Abstract
Since, oxidative stress has been suggested as one of the mechanisms underlying arsenic-induced toxicity, the present study focused on the role of antioxidant (curcumin) supplementation on behavioral, biochemical, and morphological alterations with context to mice hippocampus (CA1) following arsenic trioxide (As2O3) administration. Healthy male Swiss albino mice were divided into control and experimental groups. As2O3 (2 mg/kg bw) alone or along with curcumin (100 mg/kg bw) was administered to experimental groups by oral route for 45 days whereas the control groups received either no treatment or vehicle for curcumin. Animals were subjected to behavioral study towards the end of the experimental period (day 33–45). On day 46, the brain samples were obtained and subjected either to immersion fixation (for morphometric observations) or used afresh for biochemical test. Behavioral tests (open field, elevated plus maze, and Morris water maze) revealed enhanced anxiety levels and impairment of cognitive functions in As2O3 alone treated groups whereas a trend of recovery was evident in mice simultaneously treated with As2O3 and curcumin. Morphological observations showed noticeable reduction in stratum pyramidale thickness (CA1), along with decrease in density and size of pyramidal neurons in As2O3 alone exposed group as compared to As2O3+Cu co-treated group. Hippocampal glutathione levels were found to be downregulated in animals receiving As2O3 as against the levels of controls and curcumin supplemented animals, thereby, suggestive of beneficial role of curcumin on As2O3 induced adverse effects.
Arsenic has been labelled as the “King of Poisons” and the “Poison of Kings” based on its potent toxic nature [1]. With ubiquitous distribution in the environment, arsenic ranks first in U.S. Environmental Protection Agency list of prioritized pollutants. Both organic and inorganic forms of arsenic exist with various valency states (–3, 0, +3, +5) and the inorganic form with trivalent oxidation state is reported to be more toxic [2]. Consumption of groundwater contaminated with inorganic arsenic continues to be one of the major sources of exposure to arsenic in humans. Chronic exposure to arsenic has been reported to target various organ systems such as integumentary, renal, reproductive, hepato-biliary, gastric, cardiovascular, nervous, etc. [3]. In spite of reported arsenic-induced adverse effects on biological systems, arsenic and its compounds are being continuously harnessed for various biomedical applications.
In the realm of inorganic arsenic salts, arsenic trioxide (As2O3) has found use as an effective salvage agent for treating acute promyelocytic leukemia (APL) [4]. As2O3 was approved by Food and Drug Administration, USA in 2000 for inducing complete remission in APL patients and the therapeutic dosage of 0.15 mg/kg bw As2O3 for a period of 60 days was recommended [5]. Apart from its use in APL and other hematologic malignancies such as multiple myeloma [6], myelodysplastic syndromes [7] etc., it is being considered as treatment option for various solid tumors as well such as cancer of bladder, breast, cervix, colon, esophagus, liver, lung, germ cell tumors, melanoma etc. (http://www.clinicaltrials.gov). Nevertheless, a higher dosage of As2O3 than the therapeutic dosage used in APL patients is under consideration for treatment of solid tumors [8, 9]. As2O3 acts by targeting multiple cellular pathways that lead to apoptosis and myeloid differentiation (desired results in APL). As2O3 targets oncogenic promyelocytic leukemia/retinoic acid receptor alpha fusion protein and induces its degradation, hence, allowing the malignant promyelocytes to overcome their maturation block [10]. Other alternative mechanisms of action of As2O3 have been proposed, including mitotic arrest, inhibition of GTP dependent polymerization and microtubule formation and modulation of the glutathione (GSH) redox system [11].
A number of studies suggest As2O3 induced adverse effects on various organ systems such as gastrointestinal tract (diarrhea, vomiting, nausea, anorexia, abdominal pain), cardiovascular system (tachycardia, electrocardiogram abnormalities, atrial and ventricular arrhythmias, hypertrophy, complete atrioventricular block), central nervous system (CNS) etc., thereby posing a challenge to its use as an effective therapeutic agent [12, 13]. Increasing lines of evidence suggest that CNS is one of the major targets of arsenic-induced toxicity based on reports of peripheral neuropathy, encephalopathy, delirium, headache, coma, seizures, hallucinations, etc. in APL patients receiving As2O3 therapy [11].
Hippocampus is one of the brain areas associated with functional diversities [14, 15]. The increased vulnerability of its CA1 sub-region towards various insults such as ischemia, toxins, vascular stress, aging, epileptic seizures [16, 17] makes it a crucial study area for understanding the pathophysiology underlying major neuropsychiatric disorders (Alzheimer’s Disease, Parkinson’s Disease, Schizophrenia, Fronto-temporal Dementia) [18].
Oxidative stress has been proposed as one of the possible mechanisms underlying a number of stress-induced conditions in the CNS, being characterized by induction of selective neuronal vulnerability (SNV) [17]. Hence, the role of supplementing non-toxic, natural antioxidants as dietary adjuvants has emerged as a viable strategy in coping up with oxidative stress generated in a number of neurodegenerative disorders.
Curcumin, a dietary spice derived from rhizomes of plant Curcuma longa, is one of the most cited antioxidants experimented with over the past several years. Its wide spectrum of biological activities includes its anti-inflammatory, anti-carcinogenic, anti-mutagenic, anti-fibrotic, anti-microbial and antioxidant actions. A number of in-vivo studies have reported the potential of curcumin in enhancing the expression of various detoxifying enzymes such as glutathione-S-transferase, GSH reductase, superoxide dismutase, and reduced GSH in liver [19], small intestine, kidney, and brain [20, 21].
The present study was undertaken to determine the effects of curcumin supplementation on functional and structural features of hippocampus of mice subjected to As2O3 exposure.
Adult male Swiss albino mice were procured from the Central Animal Facility (CAF) of the Institute after obtaining ethical clearance from the Institutional Animal Ethical Committee (852/IAEC/15). Female mice were excluded from the study to avoid the interference of positive influence of female hormone (estrogen) on neurobehavior. The animal care was in accordance with the guidelines provided by Committee for the Purpose of Control and Supervision on Experiments on Animals. The animals were housed in cages and subjected to 12/12-hour light/dark cycle with ambient temperature (20°C–24°C) and humidity (50%–60%) conditions. The animals had ad libitum access to water and standard pellet diet.
Animals (n=6/group/subgroup) were randomly divided into control (with no treatment [Ia] or receiving 5% gum acacia [Ib]) and experimental groups (II, As2O3 alone [2 mg/kg bw]; III, curcumin alone [100 mg/kg bw]; IV, As2O3+curcumin [2 mg/kg bw+100 mg/kg bw]). In the present study, 5% gum acacia and distilled water were used as a vehicle for curcumin and As2O3 respectively. The test substances (As2O3 and curcumin), procured from Sigma-Aldrich, (St. Louis, MO, USA), were administered through oral gavage (22G) to the experimental animals over a period of 45 days.
The dosage of As2O3 was calculated from human equivalent dose of 0.15 mg/kg bw (×45 days) [5] and the curcumin dosage was adopted from reported literature [22, 23]. A battery of behavioral tests was carried out from day 33–45 of the experimental period to assess the anxiety levels and spatial learning and memory.
On day 46, the animals were anaesthetized with sodium pentobarbital (<200 mg/kg bw intraperitoneally) and sacrificed by euthanasia. The brain samples were subjected to either immersion fixation (for morphological and morphometric observations) or processed afresh for biochemical study.
The animals were allowed to adapt to the novel environment (temperature, light, and humidity controlled) of the setup for an hour prior to actual behavioral testing.
Open field test (OFT) is widely used for assessing locomotor activity, exploration ability and anxiety levels in rodents [24]. The OFT apparatus with its square (40×40 cm2) arena divided into central (20×20 cm2) and the peripheral areas, is connected to automated computer system and inbuilt tracking software ANY-maze (version 4.99, Stoelting, Wood Dale, IL, USA). After habituation, mice were placed individually in the center of the field and left undisturbed to explore the field freely (5 minutes). The various parameters such as time spent in the central square, and the total distance traveled in the chamber were recorded. The apparatus was cleaned with 70% alcohol before testing a new animal to eliminate possible bias due to odors left by previous animals.
