rep. 11:5629. DOI: 10.1038/s41598-021-83615-0. PMID: 33707483. PMCID: PMC7952390.
128. Genovese MC, Becker JC, Schiff M, Luggen M, Sherrer Y, Kremer J, et al. 2005; Abatacept for rheumatoid arthritis refractory to tumor necrosis factor alpha inhibition. N Engl J Med. 353:1114–23. DOI: 10.1056/NEJMoa050524. PMID: 16162882.
129. Axmann R, Herman S, Zaiss M, Franz S, Polzer K, Zwerina J, et al. 2008; CTLA-4 directly inhibits osteoclast formation. Ann Rheum Dis. 67:1603–9. DOI: 10.1136/ard.2007.080713. PMID: 18203760.
130. Bozec A, Zaiss MM, Kagwiria R, Voll R, Rauh M, Chen Z, et al. 2014; T cell costimulation molecules CD80/86 inhibit osteoclast differentiation by inducing the IDO/tryptophan pathway. Sci Transl Med. 6:235ra60. DOI: 10.1126/scitranslmed.3007764. PMID: 24807557.
131. Okada H, Kajiya H, Omata Y, Matsumoto T, Sato Y, Kobayashi T, et al. 2019; CTLA4-Ig directly inhibits osteoclastogenesis by interfering with intracellular calcium oscillations in bone marrow macrophages. J Bone Miner Res. 34:1744–52. DOI: 10.1002/jbmr.3754. PMID: 31067348.
132. Roser-Page S, Vikulina T, Zayzafoon M, Weitzmann MN. 2014; CTLA-4Ig-induced T cell anergy promotes Wnt-10b production and bone formation in a mouse model. Arthritis Rheumatol. 66:990–9. DOI: 10.1002/art.38319. PMID: 24757150. PMCID: PMC3994890.
133. Nagao N, Wakabayashi H, Miyamura G, Kato S, Naito Y, Sudo A. 2020; CTLA-4Ig improves hyperalgesia in a mouse model of osteoporosis. Int J Mol Sci. 21:9479. DOI: 10.3390/ijms21249479. PMID: 33322156. PMCID: PMC7763121.
134. Bedi B, Li JY, Grassi F, Tawfeek H, Weitzmann MN, Pacifici R. 2010; Inhibition of antigen presentation and T cell costimulation blocks PTH-induced bone loss. Ann N Y Acad Sci. 1192:215–21. DOI: 10.1111/j.1749-6632.2009.05216.x. PMID: 20392239. PMCID: PMC3269765.
135. Kawashiri SY, Endo Y, Nishino A, Okamoto M, Tsuji S, Takatani A, et al. 2021; Effect of abatacept treatment on serum osteoclast-related biomarkers in patients with rheumatoid arthritis (RA): a multicenter RA ultrasound prospective cohort in Japan. Medicine (Baltimore). 100:e26592. DOI: 10.1097/MD.0000000000026592. PMID: 34260539. PMCID: PMC8284735.
136. Kawashiri SY, Endo Y, Nishino A, Okamoto M, Tsuji S, Takatani A, et al. 2021; Association between serum bone biomarker levels and therapeutic response to abatacept in patients with rheumatoid arthritis (RA): a multicenter, prospective, and observational RA ultrasound cohort study in Japan. BMC Musculoskelet Disord. 22:506. DOI: 10.1186/s12891-021-04392-5. PMID: 34074293. PMCID: PMC8171043.
137. Tada M, Inui K, Sugioka Y, Mamoto K, Okano T, Koike T. 2018; Abatacept might increase bone mineral density at femoral neck for patients with rheumatoid arthritis in clinical practice: AIRTIGHT study. Rheumatol Int. 38:777–84. DOI: 10.1007/s00296-017-3922-z. PMID: 29294175.
138. Chen MH, Yu SF, Chen JF, Chen WS, Liou TL, Chou CT, et al. 2021; Different effects of biologics on systemic bone loss protection in rheumatoid arthritis: an interim analysis of a three-year longitudinal cohort study. Front Immunol. 12:783030. DOI: 10.3389/fimmu.2021.783030. PMID: 34987510. PMCID: PMC8720866.
