Abstract
Autophagy is a highly conserved intracellular degradation and energy-recycling mechanism that contributes to the maintenance of cellular homeostasis. Extensive researches over the past decades have defined the role of autophagy innate immune cells. In this review, we describe the current state of knowledge regarding the role of autophagy in neutrophil biology and a picture of molecular mechanism underlying autophagy in neutrophils. Neutrophils are professional phagocytes that comprise the first line of defense against pathogen. Autophagy machineries are highly conserved in neutrophils. Autophagy is not only involved in generalized function of neutrophils such as differentiation in bone marrow but also plays crucial role effector functions of neutrophils such as granule formation, degranulation, neutrophil extracellular traps release, cytokine production, bactericidal activity and controlling inflammation. This review outlines the current understanding of autophagy in neutrophils and provides insight towards identification of novel therapeutics targeting autophagy in neutrophils.
Neutrophils are the most abundant circulating human leukocytes essential for protection against pathogens [1]. The bone marrow produces huge numbers of neutrophils, which are released into the circulation during steady-state and inflammatory conditions [2]. During infection, neutrophils are recruited to the site of infection and eliminate pathogens by means of various effector mechanisms, such as the degradation of ingested microbes by fusion of phagosomes with granule proteins, production of reactive oxygen species (ROS) [34567], and release of extracellular chromatin fibers decorated with antimicrobial proteins, termed neutrophil extracellular traps (NETs) [56]. Since these neutrophil effector mechanisms are not only protective but can also be destructive to host tissue, neutrophil production, trafficking, and clearance are tightly regulated by several homeostatic mechanisms in respective organs [7].
Autophagy, an intracellular degradation and energy-recycling mechanism, is one of the key homeostatic process contributing to neutrophil differentiation and function [89]. Mitroulis et al. [10] were the first to discover the evidence of autophagy in neutrophils. Autophagy in neutrophils is activated by phagocytosis of pathogens or activation of pattern recognition receptors (PRRs) by pathogen-derived toxins and molecules [10]. Since the initial discovery of autophagy in neutrophils, many studies have outlined the biological roles of autophagy in neutrophil activities, such as differentiation and effector functions. This review outlines the current understanding of autophagy in neutrophils. We first focus on known autophagy machinery in neutrophils and discuss the role of autophagy in neutrophil differentiation. Finally, we discuss the involvement of autophagy in neutrophil effector functions.
Autophagy is an essential mechanism in cell biology. Autophagy is a complex and tightly regulated pathway which allows cells to eliminate damaged or harmful intracellular components to maintain nutrient and energy homeostasis [11]. Hence, autophagy is an important survival mechanism for cells by protecting them from potential harms [12].
Autophagy molecular mechanisms involve the interaction of several conserved autophagy-related (Atg) proteins. Evolutionarily conserved canonical autophagy can be initiated by various stimuli, and results in the formation of a phagophore, whose membrane is derived from the rough endoplasmic reticulum [13141516]. Phagophore formation is initiated by either Atg/ULK1 complex (ULK 1/2-Atg13/FIP200-Atg101) or class III PI3K complex (Atg14-Beclin1/Ambra1-hVPS34/hVPS15), while mammalian target of rapamycin (mTOR) negatively regulates autophagy induction [131416171819]. The phagophore then elongates to form a double-membrane-enclosed autophagosome. During autophagosome formation, two key ubiquitin-like conjugation systems—the Atg12 conjugation system (Atg5-Atg12 in a multimeric complex with Atg16) and the LC3/Atg8 conjugation system (phosphatidylethanolamine [PE]-conjugated LC3-II, which forms after cleavage by Atg4)—are recruited by Atg7 to the autophagosomal membrane [172021]. Conjugated LC3 is integrated into the growing phagophore by the Atg5-Atg12 conjugation system, facilitating growth and elongation of the autophagosome. The Atg5-Atg12 conjugation system dissociates after autophagosome closure and maturation. Alternatively, there is another pathway, a non-canonical autophagy that involves bypass of proteins either in elongation and closure or in initiation in canonical pathway [22]. One example of non-canonical autophagy involves LC3II-associated phagocytosis (LAP). During LAP, a hybrid autophagic-phagocytic process, toll like receptor (TLR)-bound bacteria are taken up into the phagosome. These phagocytosed TLRs triggers the recruitment of LC3II to phagosomal membrane, thereby initiating autophagy and facilitates phagosome maturation [2223]. Then the mature autophagosome fuses with lysosomes. During this final degradative process, the autophagosome undergoes loss of its inner membrane and matures into an autolysosome. This process is initiated by the hVPS34-Beclin complex in association with hVPS38 and requires membrane fusion proteins such as LAMP2/Rab7/SNARES [1820].
Activation of neutrophil autophagic machinery is initiated by various triggers or stimuli, including phagocytosis-dependent and phagocytosis-independent signals [1016]. Such initiating signals induce PI3K activation, leading to enhanced NADPH oxidase (ROS) activity and decreased mTOR signaling [1016242526]. GPCR activation can initiate autophagy in neutrophils via ROS-dependent manner by interaction with high mobility group box 1 (HMGB1) and Beclin-1 [27]. However, macrophage-inducible Ca2+-dependent lectin receptor (Mincle) activation initiates autophagy in neutrophils independent of ROS and mTOR manner through activation of Beclin-1 nucleation complex [28]. Neutrophil nutrient starvation can also initiate autophagy via the AMPK pathway [2329]. Nucleation processes mediated by ULK (inhibition of mTOR) and/or Beclin-1 are followed by recruitment of other autophagy proteins to the phagosome, resulting in autophagosome formation [172021]. Ubiquitin-like conjugation system components Atg5 and Atg7 are key regulators of phagophore elongation and autophagosome formation [172021]. Atg7 induces LC3 lipidation, whereas the Atg12-Atg5-Atg16 complex integrates LC3-II into the phagophore and completes autophagosome formation. Knockdown of these genes inhibits autophagy in neutrophils [3031]. We have also previously reported that defective Atg5-Atg12 complex formation compromises phagophore elongation, resulting in defective autophagy [32]. In addition, p62/SQSTM is incorporated into the completed autophagosome and undergoes degradation in the autolysosome [33]. Increased p62/SQSTM degradation is strong indirect evidence that autophagy is occurring [33], and decreased p62/SQSTM1 degradation is associated with defective autophagy or inhibition of autophagy machinery [323435].