Elevated plus maze (EPM) is extensively used for assessing anxiety levels in rodents [25]. Of the four arms (50 cm×10 cm) of the maze extending from a central square (5×5 cm), two of the arms were open and two were closed (40 cm high walls). The maze was kept 25 cm above the floor. The test sessions commenced by placing the mice individually on the central square facing towards one of the open arms and allowing it to explore the maze freely (5 minutes). The number of entries and the time spent in the open and closed arms of the maze was recorded by ANY-maze software (version 4.99, Stoelting).
Morris water maze (MWM) [26] is widely used to assess spatial learning and memory in rodents. The protocol advocated by Vorhees and Williams [27] was followed with some modifications. The circular pool (1.5 m diameter and 30 cm height) with inside painted black, was placed in the middle of the test room (dimly lit) and filled (24 cm deep) with water (25°C–27°C). Spatial cues (e.g., circles, squares, or triangles) were fixed at different points on the walls around the maze and one dominant visual cue was attached directly on the pool wall near the location of platform. The position of submerged platform remained constant (SW quadrant) throughout the test sessions followed by its removal from the quadrant during probe trial. Various phases of the test included the habituation phase, acquisition phase, and the probe trial.
In habituation (day 39–40) and acquisition phase (day 41–44), the animals underwent 4 test sessions (60 seconds each) of training, with each session alternating with inter-trial interval (10–15 seconds). The animal was gently released into the pool from randomly decided start locations (N, E, SE, and NW) and allowed to swim freely. The time taken by the animal to find the submerged platform (escape latency) and the total distance traveled (swim path) was recorded by ANY-maze software (stoelting). For probe trial (reference memory) on day 45, the time spent in the target quadrant (SW) and the number of platform site crossings were recorded.
Freshly excised hippocampal tissue was weighed, blotted dry and sonicated for 1 minute at 4°C in 0.3 M sodium phosphate buffer (pH 8.4) to obtain 10% w/v homogenate. Centrifugation was carried out at 5,000 rpm for 10–15 minutes and the clear supernatant was collected. 0.5 ml of coloring reagent DTNB (5,5-dithiobis 2-nitrobenzoic acid, Ellman’s reagent) was added to reaction mixture (4.5 ml) consisting of 0.2 ml of supernatant, 0.3 ml of 5% TCA and 4 ml of 0.3 M sodium phosphate (Na2HPO4) buffer (pH 8.4). The absorbance was read at 412 nm using UV visible spectrophotometer (Biomate 3S, Thermo Scientific, Waltham, MA, USA). With reference to standard curve drawn by using different known concentrations of GSH solution, GSH content was calculated and expressed as µg/mg wet tissue [28].
Microscopic observations were carried out on 7-μm-thick paraffin sections (Bregma, –1.34 to –3.52) [29]. For morphometric parameters, high-power (40×) photomicrographs of CA1 region of hippocampus were captured with the help of Nikon Digital Camera System (DS-Fil-U2) attached to microscope (Nikon E-600, Tokyo, Japan). Neuronal density, cell layer thickness, and neuronal size were analyzed using image analysis system (NIS-Elements-AR 3.1, Nikon Imaging Software). The first section was randomly chosen and the subsequent section was every seventh from it with a total of six sections per animal being analyzed [30]. A rectangular frame (250×40 μm2) was superimposed on randomly selected three reference areas [31] along the strip of CA1. For the estimation of neuronal density, the cell bodies of the neurons with or without nucleoli lying within the frame were taken into consideration [32]. The cell bodies lying along the left and lower margin of the counting frame were excluded [33], and those along right and upper margin of the frame were considered for the analysis. For the measurement of neuronal area, minimum of 24 neurons per section, an average of 144 neurons per animal and 864 neurons per group were taken into consideration. The neurons with the characterstic multipolar cell body, well-defined nucleus, intact cell membrane and abundant cytoplasmic nissl substance [34] were included for morphometric observation.
SPSS version 17 (SPSS Inc., Chicago, IL, USA) and Graphpad Prism version 6 (San Diego, CA, USA) was used for analyzing data. One-way analysis of variance (ANOVA), followed by the Bonferroni test with multiple comparisons was carried out to determine differences among the groups. Differences were considered statistically significant at P<0.05 and 0.01.
All along the experimental period (day 1–45), no obvious difference was noted in general features such as hair loss, rearing, grooming, state of alertness, etc. among the control and the experimental animals.
The body weight of the control and the experimental animals were comparable to begin with. Towards the end of the experimental period (day 45), the animals exposed to As2O3 alone showed a significant difference in the percentage change in body weight when compared to other groups (Table 1).
The values for brain weight, weight of hippocampus, and encephalization quotient were comparable amongst the control and the experimental groups (Table 1).
The total distance traveled (Fig. 1A, B) by the mice in the entire open field (measure of exploratory/locomotor activity) and the time spent in the central square (Fig. 1A, B) of the chamber (anxiety level) was significantly less in case of animals receiving As2O3 alone, thereby suggestive of reduced exploratory activity and increased anxiety levels in these animals. However, the total distance traveled in the open field and the percentage time spent in the central arena was significantly more in case of animals receiving either curcumin alone or curcumin with As2O3, thereby suggesting ameliorative role of curcumin on arsenic-induced reduced locomotion and anxiety-related behavioral deficits.
Enhanced anxiety levels in As2O3 alone exposed animals were also evident by the decreased number of entries as well as lesser time spent in the open arms, thereby suggestive of their fear for height and open environment. However, percentage time spent in the open arms and number of open arm entries (Fig. 1C, D) was significantly more in case of animals receiving either curcumin alone or curcumin and As2O3.
For escape latency, significant differences were observed during the acquisition phase amongst the control and experimental groups. As2O3 alone treated animals traveled longer distance (Fig. 1E, F) for locating the platform as compared to the control and other experimental groups (curcumin alone or curcumin+As2O3 groups). However, a significant decrease in escape latency was evident in case of animals receiving curcumin alone or curcumin+As2O3, thereby suggestive of gain in space allocation for submerged platform in these animals.
During the probe trial (Fig. 1G, H), significant decrease was observed in the number of platform site crossings, as well as time spent in the target quadrant (SW) by As2O3 alone exposed animals when compared to controls, thereby suggestive of As2O3 induced impairment of spatial memory retention. However, exogenous supplementation of curcumin resulted in substantial increase in the number of platform site crossings as well as time spent in the target quadrant, thereby suggestive of the beneficial role of curcumin in improving the retention memory.
The hippocampal GSH level was significantly lower in the As2O3 alone exposed animals as compared to the controls (Fig. 2). However, curcumin alone and As2O3+curcumin co-treated animals showed significantly higher GSH levels as compared to As2O3 alone exposed animals, thereby, suggestive of possible antioxidant role of curcumin.
Qualitative observations of cresyl violet stained coronal sections of CA1 region of hippocampus showed intact laminar organization and stratification as Stratum oriens, Stratum pyramidale (SP), Stratum radiatum, Stratum lacunosum moleculare across control and experimental animals (Fig. 3). On the whole, the pyramidal neuronal profiles exhibited rounded, pale nucleus with distinct nucleolus and brightly stained discernable nissl substance around (Fig. 3A, C, D), suggestive of their healthy status. Neuronal damage was evident in the form of pyknotic nuclei and indistinct nucleolus (Fig. 3B) in As2O3 alone exposed animals. The loose packed pyramidal cells in CA1 of As2O3 alone exposed animals were distinct from tightly packed cell arrangement in SP of the control and Cu–co-treated animals (Fig. 3A–D).
Quantitative observations (Table 2) revealed significant reduction by 20% and 15% in mean neuronal density (per mm2) and mean neuronal area (μm2) of pyramidal cells in As2O3 alone exposed group as against the control group. An increase in neuronal density (by 10%) and a significant increase in mean neuronal area was observed in the experimental group receiving curcumin (100 mg/kg bw) with As2O3 (2 mg/kg bw).
On the similar lines, the mean values for layer thickness of SP (CA1) in As2O3 alone exposed animals were low as compared to the controls, curcumin alone, and curcumin+As2O3 treated groups.
The present study was undertaken to evaluate the effect(s) of exogenously administered curcumin on behavioral, biochemical, morphological, and morphometric features with context to hippocampus in adult mice subjected to As2O3 exposure. To some extent, the dosage of As2O3 and duration of its administration was in accordance with human therapeutic dosage used in treatment of APL. Hippocampus was chosen as the study area based on its role in modulation of stress responses (anxiety and fear) and cognitive abilities (learning, memory) [35].