139. Wunderlich C, Oliviera I, Figueiredo CP, Rech J, Schett G. 2017; Effects of DMARDs on citrullinated peptide autoantibody levels in RA patients-a longitudinal analysis. Semin Arthritis Rheum. 46:709–14. DOI: 10.1016/j.semarthrit.2016.09.011. PMID: 28109618.
140. Jansen DTSL, Emery P, Smolen JS, Westhovens R, Le Bars M, Connolly SE, et al. 2018; Conversion to seronegative status after abatacept treatment in patients with early and poor prognostic rheumatoid arthritis is associated with better radiographic outcomes and sustained remission: post hoc analysis of the AGREE study. RMD Open. 4:e000564. DOI: 10.1136/rmdopen-2017-000564. PMID: 29657830. PMCID: PMC5892779.
141. Kerschbaumer A, Sepriano A, Smolen JS, van der Heijde D, Dougados M, van Vollenhoven R, et al. 2020; Efficacy of pharmacological treatment in rheumatoid arthritis: a systematic literature research informing the 2019 update of the EULAR recommendations for management of rheumatoid arthritis. Ann Rheum Dis. 79:744–59. DOI: 10.1136/annrheumdis-2019-216656. PMID: 32033937. PMCID: PMC7286044.
142. Humby F, Durez P, Buch MH, Lewis MJ, Rizvi H, Rivellese F, et al. 2021; Rituximab versus tocilizumab in anti-TNF inadequate responder patients with rheumatoid arthritis (R4RA): 16-week outcomes of a stratified, biopsy-driven, multicentre, open-label, phase 4 randomised controlled trial. Lancet. 397:305–17. DOI: 10.1016/S0140-6736(20)32341-2. PMID: 33485455.
143. Sun W, Meednu N, Rosenberg A, Rangel-Moreno J, Wang V, Glanzman J, et al. 2018; B cells inhibit bone formation in rheumatoid arthritis by suppressing osteoblast differentiation. Nat Commun. 9:5127. DOI: 10.1038/s41467-018-07626-8. PMID: 30510188. PMCID: PMC6277442.
144. Yeo L, Toellner KM, Salmon M, Filer A, Buckley CD, Raza K, et al. 2011; Cytokine mRNA profiling identifies B cells as a major source of RANKL in rheumatoid arthritis. Ann Rheum Dis. 70:2022–8. DOI: 10.1136/ard.2011.153312. PMID: 21742639. PMCID: PMC3184241.
145. Kolomansky A, Kaye I, Ben-Califa N, Gorodov A, Awida Z, Sadovnic O, et al. 2020; Anti-CD20-mediated B cell depletion is associated with bone preservation in lymphoma patients and bone mass increase in mice. Front Immunol. 11:561294. DOI: 10.3389/fimmu.2020.561294. PMID: 33193330. PMCID: PMC7604358.
146. Boumans MJ, Thurlings RM, Yeo L, Scheel-Toellner D, Vos K, Gerlag DM, et al. 2012; Rituximab abrogates joint destruction in rheumatoid arthritis by inhibiting osteoclastogenesis. Ann Rheum Dis. 71:108–13. DOI: 10.1136/annrheumdis-2011-200198. PMID: 22072013.
147. Wheater G, Elshahaly M, Naraghi K, Tuck SP, Datta HK, van Laar JM. 2018; Changes in bone density and bone turnover in patients with rheumatoid arthritis treated with rituximab, results from an exploratory, prospective study. PLoS One. 13:e0201527. DOI: 10.1371/journal.pone.0201527. PMID: 30080871. PMCID: PMC6078302.
148. Axmann R, Böhm C, Krönke G, Zwerina J, Smolen J, Schett G. 2009; Inhibition of interleukin-6 receptor directly blocks osteoclast formation in vitro and in vivo. Arthritis Rheum. 60:2747–56. DOI: 10.1002/art.24781. PMID: 19714627.