In summary, autophagy is initiated by various stimuli which either induce activation of Beclin-1 or inhibition of the mTOR pathway. These events then induce phagophore nucleation and elongation by the Atg5-Atg12 complex. Finally, autophagosome formation is completed by incorporation of lipidated LC3-II into the phagosome membrane, and p62/SQTM1 is degraded (Fig. 1). Similarly, activation or regulation of neutrophil autophagy follows autophagy machinery as discussed above. Neutrophil can sequester materials by mTOR-ULK1 dependent canonical autophagy or ATG5/ATG7 dependent non-canonical pathway with LC3 associated structures (LAP). While all stimuli which initiate autophagy in neutrophils up-regulate LC3 expression and lipidation, differential expression of ATG genes (ATG3, ATG4, ATG6 and ATG7) is observed depending on the stimuli [10]. Therefore, monitoring expression levels of Beclin-1 (Atg6), Atg5, Atg12, and p62/SQSTM1, along with LC3-II (LC3 expression and lipidation) can provide improved insights into the differential induction and regulation of autophagy machinery in neutrophils.
Neutrophils are generated from hematopoietic precursors in the bone marrow. Hematopoietic stem cells differentiate into common myeloid progenitor cells, which further differentiate into neutrophil precursors. These successive stages of neutrophil differentiation are governed by transcriptional programs [3637]. Although many reports discuss the role of autophagy in hematopoiesis and hematopoietic cell fate, its role in neutrophil differentiation has not been fully understood. Selective autophagy, macrophagy and mitophagy, differentially regulates the differentiation and maturation of myeloid lineage cells such as granulocytes, erythrocytes etc. [383940]. However, impaired autophagy in myeloid cells leads to hematopoietic diseases such as acute lymphoid leukemia [39]. Once committed to the granulocyte lineage, these progenitor cells pass through a mitotic pool composed of myeloblasts and promyelocytes. They further differentiate into myelocytes, metamyelocytes, band cells, and, finally, into mature segmented neutrophils [41]. There is increased autophagic activity in myleoblasts, promyleocytes and myleocytes, and declined as it differentiates into metamyleocytes and band cells. Autophagy is lowest in cells that had undergone terminal differentiation [3442]. Lipophagy, the autophagic ability to degrade fatty acid-enriched lipid droplets provides free fatty acids, the substrate, for oxidative phosphorylation to enhance ATP generation required for differentiation. Knockdown of either ATG5 or ATG7 results in defective neutrophil precursors differentiation into promyelocytes [42]. Such autophagy-defective neutrophil precursors exhibited an impaired lipophagy, mitochondrial respiration and ATP production, with a concomitant increase in glycolytic activity. As fatty acids represent an essential substrate for oxidative phosphorylation during the early stages of neutrophil differentiation, the resultant lack of ATP impairs differentiation during these stages [42]. However, tissue specific downregulation of ATG5 expression in immature neutrophils resulted in elevated proliferation of neutrophil precursor in bone marrow and an accelerated process of neutrophil differentiation. These ATG5 deficient neutrophils were without any abnormalities in morphology, granule protein content, apoptosis regulation and other effector functions. But, as a consequence of reduced autophagy (possibly mitophagy) the number of mitochondria were increased but did not affect the neutrophil morphology and function [34]. Moreover, over-expression of autophagy by overexpression of ATG5 or using pharmalogical approaches, delayed terminal neutrophil differentiation [34]. Overall, autophagy is upregulated in myeloblasts and progressively down-regulates as they continue to differentiate. These results highlight the important role of autophagy during early mitotic stage of neutrophil differentiation and possibly due to short life span, active autophagy is not important for post-mitotic stage of neutrophil differentiation. Altogether, autophagy appears to reciprocally regulate neutrophil differentiation in mitotic and post-mitotic pools.
Neutrophils are professional phagocytes and are thus able to phagocytose and destroy infectious agents [43]. During phagocytosis, pathogens become sequestered in highly-organized endocytic compartments known as phagosomes, and are ultimately degraded via fusion of these compartments to lysosomes [44]. During phagocytosis by neutrophil, bacteria is engulfed into phagosomes. Fusion of granule contents and NADPH complex to phagosome contribute to its maturation and antimicrobial activity [45]. Recent studies indicate neutrophils exhibits phagocytosis-dependent or non-canonical selective autophagy during bactericidal activity [264647]. During phagocytosis dependent LAP, PRR-bound bacteria in phagosome induces LC3 translocation, thereby initiating autophagy. LAP facilitates phagosome maturation and consequent bacteria degradation [23]. Phagocytosisindependent autophagy (or non-canonical selective autophagy, xenophagy) also exists, and is initiated by ubiquitinated p62/SQSTM1 bound to intracellular bacteria or bacteria which have escaped the phagocytic pathway. Such autophagy-mediated bacterial clearance is rapid and occurs independently of ROS generation [48]. Furthermore, augmentation of autophagy greatly enhanced neutrophil phagocytosis of bacteria and its bactericidal activity, facilitating effective elimination of both drug-sensitive and multi drug-resistant bacterial strains [234950]. In contrast, impaired or inhibited autophagy enhanced survival and growth of bacteria within neutrophils [235152]. These findings indicate the important contribution of non-canonical selective autophagy to the bactericidal activity of human neutrophils and possibly the defect with these processes rather than canonical pathway may hinder bacterial clearance.
Entrapment and killing of pathogens is also mediated by NETosis (a type of cell death culminating in NETs release), first discovered by Brinkmann et al. [5] as a novel extracellular microbicidal mechanism employed by neutrophils. NETs are composed of dense extracellular chromatin studded with antimicrobial proteins (e.g., histones, neutrophil-derived granule proteins, and other cytosolic proteins) [5354]. During NETosis, chromatin becomes decondensed, followed by nuclear swelling, nuclear membrane disintegration, rupture of the cell membrane, and release of NETs into the extracellular space. Released NETs are eventually phagocytosed and eliminated from circulation by macrophages [55].