Our observations of a significant increase in the percentage change in body weight of As2O3 alone treated animals is in line with the earlier reports of weight gain following As2O3 treatment [36, 37]. Moreover, the commercial use of arsenic based products (roxarsone) as dietary additives for inducing faster weight gain in poultry animals [38, 39] substantially supports the role of arsenic in gaining weight.
In As2O3 alone treated animals, increase in anxiety level was suggested by the reduced number of entries and lesser time spent in the open area of both OFT and EPM. Similar observations were put forth by Chang et al. [40], who observed enhanced anxiety levels in mice exposed to As2O3 at dosage of 10 mg/l. Several experimental studies on animal models have demonstrated alteration in locomotor activity following arsenic exposure, the nature of alteration varying with the dose, route of administration, duration of exposure along with the gender and specific strain of the animal [41, 42]. In the present study, lesser distance traveled in the entire open field (OFT) by As2O3 alone exposed animals suggested reduced locomotor activity. Recent reports have suggested the important role of hippocampus in control of locomotion [43] based on its connections with medial septum from where it receives cholinergic, glutamatergic, and GABAergic afferents for synapse formation [44, 45]. Decreased locomotor activity could be the result of arsenic-induced alterations in neurotransmitter levels involved in locomotion such as dopamine [46, 47].
An association between chronic inorganic arsenic exposure and CNS malfunctioning such as deficits in intelligence, verbal comprehension, attention, learning, and memory have been reported [48, 49]. Cognitive deficits in animal models following exposure to substances of abuse such as alcohol and morphine have also been reported [50]. Hippocampus is one of the key areas involved in learning, memory, emotions and cognitive map formation [18]. In the present study, MWM testing (acquisition phase) indicated prolonged escape latency in As2O3 alone exposed mice with the advancing days, thereby suggesting the inability of these animals to recall the spatial information. A number of studies based on animal experimentation have reported an association between arsenic exposure and cognitive functions [51, 52]. Neurotransmitters and their receptors play an integral role in synaptic transmission (CNS), thereby influencing the functioning of spatial learning and memory. Jiang et al. [51] observed impaired spatial learning and memory in animals along with significant decrease in the levels of glutamate and its metabotropic receptor (mGluR5) mRNA expression in the hippocampus and cortex of the animals exposed to arsenic.
The practice of exogenous supplementation of non-toxic, natural compounds with antioxidant property has gained momentum in recent years. Curcumin, a polyphenol compound has gained the importance as one of the most cited antioxidants due to diverse biological activities at cellular and molecular level. Curcumin is considered as an efficient neuroprotective drug based on its safety profile and pleiotropic actions along with its anti-inflammatory, antioxidant, anti-amyloid and anti-protein-aggregate activities [53].
The observations of the present study are suggestive of positive role of curcumin on cognitive deficits in animals receiving curcumin alone or curcumin+As2O3. Also, a significant decrease in the hippocampal GSH level in As2O3 alone exposed animals suggested arsenic-induced oxidative stress. The homeostasis of brain redox status is controlled by GSH to a large extent which helps in radical scavenging and protecting cells from oxidative stress. Increased generation of reactive oxygen species (ROS) and reaction nitrogen species are proposed as the underlying factors for inducing the toxicity by trivalent arsenicals (AsIII) and leading to increased oxidative stress [54, 55]. At cellular level, the increased ROS interact directly with the important biomolecules, leading to alterations in the gene expression via activation of various signal transduction pathways such as tyrosine phosphorylation system; transcription factors, including the activating protein-1 (AP-1) and nuclear factor κB (NF-κB); and mitogen-activated protein kinases like ERK1/2 [56, 57]. Also, As2O3 is reported to react with reduced GSH to form the transient molecule–GSH trioxide As(GS)3 [12]. This depletion of GSH levels is associated with an increase in GSSG concentration and decrease in GSH/glutathione disulfide (GSSG) ratio [58]. However, significant restoration in the GSH levels, evident in the animals receiving either curcumin (100 mg/kg bw) alone or along with As2O3 suggests the role of curcumin in combating the oxidative stress to a certain extent. The phenolic and β-diketone radical chain breaking property of curcumin is associated with the presence of two O-methoxy phenolic OH groups attached to the β-diketone moiety with methylene CH2 group, thereby allowing it to donate hydrogen atom from both OH and CH2 groups [59].
Our observations revealed significant reduction in neuronal density and neuronal area of pyramidal cells in CA1 of As2O3 alone treated animals. The pyramidal cells of CA1 subfield of hippocampus are reported to be highly susceptible to insults such as ischemia, toxins, etc. [60, 61] and oxidative stress [62]. However, the exact mechanism of SNV of these cells remains to be poorly understood though oxidative stress has been largely suggested as one of the major underlying factors [17].
The therapeutic activity of As2O3 in APL is based on its anti-proliferative action and induction of apoptosis [63]. Hippocampal neuronal apoptosis and other histopathological changes (neuronal shrinkage, chromatin condensation and margination along the inner nuclear membrane, and karyopyknosis) were also observed by Wang et al. [64] in mice exposed to different doses of arsenic (1 ppm, 2 ppm, and 4 ppm). Luo et al. [65] suggested the possible alteration in neurosignaling mechanisms as the basis for altered hippocampal morphology in rat offspring following arsenite exposure. Altered morphological features following inorganic arsenic exposure have been reported in other brain regions as well. Alterations in nuclear area measurements of Purkinje neurons in cerebellum [66] have been reported following exposure of rat pups to inorganic arsenic during postnatal period. Also, there are reports of structural alterations of myelinated tracts and inhibited neurite outgrowth in various in-vitro studies following arsenic exposure [67, 68].
Curcumin being lipophilic and hydrophobic, passes through the blood brain barrier and exerts its beneficial effects against various neurodegenerative disorders [69]. Protective effects of curcumin were suggested by Kim et al. [70], based on their study on mouse multi-potent neural progenitor cells and adult hippocampal neurogenesis. These investigators observed significant increase in the number of newly-generated cells in hippocampus and proliferation of neural stem cells in subventricular region of cerebral cortex of adult mice following curcumin administration, thereby suggestive of its role in neurogenesis. Wang et al. [71] also reported similar neuroprotective role of curcumin against neuronal death by creating an animal model of global cerebral ischemia. Also, the role of curcumin in inducing endogenous antioxidant defense mechanisms against neuronal injuries by activating certain transcription factors or pathways including Akt/Nrf2 pathway [72]; AP-1, NF-κB have been suggested [73].
In conclusion, the observations of the present study provide preliminary evidence of neurobehavioral deficits, altered morphology and down-regulation of antioxidant marker (GSH) in mouse hippocampus following chronic arsenic exposure. Supplementation of curcumin along with arsenic trioxide led to modification in cognitive functions and a significant up-regulation in hippocampal GSH. Hence, it could be proposed that, curcumin is a safe and cost-effective dietary adjuvant and its supplementation could hold a definitive promise in combating arsenic-induced adverse effects amongst populations inhabiting endemic areas.
Acknowledgements
The authors acknowledge the financial and technical support provided by the Department of Anatomy, All India Institute of Medical Sciences (AIIMS), New Delhi, India.
Notes
References
1. Vahidnia A, van der Voet GB, de Wolff FA. 2007; Arsenic neurotoxicity: a review. Hum Exp Toxicol. 26:823–32. DOI: 10.1177/0960327107084539. PMID: 18025055.
2. Agency for Toxic Substances and Disease Registry. 2007. Toxicological profile for arsenic. Public Health Service, Agency for Toxic Substances and Disease Registry;Atlanta, GA:
3. Brinkel J, Khan MH, Kraemer A. 2009; A systematic review of arsenic exposure and its social and mental health effects with special reference to Bangladesh. Int J Environ Res Public Health. 6:1609–19. DOI: 10.3390/ijerph6051609. PMID: 19543409. PMCID: PMC2697931.