149. Bijlsma JWJ, Welsing PMJ, Woodworth TG, Middelink LM, Pethö-Schramm A, Bernasconi C, et al. 2016; Early rheumatoid arthritis treated with tocilizumab, methotrexate, or their combination (U-Act-Early): a multicentre, randomised, double-blind, double-dummy, strategy trial. Lancet. 388:343–55. DOI: 10.1016/S0140-6736(16)30363-4. PMID: 27287832.
150. Briot K, Rouanet S, Schaeverbeke T, Etchepare F, Gaudin P, Perdriger A, et al. 2015; The effect of tocilizumab on bone mineral density, serum levels of Dickkopf-1 and bone remodeling markers in patients with rheumatoid arthritis. Joint Bone Spine. 82:109–15. DOI: 10.1016/j.jbspin.2014.10.015. PMID: 25557658.
151. Karsdal MA, Schett G, Emery P, Harari O, Byrjalsen I, Kenwright A, et al. 2012; IL-6 receptor inhibition positively modulates bone balance in rheumatoid arthritis patients with an inadequate response to anti-tumor necrosis factor therapy: biochemical marker analysis of bone metabolism in the tocilizumab RADIATE study (NCT00106522). Semin Arthritis Rheum. 42:131–9. DOI: 10.1016/j.semarthrit.2012.01.004. PMID: 22397953.
152. Kanbe K, Nakamura A, Inoue Y, Hobo K. 2012; Osteoprotegerin expression in bone marrow by treatment with tocilizumab in rheumatoid arthritis. Rheumatol Int. 32:2669–74. DOI: 10.1007/s00296-011-2021-9. PMID: 21789615.
153. Terpos E, Fragiadaki K, Konsta M, Bratengeier C, Papatheodorou A, Sfikakis PP. 2011; Early effects of IL-6 receptor inhibition on bone homeostasis: a pilot study in women with rheumatoid arthritis. Clin Exp Rheumatol. 29:921–5.
154. Abu-Shakra M, Zisman D, Balbir-Gurman A, Amital H, Levy Y, Langevitz P, et al. 2018; Effect of tocilizumab on fatigue and bone mineral density in patients with rheumatoid arthritis. Isr Med Assoc J. 20:239–44.
155. Kume K, Amano K, Yamada S, Kanazawa T, Ohta H, Hatta K, et al. 2014; The effect of tocilizumab on bone mineral density in patients with methotrexate-resistant active rheumatoid arthritis. Rheumatology (Oxford). 53:900–3. DOI: 10.1093/rheumatology/ket468. PMID: 24441151.
156. Chen YM, Chen HH, Huang WN, Liao TL, Chen JP, Chao WC, et al. 2017; Tocilizumab potentially prevents bone loss in patients with anticitrullinated protein antibody-positive rheumatoid arthritis. PLoS One. 12:e0188454. DOI: 10.1371/journal.pone.0188454. PMID: 29155868. PMCID: PMC5695761.
157. Suzuki T, Nakamura Y, Kato H. 2018; Effects of denosumab on bone metabolism and bone mineral density with anti-TNF inhibitors, tocilizumab, or abatacept in osteoporosis with rheumatoid arthritis. Ther Clin Risk Manag. 14:453–9. DOI: 10.2147/TCRM.S156350. PMID: 29535527. PMCID: PMC5840187.
Fig. 2
Immunopathogenesis of osteoporosis in RA. DC: dendritic cell, IL: interleukin, DKK-1: dicckopf-1, Th: T helper, Treg: regulator T, TNF: tumor necrosis factor, MSC: mesenchymal stem cell, IFN: interferon, HSC: mesenchymal stem cell, OPG: osteoprotegerin, RANKL: receptor activator of NF-κB ligand, RANK: receptor activator of NF-κB, G-CSF: granulocyte colony stimulating factor [21].