Various stimuli induce neutrophil NETs formation, including pathogen associated molecular patterns (e.g., Staphylococcus aureus, Candida albicans, Leishmania spp., and other bacteria and fungi), exogenous compounds (e.g., phorbol myristate acetate, calcium ionophores, and potassium ionophores), danger associated molecular patterns (e.g., interleukin [IL]-33, immune complexes, auto-antibodies, histones, LL-37, and HMGB1), inflammatory cytokines (e.g., tumor necrosis factor-α and IL-8), platelets, and antibodies [565758596061]. Different stimuli induce activation of differential signaling pathways which nonetheless converge on NETosis [566062].
Remijsen et al. [63] reported that both autophagy and ROS are required for NETosis. They demonstrated that both autophagy and ROS generation are required for chromatin decondensation: defects in either pathway result in apoptotic cell death rather than NETosis [63]. Although this suggests that the ROS-autophagy axis is essential for NETosis, autophagy can be induced in both ROS-dependent and ROS-independent manners [63]. Calcium ionophore induces rapid, NADPH oxidase-independent NETosis [64], and NETosis induced by certain bacteria is known to be NADPH oxidase-independent [656667]. Interestingly, Itakura and McCarty described regulation of autophagy and cytoskeletal remodeling by the mTOR pathway during NETs formation [16]. Inhibition of mTOR or autophagy activation by rapamycin enhanced NETs formation, whereas autophagy inhibition by wortmannin (a PI3K inhibitor) diminished NETs release [16]. Since autophagy is reciprocally regulated by the mTOR pathway, this study indicates that ROS-independent NETosis is mediated by mTOR-autophagy signaling. Moreover, evidence is accumulating that autophagy is an important regulator of NETs formation. Chargui et al. [23] observed that autophagy preceded NETs formation in neutrophils infected with invasive Escherichia coli, and that Atg5 silencing completely blocked NETs formation. Pharmacological inhibition of autophagy (using wortmannin, 3-MA, bafilomycin, chloroquine, or atrazine) or inhibition of autophagy via siRNA-mediated ATG7 silencing both inhibited NETs release [2832616869]. Neutrophils isolated from aged mice possessing a defect in ATG5 (and thus in autophagic pathways) exhibited decreased NETs release [30]. Additionally, Mincle knockdown attenuated autophagy, concomitant with impaired NETs formation [28]. We have previously observed that impaired autophagy in neutrophils results in decreased NETosis during sepsis [32]. Furthermore, pharmacological induction of autophagy in neutrophils (using rapamycin or tamoxifen) rescued NETs formation [2832]. However, Germic et al. [70] reported that neutrophils isolated from ATG5 -knockout mice still retained the capacity for NETs release. Furthermore, only PI3K inhibition attenuated NETs formation, while inhibition of downstream autophagy pathways (using bafilomycin or chloroquine) did not.
Further, autophagy and NETosis are closely related with various pathologies, both in experimental disease models and in the clinical conditions [31717273]. Autophagy positively regulates NETosis, and impaired autophagy thus correlates with reduced NETs formation [283263]. Decreased NETosis and resultant prolonged neutrophil survival increases mortality among sepsis patients [74]. Furthermore, NETs depletion resulted in a delayed immune response and polymicrobial sepsis in mice [75]. We previously demonstrated that neutrophils isolated from patients experiencing fatal sepsis exhibit decreased NETs formation. Additionally, decreased NETs formation correlated with impaired autophagy, and autophagy augmentation protected mice from sepsis in a NETs-dependent manner [33]. Furthermore, clarithromycin, a macrolide antibiotic, induced autophagy-dependent formation of NETs decorated with the antimicrobial peptide LL-37. Clarithromycin-induced NETs formation inhibited growth and biofilm formation of Acinetobacter baumannii [76]. Tamoxifen, an autophagy inducer, restored impaired autophagy and NETs formation in Mincle-defective neutrophils and enhanced neutrophil phagocytosis of Klebsiella pneumoniae, limiting bacterial pneumonia [77]. One primary function of NETs is sequestration of inflammatory sites from circulation to prevent pathogen spreading. However, tumor-infiltrating neutrophils also exhibited increased autophagy and NETs formation. Such NETs may physically sequester cancer cells, interfering with the anti-tumoral activities of effector T cells [7879]. Moreover, cancer cells exhibit increased metastatic rates in a NETs-enriched environment [808182]. These studies showed autophagy is important but not essential for NETosis. However, as discussed above intervention of both canonical and non-canonical autophagy with molecular or pharmacological interventions impacts NETsosis. Dissecting among the ATG-dependent autophagy, ATG-independent autophagy and ATG-dependent non-autophagic pathway during NETosis may further clarify the importance of autophagy during NETosis.
Neutrophils possess various cytoplasmic granules: azurophil (primary) granules, specific (secondary) granules, gelatinase-containing tertiary granules, and secretory vesicles (phosphasomes) [838485]. A recent study also described a fourth (ficolin-1-rich) neutrophil granule [71]. Granule heterogeneity is explained by a targeting-by-time hypothesis: granules are formed and stored in a time-dependent order which corresponds to neutrophil developmental stages. Thus, granule formation occurs throughout neutrophil development, beginning with azurophil granules (in myeloblasts-promyelocytes) and ending with secretory vesicles (in band cells) [718384]. Different transcription factors regulate expression of different granule proteins [71]. Recent studies have demonstrated the involvement of autophagy in granule formation. Knockdown of ATG7 correlated with decreased expression of the transcription factor C/EBPε (involved in myeloid differentiation and granule maturation), leading to defective neutrophil granule maturation and fewer neutrophil granules with diminished content [42]. Such neutrophils exhibited increased primary and secondary granule protein expression, but decreased tertiary granule protein expression. Since tertiary granules are formed during the band cell stage, while primary and secondary granules are continually produced and degraded throughout neutrophil differentiation [72738486], it is speculated that autophagy might be involved in regulation of the relevant transcription program governing granule protein formation prior to the band cell stage.