4. Rao Y, Li R, Zhang D. 2013; A drug from poison: how the therapeutic effect of arsenic trioxide on acute promyelocytic leukemia was discovered. Sci China Life Sci. 56:495–502. DOI: 10.1007/s11427-013-4487-z. PMID: 23645104.


5. Iland HJ, Seymour JF. 2013; Role of arsenic trioxide in acute promyelocytic leukemia. Curr Treat Options Oncol. 14:170–84. DOI: 10.1007/s11864-012-0223-3. PMID: 23322117.


6. Park WH, Seol JG, Kim ES, Hyun JM, Jung CW, Lee CC, Kim BK, Lee YY. 2000; Arsenic trioxide-mediated growth inhibition in MC/CAR myeloma cells via cell cycle arrest in association with induction of cyclin-dependent kinase inhibitor, p21, and apoptosis. Cancer Res. 60:3065–71. PMID: 10850458.
7. Rojewski MT, Baldus C, Knauf W, Thiel E, Schrezenmeier H. 2002; Dual effects of arsenic trioxide (As2O3) on non-acute promyelocytic leukaemia myeloid cell lines: induction of apoptosis and inhibition of proliferation. Br J Haematol. 116:555–63. DOI: 10.1046/j.0007-1048.2001.03298.x. PMID: 11849211.


8. Hussein MA, Saleh M, Ravandi F, Mason J, Rifkin RM, Ellison R. 2004; Phase 2 study of arsenic trioxide in patients with relapsed or refractory multiple myeloma. Br J Haematol. 125:470–6. DOI: 10.1111/j.1365-2141.2004.04941.x. PMID: 15142117.


9. Wang L, Wang R, Fan L, Liang W, Liang K, Xu Y, Peng G, Ye Q. 2017; Arsenic trioxide is an immune adjuvant in liver cancer treatment. Mol Immunol. 81:118–26. DOI: 10.1016/j.molimm.2016.12.001. PMID: 27940255.


10. Novick SC. 2000; Arsenicals in hematologic cancers. Semin Oncol. 27:495–501.
11. Lengfelder E, Hofmann WK, Nowak D. 2012; Impact of arsenic trioxide in the treatment of acute promyelocytic leukemia. Leukemia. 26:433–42. DOI: 10.1038/leu.2011.245. PMID: 21904379.


12. Emadi A, Gore SD. 2010; Arsenic trioxide: an old drug rediscovered. Blood Rev. 24:191–9. DOI: 10.1016/j.blre.2010.04.001. PMID: 20471733.
13. Kritharis A, Bradley TP, Budman DR. 2013; The evolving use of arsenic in pharmacotherapy of malignant disease. Ann Hematol. 92:719–30. DOI: 10.1007/s00277-013-1707-3. PMID: 23494203.


14. Fanselow MS, Dong HW. 2010; Are the dorsal and ventral hippocampus functionally distinct structures? Neuron. 65:7–19. DOI: 10.1016/j.neuron.2009.11.031. PMID: 20152109. PMCID: PMC2822727.


15. Kosaki Y, Lin TC, Horne MR, Pearce JM, Gilroy KE. 2014; The role of the hippocampus in passive and active spatial learning. Hippocampus. 24:1633–52. DOI: 10.1002/hipo.22343. PMID: 25131441. PMCID: PMC4258078.


16. Wang Q, Sun AY, Simonyi A, Jensen MD, Shelat PB, Rottinghaus GE, MacDonald RS, Miller DK, Lubahn DE, Weisman GA, Sun GY. 2005; Neuroprotective mechanisms of curcumin against cerebral ischemia-induced neuronal apoptosis and behavioral deficits. J Neurosci Res. 82:138–48. DOI: 10.1002/jnr.20610. PMID: 16075466. PMCID: PMC5822585.


17. Wang X, Michaelis EK. 2010; Selective neuronal vulnerability to oxidative stress in the brain. Front Aging Neurosci. 2:12. DOI: 10.3389/fnagi.2010.00012. PMID: 20552050.


18. Griffin AL. 2015; Role of the thalamic nucleus reuniens in mediating interactions between the hippocampus and medial prefrontal cortex during spatial working memory. Front Syst Neurosci. 9:29. DOI: 10.3389/fnsys.2015.00029. PMID: 25805977. PMCID: PMC4354269.


19. Zhang NY, Qi M, Zhao L, Zhu MK, Guo J, Liu J, Gu CQ, Rajput SA, Krumm CS, Qi DS, Sun LH. 2016; Curcumin prevents aflatoxin B1 hepatoxicity by inhibition of cytochrome P450 isozymes in chick liver. Toxins (Basel). 8:E327. DOI: 10.3390/toxins8110327. PMID: 27834912.


20. Da Silva Morrone M, Schnorr CE, Behr GA, Gasparotto J, Bortolin RC, Moresco KS, Bittencourt L, Zanotto-Filho A, Gelain DP, Moreira JC. 2016; Oral administration of curcumin relieves behavioral alterations and oxidative stress in the frontal cortex, hippocampus, and striatum of ovariectomized Wistar rats. J Nutr Biochem. 32:181–8. DOI: 10.1016/j.jnutbio.2016.03.010. PMID: 27142750.


21. Sankar P, Telang AG, Kalaivanan R, Karunakaran V, Suresh S, Kesavan M. 2016; Oral nanoparticulate curcumin combating arsenic-induced oxidative damage in kidney and brain of rats. Toxicol Ind Health. 32:410–21. DOI: 10.1177/0748233713498455. PMID: 24105067.


22. Rajasekar N, Dwivedi S, Tota SK, Kamat PK, Hanif K, Nath C, Shukla R. 2013; Neuroprotective effect of curcumin on okadaic acid induced memory impairment in mice. Eur J Pharmacol. 715:381–94. DOI: 10.1016/j.ejphar.2013.04.033. PMID: 23685326.


23. Srivastava P, Yadav RS, Chandravanshi LP, Shukla RK, Dhuriya YK, Chauhan LK, Dwivedi HN, Pant AB, Khanna VK. 2014; Unraveling the mechanism of neuroprotection of curcumin in arsenic induced cholinergic dysfunctions in rats. Toxicol Appl Pharmacol. 279:428–40. DOI: 10.1016/j.taap.2014.06.006. PMID: 24952339.