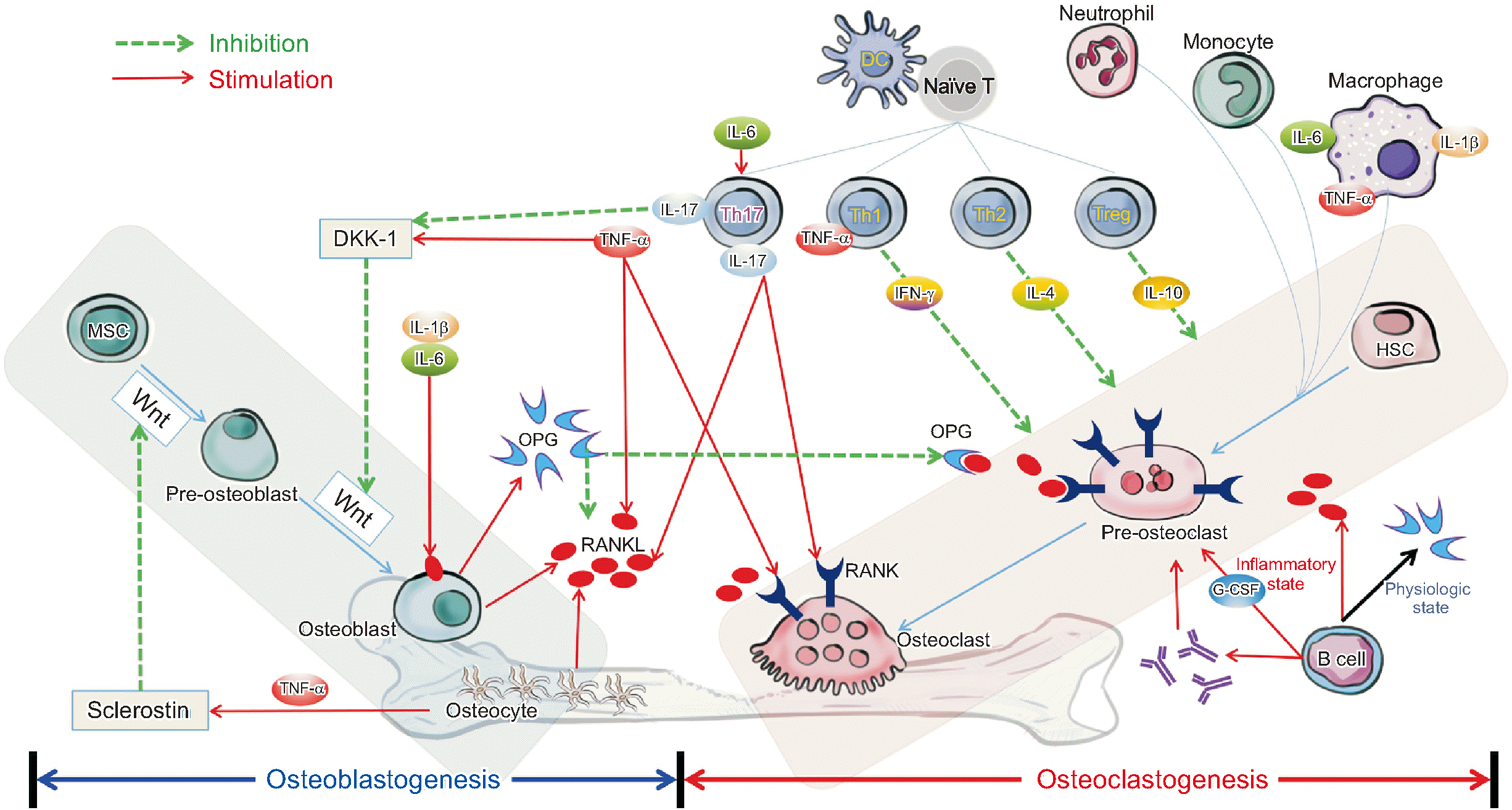
Table 1
Summary of the role of main cells in bone biology
Table 2
Summary of the role of cytokines in bone biology
Table 3
Summary of effects of biologics on bone biochemical marker in RA