Autophagy is also involved in neutrophil degranulation. Activated neutrophils release their granule contents into the phagosome and/or extracellular space via exocytosis, in a process known as degranulation [87]. Degranulation occurs in an order opposite to that of granule formation, with secretory vesicle contents being released first and azurophil granule contents being released last [738384]. Knockdown of ATG5 and ATG7 led to impaired ROS generation and impaired degranulation, reducing inflammatory function of neutrophils [31]. Autophagy deficiency diminished neutrophil degranulation (primary and secondary granules), with the exception of myeloperoxidase (MPO) [31]. Since the total intracellular quantity of neutrophil MPO remained consistent regardless of Atg knockdown [4283], the above phenomenon is likely due to storage impairment of MPO in Atg-knockdown neutrophils. These studies suggest a role for both conventional secretion and selective autophagy in neutrophil granule production, storage, and content release. Further dissecting the molecular signatures of autophagic process may facilitates understanding the specific process associated with neutrophil granule formation and degranulation.
Autophagy is also involved in the production and release of certain neutrophil cytokines. Neutrophils produce pro- and anti-inflammatory cytokines either spontaneously or in response to stimulation [88899091]. The pro-inflammatory cytokine IL-1β is one of the most extensively studied neutrophil-derived cytokine [929394]. Autophagy is involved in the release of IL-1β by human neutrophils in an ATG-dependent secretory pathway. Neutrophil release of IL-1β was inhibited by autophagy inhibitors or ATG5 silencing [94]. This is particularly interesting given that autophagy degrades and blocks maturation and secretion of IL-1β in other immune cells such as macrophages [9596].
Autophagy is involved in modulation of inflammation through regulating pathogen clearance, antigen presentation, cytokine generation, and immune responses [97]. Abnormalities in autophagic machinery have been linked to several diseases, such as Crohn's disease, cystic fibrosis, and inflammation-associated metabolic disease [98]. As we have reviewed, autophagy has also been established as a regulator of neutrophil function [31]. Excessive or dysregulated neutrophil functions has been noted in various diseases such as sepsis, chronic obstructive pulmonary disease (COPD), chronic granulomatous disease, Crohn's disease, and familial Mediterranean fever (FMF) [99100101]. Recently, the role of autophagy in neutrophil-mediated inflammation and autoimmune disease has been established [31]. Inhibition of autophagy during neutrophil-mediated inflammation and autoimmune disease reduced disease severity and progression by suppressing degranulation and ROS production [2335]. Similarly, suppression of autophagy through NLRP3 knockdown or inhibition of the NLRP3 inflammasome enhanced neutrophil recruitment and phagocytosis, thereby improving bacterial clearance and augmenting the survival of septic mice [102]. Furthermore, during the pathogenesis of COPD, autophagy induction increased neutrophil apoptosis and accelerated disease development via platelet-activating factor receptor (PAFR) [27]. Inhibition of PAFR-dependent autophagy reversed autophagic neutrophil death, and slowed disease development [27]. In contrast, autophagy defects in myeloid cells attenuated neutrophilic differentiation of acute promyelocytic leukemia cells and lowered the efficacy of all-trans retinoic acid therapy [103104105]. Inhibiting autophagy in myeloid cells also resulted in spontaneous lung inflammation and enhanced susceptibility to endotoxemia, an effect attributed to increased levels of IL-8 [106]. Similarly, adherent-invasive E. coli (AIEC)-infected neutrophils exhibited a defect in the degradation stage of autophagy (delayed or limited autolysosome maturation), enhancing inflammation via autophagic neutrophil death and increased IL-18 secretion [23]. Further, neutrophil ATG5 knockdown induced dysregulated function, increasing the susceptibility to M. tuberculosis infection [35]. In contrast, autophagy activation through serum starvation or rapamycin treatment of neutrophils enhanced their bactericidal activity against AIEC [23]. Autophagy augmentation in neutrophils by intravenous immunoglobulin treatment also enhanced bactericidal activity against multi-drug resistant strains [49]. However, increased autophagy in neutrophils is associated with IL-β-mediated inflammatory responses during active ulcerative colitis and FMF [9293107]. This is attributed to the increased load of IL-1β in autophagydriven NETs. Increased IL-1β-induced REDD1 (regulated in development and DNA damage response 1) expression enhances autophagy in neutrophils, leading to increased formation of NETs loaded with IL-1β [9293107].
These studies suggest a dual role for autophagy in neutrophil function during inflammation (Fig. 2). Augmentation of autophagy may be an effective target for enhancement of proper myeloid differentiation and antimicrobial defense. Conversely, autophagy inhibition may be useful in neutrophil-mediated inflammatory disease. Broadly, autophagy is important for neutrophil differentiation and function. However, one important question is whether these pathological outcomes are mediated by canonical or non-canonical autophagic processes. This implies that understanding the molecular mechanism of autophagy during cell physiology and pathological condition would further clarify role of specific autophagy during infection and inflammation.
Autophagy is an important phenomenon involved in sequestering cytoplasmic materials and pathogens. Autophagy in neutrophil is associated with its differentiation (lipophagy) during early stage. Autophagy is associated with regulation of degranulation and cytokine production (secretory autophagy), bactericidal activity (xenophagy and LAP) and NETs release. Depending on the inflammatory environment, autophagy in neutrophil may behave as a double-edged sword. While it is beneficial in combating infection, it may favor excessive inflammation through exaggerated NETs formation and cytokine release. Thus, Autophagic homeostasis is important for proper neutrophil effector function and host health. Learning to distinguish between canonical and non-canonical autophagic process could add a significant contribution to understanding autophagy regulation of neutrophil's fate and function. This essential insight may also contribute towards appropriate development of novel pharmacological therapies targeting autophagy in neutrophil-mediated diseases.
ACKNOWLEDGEMENTS
This study was supported by 2017R1C1B2009015, 2017R1A4A1015652 and 2018R1D1A1B07050421 from National Research Foundation of Korea (NRF).
Notes
References
1. Nathan C. Neutrophils and immunity: challenges and opportunities. Nat Rev Immunol. 2006; 6:173–182. PMID: 16498448.