24. Tatem KS, Quinn JL, Phadke A, Yu Q, Gordish-Dressman H, Nagaraju K. 2014; Gordish-Dressman H, Nagaraju K. Behavioral and locomotor measurements using an open field activity monitoring system for skeletal muscle diseases. J Vis Exp. (91):51785. DOI: 10.3791/51785. PMID: 25286313. PMCID: PMC4672952.


25. Walf AA, Frye CA. 2007; The use of the elevated plus maze as an assay of anxiety-related behavior in rodents. Nat Protoc. 2:322–8. DOI: 10.1038/nprot.2007.44. PMID: 17406592.


26. Morris R. 1984; Developments of a water-maze procedure for studying spatial learning in the rat. J Neurosci Methods. 11:47–60. DOI: 10.1016/0165-0270(84)90007-4. PMID: 6471907. PMCID: PMC2566857.


27. Vorhees CV, Williams MT. 2006; Morris water maze: procedures for assessing spatial and related forms of learning and memory. Nat Protoc. 1:848–58. DOI: 10.1038/nprot.2006.116. PMID: 17406317. PMCID: PMC2895266.


28. Ellman GL. 1959; Tissue sulfhydryl groups. Arch Biochem Biophys. 82:70–7. DOI: 10.1016/0003-9861(59)90090-6.


29. Paxinos G, Franklin KB. 2008. The mouse brain in stereotaxic coordinates. Academic Press;San Diego, CA:
30. Rhodes MC, Seidler FJ, Abdel-Rahman A, Tate CA, Nyska A, Rincavage HL, Slotkin TA. 2004; Terbutaline is a developmental neurotoxicant: effects on neuroproteins and morphology in cerebellum, hippocampus, and somatosensory cortex. J Pharmacol Exp Ther. 308:529–37. DOI: 10.1124/jpet.103.060095. PMID: 14610225.


31. Miki T, Harris SJ, Wilce PA, Takeuchi Y, Bedi KS. 2004; Effects of age and alcohol exposure during early life on pyramidal cell numbers in the CA1-CA3 region of the rat hippocampus. Hippocampus. 14:124–34. DOI: 10.1002/hipo.10155. PMID: 15058490.


32. Ferris NJ, Cragg BG. 1984; Organic lead and histological parameters of brain development. Acta Neuropathol. 63:306–12. DOI: 10.1007/BF00687338. PMID: 6475490. PMCID: PMC6590474.


33. Gundersen HJ. 1977; Notes on the estimation of the numerical density of arbitrary profiles: the edge effect. J Microsc. 111:219–23. DOI: 10.1111/j.1365-2818.1977.tb00062.x.


34. Isgor C, Kabbaj M, Akil H, Watson SJ. 2004; Delayed effects of chronic variable stress during peripubertal-juvenile period on hippocampal morphology and on cognitive and stress axis functions in rats. Hippocampus. 14:636–48. DOI: 10.1002/hipo.10207. PMID: 15301440.


35. Anacker C, Hen R. 2017; Adult hippocampal neurogenesis and cognitive flexibility: linking memory and mood. Nat Rev Neurosci. 18:335–46. DOI: 10.1038/nrn.2017.45. PMID: 28469276.
36. Evens AM, Tallman MS, Gartenhaus RB. 2004; The potential of arsenic trioxide in the treatment of malignant disease: past, present, and future. Leuk Res. 28:891–900. DOI: 10.1016/j.leukres.2004.01.011. PMID: 15234563.


37. Rust DM, Soignet SL. 2001; Risk/benefit profile of arsenic trioxide. Oncologist. 6 Suppl 2:29–32. DOI: 10.1634/theoncologist.6-suppl_2-29. PMID: 11331438.


38. Nachman KE, Baron PA, Raber G, Francesconi KA, Navas-Acien A, Love DC. 2013; Roxarsone, inorganic arsenic, and other arsenic species in chicken: a U.S.-based market basket sample. Environ Health Perspect. 121:818–24. DOI: 10.1289/ehp.1206245. PMID: 23694900. PMCID: PMC3701911.


39. Silbergeld EK, Nachman K. 2008; The environmental and public health risks associated with arsenical use in animal feeds. Ann N Y Acad Sci. 1140:346–57. DOI: 10.1196/annals.1454.049. PMID: 18991934. PMCID: PMC6941779.


40. Chang CY, Guo HR, Tsai WC, Yang KL, Lin LC, Cheng TJ, Chuu JJ. 2015; Subchronic arsenic exposure induces anxiety-like behaviors in normal mice and enhances depression-like behaviors in the chemically induced mouse model of depression. Biomed Res Int. 2015:159015. DOI: 10.1155/2015/159015. PMID: 26114099. PMCID: PMC4465655.


41. Bardullas U, Limón-Pacheco JH, Giordano M, Carrizales L, Mendoza-Trejo MS, Rodríguez VM. 2009; Chronic low-level arsenic exposure causes gender-specific alterations in locomotor activity, dopaminergic systems, and thioredoxin expression in mice. Toxicol Appl Pharmacol. 239:169–77. DOI: 10.1016/j.taap.2008.12.004. PMID: 19121333.


42. Rodríguez VM, Jiménez-Capdeville ME, Giordano M. 2003; The effects of arsenic exposure on the nervous system. Toxicol Lett. 145:1–18. DOI: 10.1016/S0378-4274(03)00262-5.


43. López Ruiz JR, Osuna Carrasco LP, López Valenzuela CL, Franco Rodríguez NE, de la Torre Valdovinos B, Jiménez Estrada I, Dueñas Jiménez JM, Dueñas Jiménez SH. 2015; The hippocampus participates in the control of locomotion speed. Neuroscience. 311:207–15. DOI: 10.1016/j.neuroscience.2015.10.034. PMID: 26597762. PMCID: PMC5376603.


44. Lu CB, Henderson Z. 2010; Nicotine induction of theta frequency oscillations in rodent hippocampus in vitro. Neuroscience. 166:84–93. DOI: 10.1016/j.neuroscience.2009.11.072. PMID: 20004706.
45. Fuhrmann F, Justus D, Sosulina L, Kaneko H, Beutel T, Friedrichs D, Schoch S, Schwarz MK, Fuhrmann M, Remy S. 2015; Locomotion, theta oscillations, and the speed-correlated firing of hippocampal neurons are controlled by a medial septal glutamatergic circuit. Neuron. 86:1253–64. DOI: 10.1016/j.neuron.2015.05.001. PMID: 25982367.


46. Cenci MA. 2007; Dopamine dysregulation of movement control in L-DOPA-induced dyskinesia. Trends Neurosci. 30:236–43. DOI: 10.1016/j.tins.2007.03.005. PMID: 17400300.


47. Naqvi F, Haider S, Batool Z, Perveen T, Haleem DJ. 2012; Sub-chronic exposure to noise affects locomotor activity and produces anxiogenic and depressive like behavior in rats. Pharmacol Rep. 64:64–9. DOI: 10.1016/S1734-1140(12)70731-4. PMID: 22580521.


48. Rosado JL, Ronquillo D, Kordas K, Rojas O, Alatorre J, Lopez P, Garcia-Vargas G, Del Carmen Caamaño M, Cebrián ME, Stoltzfus RJ. 2007; Arsenic exposure and cognitive performance in Mexican schoolchildren. Environ Health Perspect. 115:1371–5. DOI: 10.1289/ehp.9961. PMID: 17805430. PMCID: PMC1964916.


49. Tyler CR, Allan AM. 2014; The effects of arsenic exposure on neurological and cognitive dysfunction in human and rodent studies: a review. Curr Environ Health Rep. 1:132–47. DOI: 10.1007/s40572-014-0012-1. PMID: 24860722. PMCID: PMC4026128.