2. Furze RC, Rankin SM. Neutrophil mobilization and clearance in the bone marrow. Immunology. 2008; 125:281–288. PMID: 19128361.


3. Hampton MB, Kettle AJ, Winterbourn CC. Inside the neutrophil phagosome: oxidants, myeloperoxidase, and bacterial killing. Blood. 1998; 92:3007–3017. PMID: 9787133.


5. Brinkmann V, Reichard U, Goosmann C, Fauler B, Uhlemann Y, Weiss DS, Weinrauch Y, Zychlinsky A. Neutrophil extracellular traps kill bacteria. Science. 2004; 303:1532–1535. PMID: 15001782.


6. Naegele M, Tillack K, Reinhardt S, Schippling S, Martin R, Sospedra M. Neutrophils in multiple sclerosis are characterized by a primed phenotype. J Neuroimmunol. 2012; 242:60–71. PMID: 22169406.


8. Ryter SW, Cloonan SM, Choi AM. Autophagy: a critical regulator of cellular metabolism and homeostasis. Mol Cells. 2013; 36:7–16. PMID: 23708729.


9. Nezis IP, Vaccaro MI, Devenish RJ, Juhász G. Autophagy in development, cell differentiation, and homeodynamics: from molecular mechanisms to diseases and pathophysiology. Biomed Res Int. 2014; 2014:349623. PMID: 25247173.


10. Mitroulis I, Kourtzelis I, Kambas K, Rafail S, Chrysanthopoulou A, Speletas M, Ritis K. Regulation of the autophagic machinery in human neutrophils. Eur J Immunol. 2010; 40:1461–1472. PMID: 20162553.


11. Yang Z, Klionsky DJ. An overview of the molecular mechanism of autophagy. Curr Top Microbiol Immunol. 2009; 335:1–32. PMID: 19802558.


12. Das G, Shravage BV, Baehrecke EH. Regulation and function of autophagy during cell survival and cell death. Cold Spring Harb Perspect Biol. 2012; 4:a008813. PMID: 22661635.


14. Wang CW, Klionsky DJ. The molecular mechanism of autophagy. Mol Med. 2003; 9:65–76. PMID: 12865942.


16. Itakura A, McCarty OJ. Pivotal role for the mTOR pathway in the formation of neutrophil extracellular traps via regulation of autophagy. Am J Physiol Cell Physiol. 2013; 305:C348–C354. PMID: 23720022.


17. Parzych KR, Klionsky DJ. An overview of autophagy: morphology, mechanism, and regulation. Antioxid Redox Signal. 2014; 20:460–473. PMID: 23725295.


18. Backer JM. The intricate regulation and complex functions of the Class III phosphoinositide 3-kinase Vps34. Biochem J. 2016; 473:2251–2271. PMID: 27470591.


19. Mizushima N, Noda T, Yoshimori T, Tanaka Y, Ishii T, George MD, Klionsky DJ, Ohsumi M, Ohsumi Y. A protein conjugation system essential for autophagy. Nature. 1998; 395:395–398. PMID: 9759731.


20. Glick D, Barth S, Macleod KF. Autophagy: cellular and molecular mechanisms. J Pathol. 2010; 221:3–12. PMID: 20225336.


21. Ichimura Y, Kirisako T, Takao T, Satomi Y, Shimonishi Y, Ishihara N, Mizushima N, Tanida I, Kominami E, Ohsumi M, Noda T, Ohsumi Y. A ubiquitin-like system mediates protein lipidation. Nature. 2000; 408:488–492. PMID: 11100732.


22. Codogno P, Mehrpour M, Proikas-Cezanne T. Canonical and non-canonical autophagy: variations on a common theme of self-eating? Nat Rev Mol Cell Biol. 2011; 13:7–12. PMID: 22166994.


23. Chargui A, Cesaro A, Mimouna S, Fareh M, Brest P, Naquet P, Darfeuille-Michaud A, Hébuterne X, Mograbi B, Vouret-Craviari V, Hofman P. Subversion of autophagy in adherent invasive Escherichia coli-infected neutrophils induces inflammation and cell death. PLoS One. 2012; 7:e51727. PMID: 23272151.


24. Goldmann O, Medina E. The expanding world of extracellular traps: not only neutrophils but much more. Front Immunol. 2013; 3:420. PMID: 23335924.


25. Mihalache CC, Yousefi S, Conus S, Villiger PM, Schneider EM, Simon HU. Inflammation-associated autophagy-related programmed necrotic death of human neutrophils characterized by organelle fusion events. J Immunol. 2011; 186:6532–6542. PMID: 21515790.


26. Huang J, Canadien V, Lam GY, Steinberg BE, Dinauer MC, Magalhaes MA, Glogauer M, Grinstein S, Brumell JH. Activation of antibacterial autophagy by NADPH oxidases. Proc Natl Acad Sci U S A. 2009; 106:6226–6231. PMID: 19339495.


27. Lv XX, Liu SS, Li K, Cui B, Liu C, Hu ZW. Cigarette smoke promotes COPD by activating platelet-activating factor receptor and inducing neutrophil autophagic death in mice. Oncotarget. 2017; 8:74720–74735. PMID: 29088819.


28. Sharma A, Simonson TJ, Jondle CN, Mishra BB, Sharma J. Mincle-mediated neutrophil extracellular trap formation by regulation of autophagy. J Infect Dis. 2017; 215:1040–1048. PMID: 28186242.


29. Zhao M, Klionsky DJ. AMPK-dependent phosphorylation of ULK1 induces autophagy. Cell Metab. 2011; 13:119–120. PMID: 21284977.