50. Adedayo AD, Aderinola AA, Adekilekun TA, Olaolu OO, Olanike AM, Olayemi IK. 2018; Morphine-alcohol treatment impairs cognitive functions and increases neuro-inflammatory responses in the medial prefrontal cortex of juvenile male rats. Anat Cell Biol. 51:41–51. DOI: 10.5115/acb.2018.51.1.41. PMID: 29644109. PMCID: PMC5890016.


51. Jiang S, Su J, Yao S, Zhang Y, Cao F, Wang F, Wang H, Li J, Xi S. 2014; Fluoride and arsenic exposure impairs learning and memory and decreases mGluR5 expression in the hippocampus and cortex in rats. PLoS One. 9:e96041. DOI: 10.1371/journal.pone.0096041. PMID: 24759735. PMCID: PMC3997496.


52. Ramos-Chávez LA, Rendón-López CR, Zepeda A, Silva-Adaya D, Del Razo LM, Gonsebatt ME. 2015; Neurological effects of inorganic arsenic exposure: altered cysteine/glutamate transport, NMDA expression and spatial memory impairment. Front Cell Neurosci. 9:21. DOI: 10.3389/fncel.2015.00021. PMID: 25709567. PMCID: PMC4321597.


53. Lucetti EC, Lucetti DL, da Silva Ribeiro AE, de Moura RB, Sampaio TM, de Almeida VL, Sérvula , da Silva AS, Bezerra LR, Neves KR, de Barros Viana GS. 2016; Curcumin reversion of neurochemical and immunohistochemical alterations in brain ischemia is related to its antioxidant and anti-inflammatory properties. J Med Plants Stud. 4:20–9.
54. Kitchin KT, Conolly R. 2010; Arsenic-induced carcinogenesis--oxidative stress as a possible mode of action and future research needs for more biologically based risk assessment. Chem Res Toxicol. 23:327–35. DOI: 10.1021/tx900343d. PMID: 20035570.


55. Tolins M, Ruchirawat M, Landrigan P. 2014; The developmental neurotoxicity of arsenic: cognitive and behavioral consequences of early life exposure. Ann Glob Health. 80:303–14. DOI: 10.1016/j.aogh.2014.09.005. PMID: 25459332.


56. Shi H, Shi X, Liu KJ. 2004; Oxidative mechanism of arsenic toxicity and carcinogenesis. Mol Cell Biochem. 255:67–78. DOI: 10.1023/B:MCBI.0000007262.26044.e8. PMID: 14971647.


57. Yen YP, Tsai KS, Chen YW, Huang CF, Yang RS, Liu SH. 2012; Arsenic induces apoptosis in myoblasts through a reactive oxygen species-induced endoplasmic reticulum stress and mitochondrial dysfunction pathway. Arch Toxicol. 86:923–33. DOI: 10.1007/s00204-012-0864-9. PMID: 22622864.


58. Flora SJ, Mittal M, Mehta A. 2008; Heavy metal induced oxidative stress & its possible reversal by chelation therapy. Indian J Med Res. 128:501–23.
59. Barzegar A, Moosavi-Movahedi AA. 2011; Intracellular ROS protection efficiency and free radical-scavenging activity of curcumin. PLoS One. 6:e26012. DOI: 10.1371/journal.pone.0026012. PMID: 22016801. PMCID: PMC3189944.


60. Bartsch T, Alfke K, Stingele R, Rohr A, Freitag-Wolf S, Jansen O, Deuschl G. 2006; Selective affection of hippocampal CA-1 neurons in patients with transient global amnesia without long-term sequelae. Brain. 129(Pt 11):2874–84. DOI: 10.1093/brain/awl248. PMID: 17003071.


61. Wang JY, Xia Q, Chu KT, Pan J, Sun LN, Zeng B, Zhu YJ, Wang Q, Wang K, Luo BY. 2011; Severe global cerebral ischemia-induced programmed necrosis of hippocampal CA1 neurons in rat is prevented by 3-methyladenine: a widely used inhibitor of autophagy. J Neuropathol Exp Neurol. 70:314–22. DOI: 10.1097/NEN.0b013e31821352bd. PMID: 21412169.


62. Wang X, Zaidi A, Pal R, Garrett AS, Braceras R, Chen XW, Michaelis ML, Michaelis EK. 2009; Genomic and biochemical approaches in the discovery of mechanisms for selective neuronal vulnerability to oxidative stress. BMC Neurosci. 10:12. DOI: 10.1186/1471-2202-10-12. PMID: 19228403. PMCID: PMC2677396.


63. Schipper HM, Lee JS, Singer J, Waxman S. 2002; Mechanisms of action of arsenic trioxide. Cancer Res. 62:3893–903. DOI: 10.5353/th_b3689998.
64. Wang Y, Bai C, Guan H, Chen R, Wang X, Wang B, Jin H, Piao F. 2015; Subchronic exposure to arsenic induces apoptosis in the hippocampus of the mouse brains through the Bcl-2/Bax pathway. J Occup Health. 57:212–21. DOI: 10.1539/joh.14-0226-OA. PMID: 25787108.


65. Luo J, Qiu Z, Chen J, Zhang L, Liu W, Tan Y, Shu W. 2013; Maternal and early life arsenite exposure impairs neurodevelopment and increases the expression of PSA-NCAM in hippocampus of rat offspring. Toxicology. 311:99–106. DOI: 10.1016/j.tox.2013.06.007. PMID: 23811142.


66. Dhar P, Mohari N, Mehra RD. 2007; Preliminary morphological and morphometric study of rat cerebellum following sodium arsenite exposure during rapid brain growth (RBG) period. Toxicology. 234:10–20. DOI: 10.1016/j.tox.2007.01.024. PMID: 17374429.


67. Frankel S, Concannon J, Brusky K, Pietrowicz E, Giorgianni S, Thompson WD, Currie DA. 2009; Arsenic exposure disrupts neurite growth and complexity in vitro. Neurotoxicology. 30:529–37. DOI: 10.1016/j.neuro.2009.02.015. PMID: 19635389.
68. Wang X, Meng D, Chang Q, Pan J, Zhang Z, Chen G, Ke Z, Luo J, Shi X. 2010; Arsenic inhibits neurite outgrowth by inhibiting the LKB1-AMPK signaling pathway. Environ Health Perspect. 118:627–34. DOI: 10.1289/ehp.0901510. PMID: 20439172. PMCID: PMC2866677.


69. Jia T, Sun Z, Lu Y, Gao J, Zou H, Xie F, Zhang G, Xu H, Sun D, Yu Y, Zhong Y. 2016; A dual brain-targeting curcumin-loaded polymersomes ameliorated cognitive dysfunction in intrahippocampal amyloid-β1-42-injected mice. Int J Nanomedicine. 11:3765–75. DOI: 10.2147/IJN.S94622. PMID: 27540290. PMCID: PMC4981163.
70. Kim SJ, Son TG, Park HR, Park M, Kim MS, Kim HS, Chung HY, Mattson MP, Lee J. 2008; Curcumin stimulates proliferation of embryonic neural progenitor cells and neurogenesis in the adult hippocampus. J Biol Chem. 283:14497–505. DOI: 10.1074/jbc.M708373200. PMID: 18362141. PMCID: PMC2386914.


71. Wang X, Pal R, Chen XW, Limpeanchob N, Kumar KN, Michaelis EK. 2005; High intrinsic oxidative stress may underlie selective vulnerability of the hippocampal CA1 region. Brain Res Mol Brain Res. 140:120–6. DOI: 10.1016/j.molbrainres.2005.07.018. PMID: 16137784.