30. Xu F, Zhang C, Zou Z, Fan EKY, Chen L, Li Y, Billiar TR, Wilson MA, Shi X, Fan J. Aging-related Atg5 defect impairs neutrophil extracellular traps formation. Immunology. 2017; 151:417–432. PMID: 28375544.


31. Bhattacharya A, Wei Q, Shin JN, Abdel Fattah E, Bonilla DL, Xiang Q, Eissa NT. Autophagy Is required for neutrophil-mediated inflammation. Cell Rep. 2015; 12:1731–1739. PMID: 26344765.


32. Park SY, Shrestha S, Youn YJ, Kim JK, Kim SY, Kim HJ, Park SH, Ahn WG, Kim S, Lee MG, Jung KS, Park YB, Mo EK, Ko Y, Lee SY, Koh Y, Park MJ, Song DK, Hong CW. Autophagy primes neutrophils for neutrophil extracellular trap formation during sepsis. Am J Respir Crit Care Med. 2017; 196:577–589. PMID: 28358992.


33. Klionsky DJ, Abdelmohsen K, Abe A, Abedin MJ, Abeliovich H, Acevedo Arozena A, Adachi H, Adams CM, Adams PD, Adeli K, Adhihetty PJ, Adler SG, Agam G, Agarwal R, Aghi MK, Agnello M, Agostinis P, Aguilar PV, Aguirre-Ghiso J, Airoldi EM, et al. Guidelines for the use and interpretation of assays for monitoring autophagy (3rd edition). Autophagy. 2016; 12:1–222. PMID: 26799652.
34. Rožman S, Yousefi S, Oberson K, Kaufmann T, Benarafa C, Simon HU. The generation of neutrophils in the bone marrow is controlled by autophagy. Cell Death Differ. 2015; 22:445–456. PMID: 25323583.


35. Kimmey JM, Huynh JP, Weiss LA, Park S, Kambal A, Debnath J, Virgin HW, Stallings CL. Unique role for ATG5 in neutrophil-mediated immunopathology during M. tuberculosis infection. Nature. 2015; 528:565–569. PMID: 26649827.


36. Theilgaard-Mönch K, Jacobsen LC, Borup R, Rasmussen T, Bjerregaard MD, Nielsen FC, Cowland JB, Borregaard N. The transcriptional program of terminal granulocytic differentiation. Blood. 2005; 105:1785–1796. PMID: 15514007.
37. Akashi K, He X, Chen J, Iwasaki H, Niu C, Steenhard B, Zhang J, Haug J, Li L. Transcriptional accessibility for genes of multiple tissues and hematopoietic lineages is hierarchically controlled during early hematopoiesis. Blood. 2003; 101:383–389. PMID: 12393558.


38. Huang Y, Tan P, Wang X, Yi Y, Hu Y, Wang D, Wang F. Transcriptomic insights into temporal expression pattern of autophagy genes during monocytic and granulocytic differentiation. Autophagy. 2018; 14:558–559. PMID: 29313406.


39. Koschade SE, Brandts CH. Selective autophagy in normal and malignant hematopoiesis. J Mol Biol. 2019; DOI: 10.1016/j.jmb.2019.06.025. [Epub ahead of print].


40. Orsini M, Chateauvieux S, Rhim J, Gaigneaux A, Cheillan D, Christov C, Dicato M, Morceau F, Diederich M. Sphingolipid-mediated inflammatory signaling leading to autophagy inhibition converts erythropoiesis to myelopoiesis in human hematopoietic stem/progenitor cells. Cell Death Differ. 2019; 26:1796–1812. PMID: 30546074.


41. da Silva FM, Massart-Leën AM, Burvenich C. Development and maturation of neutrophils. Vet Q. 1994; 16:220–225. PMID: 7740747.


42. Riffelmacher T, Clarke A, Richter FC, Stranks A, Pandey S, Danielli S, Hublitz P, Yu Z, Johnson E, Schwerd T, McCullagh J, Uhlig H, Jacobsen SEW, Simon AK. Autophagy-dependent generation of free fatty acids is critical for normal neutrophil differentiation. Immunity. 2017; 47:466–480.e5. PMID: 28916263.


43. Kantari C, Pederzoli-Ribeil M, Witko-Sarsat V. The role of neutrophils and monocytes in innate immunity. Contrib Microbiol. 2008; 15:118–146. PMID: 18511859.


44. Wong CO, Gregory S, Hu H, Chao Y, Sepúlveda VE, He Y, Li-Kroeger D, Goldman WE, Bellen HJ, Venkatachalam K. Lysosomal degradation is required for sustained phagocytosis of bacteria by macrophages. Cell Host Microbe. 2017; 21:719–730.e6. PMID: 28579255.


45. Lee WL, Harrison RE, Grinstein S. Phagocytosis by neutrophils. Microbes Infect. 2003; 5:1299–1306. PMID: 14613773.


46. Chargui A, El May MV. Autophagy mediates neutrophil responses to bacterial infection. APMIS. 2014; 122:1047–1058. PMID: 24735202.


47. Gong L, Cullinane M, Treerat P, Ramm G, Prescott M, Adler B, Boyce JD, Devenish RJ. The Burkholderia pseudomallei type III secretion system and BopA are required for evasion of LC3-associated phagocytosis. PLoS One. 2011; 6:e17852. PMID: 21412437.


48. Ramachandran G, Gade P, Tsai P, Lu W, Kalvakolanu DV, Rosen GM, Cross AS. Potential role of autophagy in the bactericidal activity of human PMNs for Bacillus anthracis. Pathog Dis. 2015; 73:ftv080. PMID: 26424808.
49. Itoh H, Matsuo H, Kitamura N, Yamamoto S, Higuchi T, Takematsu H, Kamikubo Y, Kondo T, Yamashita K, Sasada M, Takaori-Kondo A, Adachi S. Enhancement of neutrophil autophagy by an IVIG preparation against multidrug-resistant bacteria as well as drug-sensitive strains. J Leukoc Biol. 2015; 98:107–117. PMID: 25908735.