72. Wu J, Li Q, Wang X, Yu S, Li L, Wu X, Chen Y, Zhao J, Zhao Y. 2013; Neuroprotection by curcumin in ischemic brain injury involves the Akt/Nrf2 pathway. PLoS One. 8:e59843. DOI: 10.1371/journal.pone.0059843. PMID: 23555802. PMCID: PMC3610879.


73. Cui Q, Li X, Zhu H. 2016; Curcumin ameliorates dopaminergic neuronal oxidative damage via activation of the Akt/Nrf2 pathway. Mol Med Rep. 13:1381–8. DOI: 10.3892/mmr.2015.4657. PMID: 26648392.


Fig. 1
Percentage center time (A) and total distance traveled (B) during open field test in control and experimental groups. Percentage open arm entries (C) and percentage open arm time (D) during elevated plus maze test in control and experimental groups. Mean escape latency (E) and mean distance traveled (F) during acquisition phase of Morris water maze (MWM) test in control and experimental groups. Time spent in platform quadrant (G) and number of platform crossings (H) during probe trial of MWM test in control and experimental groups. Values are presented as mean±SD. *P≤0.05, **P≤0.01, ***P≤0.001; ns, non-significant.
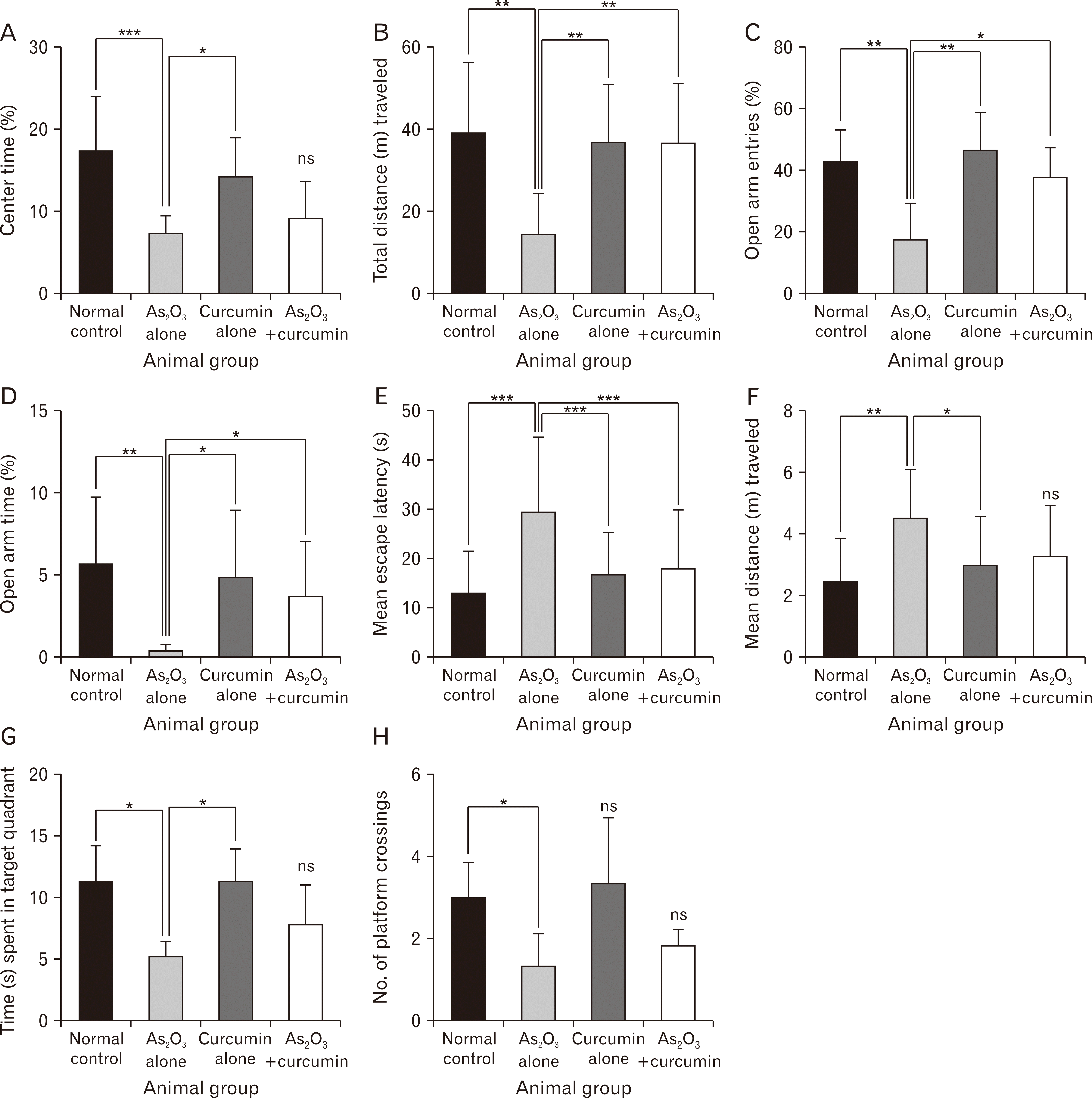
Fig. 2
Reduced glutathione (GSH) levels in mice hippocampus. Values are presented as mean±SD. *P≤0.05, **P≤0.01.
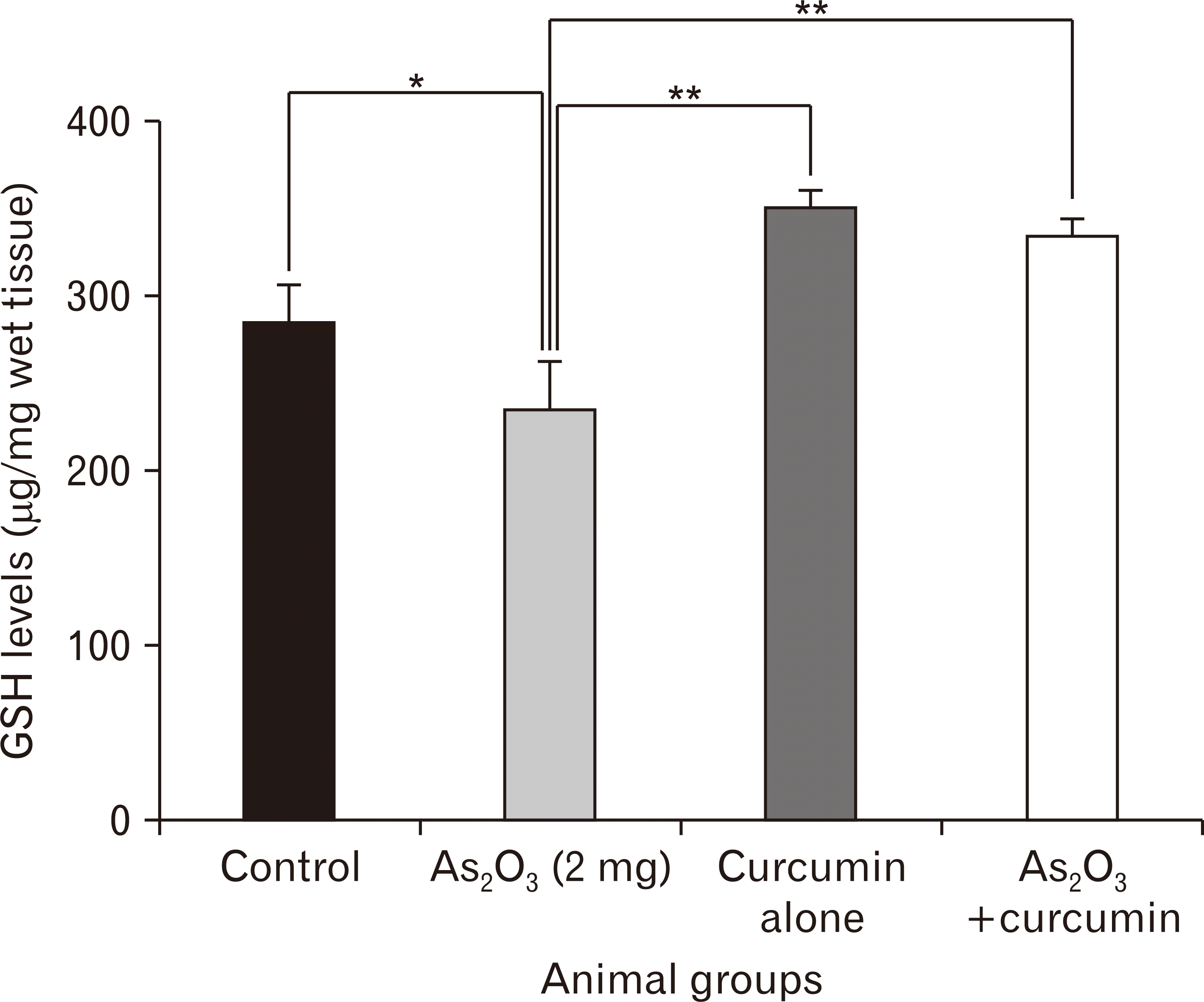
Fig. 3
Cresyl violet (CV) stained coronal sections of CA1 from control (A) and experimental animals (B, As2O3 alone; C, curcumin alone; D, As2O3+curcumin). Well-arranged 3–5 cell layer Stratum pyramidale (SP) with densely packed pyramidal neurons showing large/round-oval nucleus, marked by star and 1–3 distinct nucleoli marked by asterisk (A, C, D). (B) SP (1–3 cell layer thick, marked by vertical bar) shows disruption, evident by loosely packed pyramidal neurons, indicated by double head arrows with shrunken nuclei marked by oblique arrows. SO, Stratum oriens; SR, Stratum radiatum.