50. Ullah I, Ritchie ND, Evans TJ. The interrelationship between phagocytosis, autophagy and formation of neutrophil extracellular traps following infection of human neutrophils by Streptococcus pneumoniae. Innate Immun. 2017; 23:413–423. PMID: 28399692.


51. Wang C, Mendonsa GR, Symington JW, Zhang Q, Cadwell K, Virgin HW, Mysorekar IU. Atg16L1 deficiency confers protection from uropathogenic Escherichia coli infection in vivo. Proc Natl Acad Sci U S A. 2012; 109:11008–11013. PMID: 22715292.


52. Rinchai D, Riyapa D, Buddhisa S, Utispan K, Titball RW, Stevens MP, Stevens JM, Ogawa M, Tanida I, Koike M, Uchiyama Y, Ato M, Lertmemongkolchai G. Macroautophagy is essential for killing of intracellular Burkholderia pseudomallei in human neutrophils. Autophagy. 2015; 11:748–755. PMID: 25996656.
53. Remijsen Q, Kuijpers TW, Wirawan E, Lippens S, Vandenabeele P, Vanden Berghe T. Dying for a cause: NETosis, mechanisms behind an antimicrobial cell death modality. Cell Death Differ. 2011; 18:581–588. PMID: 21293492.


54. Urban CF, Ermert D, Schmid M, Abu-Abed U, Goosmann C, Nacken W, Brinkmann V, Jungblut PR, Zychlinsky A. Neutrophil extracellular traps contain calprotectin, a cytosolic protein complex involved in host defense against Candida albicans. PLoS Pathog. 2009; 5:e1000639. PMID: 19876394.


55. Boe DM, Curtis BJ, Chen MM, Ippolito JA, Kovacs EJ. Extracellular traps and macrophages: new roles for the versatile phagocyte. J Leukoc Biol. 2015; 97:1023–1035. PMID: 25877927.


56. Kenny EF, Herzig A, Krüger R, Muth A, Mondal S, Thompson PR, Brinkmann V, Bernuth HV, Zychlinsky A. Diverse stimuli engage different neutrophil extracellular trap pathways. Elife. 2017; 6:e24437. PMID: 28574339.


57. Kaplan MJ, Radic M. Neutrophil extracellular traps: double-edged swords of innate immunity. J Immunol. 2012; 189:2689–2695. PMID: 22956760.


58. Peng HH, Liu YJ, Ojcius DM, Lee CM, Chen RH, Huang PR, Martel J, Young JD. Mineral particles stimulate innate immunity through neutrophil extracellular traps containing HMGB1. Sci Rep. 2017; 7:16628. PMID: 29192209.


59. Neumann A, Papareddy P, Westman J, Hyldegaard O, Snäll J, Norrby-Teglund A, Herwald H. Immunoregulation of neutrophil extracellular trap formation by endothelial-derived p33 (gC1q receptor). J Innate Immun. 2018; 10:30–43. PMID: 29035880.


60. van der Linden M, Westerlaken GHA, van der Vlist M, van Montfrans J, Meyaard L. Differential signalling and kinetics of neutrophil extracellular trap release revealed by quantitative live imaging. Sci Rep. 2017; 7:6529. PMID: 28747804.


61. Maugeri N, Campana L, Gavina M, Covino C, De Metrio M, Panciroli C, Maiuri L, Maseri A, D'Angelo A, Bianchi ME, Rovere-Querini P, Manfredi AA. Activated platelets present high mobility group box 1 to neutrophils, inducing autophagy and promoting the extrusion of neutrophil extracellular traps. J Thromb Haemost. 2014; 12:2074–2088. PMID: 25163512.


62. Chatfield SM, Grebe K, Whitehead LW, Rogers KL, Nebl T, Murphy JM, Wicks IP. Monosodium urate crystals generate nuclease-resistant neutrophil extracellular traps via a distinct molecular pathway. J Immunol. 2018; 200:1802–1816. PMID: 29367211.


63. Remijsen Q, Vanden Berghe T, Wirawan E, Asselbergh B, Parthoens E, De Rycke R, Noppen S, Delforge M, Willems J, Vandenabeele P. Neutrophil extracellular trap cell death requires both autophagy and superoxide generation. Cell Res. 2011; 21:290–304. PMID: 21060338.


64. Douda DN, Khan MA, Grasemann H, Palaniyar N. SK3 channel and mitochondrial ROS mediate NADPH oxidase-independent NETosis induced by calcium influx. Proc Natl Acad Sci U S A. 2015; 112:2817–2822. PMID: 25730848.


65. Pilsczek FH, Salina D, Poon KK, Fahey C, Yipp BG, Sibley CD, Robbins SM, Green FH, Surette MG, Sugai M, Bowden MG, Hussain M, Zhang K, Kubes P. A novel mechanism of rapid nuclear neutrophil extracellular trap formation in response to Staphylococcus aureus. J Immunol. 2010; 185:7413–7425. PMID: 21098229.
66. Chow OA, von Köckritz-Blickwede M, Bright AT, Hensler ME, Zinkernagel AS, Cogen AL, Gallo RL, Monestier M, Wang Y, Glass CK, Nizet V. Statins enhance formation of phagocyte extracellular traps. Cell Host Microbe. 2010; 8:445–454. PMID: 21075355.


67. Byrd AS, O'Brien XM, Johnson CM, Lavigne LM, Reichner JS. An extracellular matrix-based mechanism of rapid neutrophil extracellular trap formation in response to Candida albicans. J Immunol. 2013; 190:4136–4148. PMID: 23509360.
68. Stranks AJ, Hansen AL, Panse I, Mortensen M, Ferguson DJ, Puleston DJ, Shenderov K, Watson AS, Veldhoen M, Phadwal K, Cerundolo V, Simon AK. Autophagy controls acquisition of aging features in macrophages. J Innate Immun. 2015; 7:375–391. PMID: 25764971.