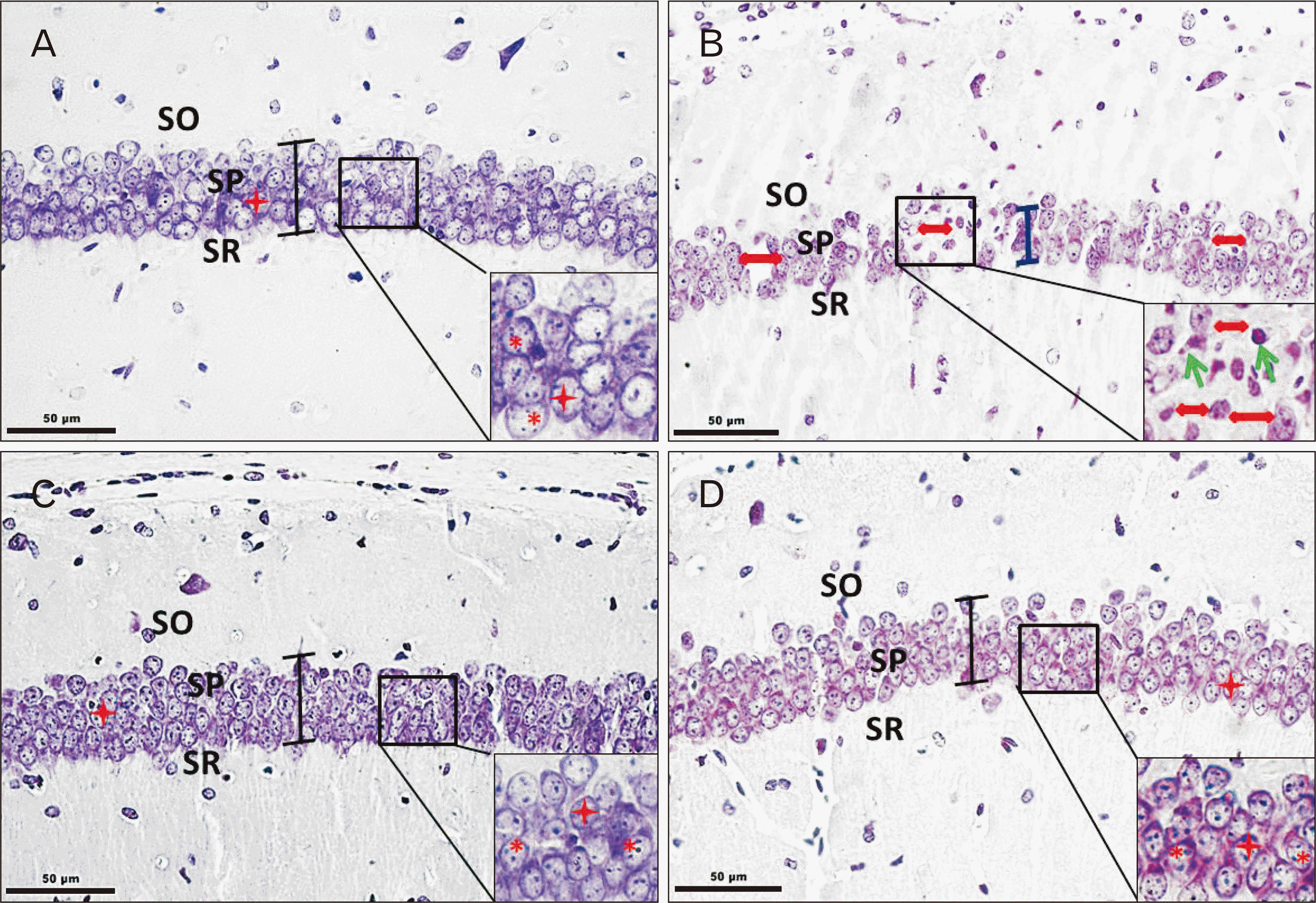
Table 1
Percentage change in body weight from day 1 to 45
Animal group | Body weight on day 1 | Body weight on day 45 | Change in body weight on day 45 | Brain weight (g) | Hippocampus weight (mg) | Encephalization quotient (EQ) | |
---|---|---|---|---|---|---|---|
|
|||||||
Percent change | P-value | ||||||
Normal control | 32.92±5.86 | 37.07±4.42 | 12.61±5.25 | ≤0.001a) | 0.312±0.011 | 33.67±12.93 | 0.0086±0.0005 |
As2O3 alone | 32.07±2.47 | 39.88±3.19 | 24.38±4.95 | - | 0.327±0.031 | 38.2±2.19 | 0.0088±0.0008 |
Curcumin alone | 32.18±6.2 | 37.2±3.42 | 15.59±4.80 | ≤0.001b) | 0.314±0.03 | 40.7±6.05 | 0.0085±0.0008 |
As2O3+curcumin | 32.15±2.09 | 38.62±2.79 | 20.11±4.80 | ≤0.001c) | 0.311±0.002 | 34.43±2.63 | 0.0085±0.0005 |
Table 2
Neuronal density (number of neurons/mm2), cell layer (SP) thickness (µm), and neuronal area (µm2) in CA1 of control and experimental animals
Animal group | Neuronal density (per μm2) | P-value | Cell layer (SP) thickness (µm) | P-value | Neuronal area (µm2) | P-value |
---|---|---|---|---|---|---|
Normal control | 93.95±13.71 | ≤0.05a) | 41.58±7.098 | ≤0.001a) | 78.21±21.19 | ≤0.001a) |
As2O3 alone | 74.81±16.81 | - | 29.65±5.281 | - | 54.74±10.55 | - |
Curcumin alone | 82.86±8.763 | ≤0.05b) | 37.74±1.768 | ≤0.001b) | 74.31±11.52 | ≤0.001b) |
As2O3+curcumin | 82.47±11.79 | >0.05c) | 37.36±2.552 | ≤0.001c) | 75.48±14.21 | ≤0.001c) |