69. Ma R, Li T, Cao M, Si Y, Wu X, Zhao L, Yao Z, Zhang Y, Fang S, Deng R, Novakovic VA, Bi Y, Kou J, Yu B, Yang S, Wang J, Zhou J, Shi J. Extracellular DNA traps released by acute promyelocytic leukemia cells through autophagy. Cell Death Dis. 2016; 7:e2283. PMID: 27362801.


70. Germic N, Stojkov D, Oberson K, Yousefi S, Simon HU. Neither eosinophils nor neutrophils require ATG5-dependent autophagy for extracellular DNA trap formation. Immunology. 2017; 152:517–525. PMID: 28703297.


71. Rørvig S, Østergaard O, Heegaard NH, Borregaard N. Proteome profiling of human neutrophil granule subsets, secretory vesicles, and cell membrane: correlation with transcriptome profiling of neutrophil precursors. J Leukoc Biol. 2013; 94:711–721. PMID: 23650620.


72. Masson PL, Heremans JF, Schonne E. Lactoferrin, an iron-binding protein in neutrophilic leukocytes. J Exp Med. 1969; 130:643–658. PMID: 4979954.
73. Faurschou M, Borregaard N. Neutrophil granules and secretory vesicles ininflammation. Microbes Infect. 2003; 5:1317–1327. PMID: 14613775.
74. Patel JM, Sapey E, Parekh D, Scott A, Dosanjh D, Gao F, Thickett DR. Sepsis Induces a Dysregulated neutrophil phenotype that is associated with increased mortality. Mediators Inflamm. 2018; 2018:4065362. PMID: 29849488.


75. Meng W, Paunel-Görgülü A, Flohé S, Hoffmann A, Witte I, MacKenzie C, Baldus SE, Windolf J, Lögters TT. Depletion of neutrophil extracellular traps in vivo results in hypersusceptibility to polymicrobial sepsis in mice. Crit Care. 2012; 16:R137. PMID: 22835277.


76. Konstantinidis T, Kambas K, Mitsios A, Panopoulou M, Tsironidou V, Dellaporta E, Kouklakis G, Arampatzioglou A, Angelidou I, Mitroulis I, Skendros P, Ritis K. Immunomodulatory role of clarithromycin in acinetobacter baumannii infection via formation of neutrophil extracellular traps. Antimicrob Agents Chemother. 2015; 60:1040–1048. PMID: 26643338.


77. Sharma A, Steichen AL, Jondle CN, Mishra BB, Sharma J. Protective role of Mincle in bacterial pneumonia by regulation of neutrophil mediated phagocytosis and extracellular trap formation. J Infect Dis. 2014; 209:1837–1846. PMID: 24353272.


78. Li XF, Chen DP, Ouyang FZ, Chen MM, Wu Y, Kuang DM, Zheng L. Increased autophagy sustains the survival and pro-tumourigenic effects of neutrophils in human hepatocellular carcinoma. J Hepatol. 2015; 62:131–139. PMID: 25152203.


79. Park J, Wysocki RW, Amoozgar Z, Maiorino L, Fein MR, Jorns J, Schott AF, Kinugasa-Katayama Y, Lee Y, Won NH, Nakasone ES, Hearn SA, Küttner V, Qiu J, Almeida AS, Perurena N, Kessenbrock K, Goldberg MS, Egeblad M. Cancer cells induce metastasis-supporting neutrophil extracellular DNA traps. Sci Transl Med. 2016; 8:361ra138.


80. Tohme S, Yazdani HO, Al-Khafaji AB, Chidi AP, Loughran P, Mowen K, Wang Y, Simmons RL, Huang H, Tsung A. Neutrophil extracellular traps promote the development and progression of liver metastases after surgical stress. Cancer Res. 2016; 76:1367–1380. PMID: 26759232.


81. Najmeh S, Cools-Lartigue J, Rayes RF, Gowing S, Vourtzoumis P, Bourdeau F, Giannias B, Berube J, Rousseau S, Ferri LE, Spicer JD. Neutrophil extracellular traps sequester circulating tumor cells via β1-integrin mediated interactions. Int J Cancer. 2017; 140:2321–2330. PMID: 28177522.


82. Leach J, Morton JP, Sansom OJ. Neutrophils: Homing in on the myeloid mechanisms of metastasis. Mol Immunol. 2019; 110:69–76. PMID: 29269005.


83. Borregaard N, Cowland JB. Granules of the human neutrophilic polymorphonuclear leukocyte. Blood. 1997; 89:3503–3521. PMID: 9160655.


84. Cowland JB, Borregaard N. Granulopoiesis and granules of human neutrophils. Immunol Rev. 2016; 273:11–28. PMID: 27558325.


85. Lawrence SM, Corriden R, Nizet V. The ontogeny of a neutrophil: mechanisms of granulopoiesis and homeostasis. Microbiol Mol Biol Rev. 2018; 82:e00057–e00017. PMID: 29436479.


86. Gullberg U, Andersson E, Garwicz D, Lindmark A, Olsson I. Biosynthesis, processing and sorting of neutrophil proteins: insight into neutrophil granule development. Eur J Haematol. 1997; 58:137–153. PMID: 9150707.


87. Lacy P. Mechanisms of degranulation in neutrophils. Allergy Asthma Clin Immunol. 2006; 2:98–108. PMID: 20525154.


88. Mantovani A, Cassatella MA, Costantini C, Jaillon S. Neutrophils in the activation and regulation of innate and adaptive immunity. Nat Rev Immunol. 2011; 11:519–531. PMID: 21785456.


89. Tecchio C, Cassatella MA. Neutrophil-derived cytokines involved in physiological and pathological angiogenesis. Chem Immunol Allergy. 2014; 99:123–137. PMID: 24217606.


90. Tecchio C, Micheletti A, Cassatella MA. Neutrophil-derived cytokines: facts beyond expression. Front Immunol. 2014; 5:508. PMID: 25374568.


91. Naegelen I, Beaume N, Plançon S, Schenten V, Tschirhart EJ, Bréchard S. Regulation of neutrophil degranulation and cytokine secretion: a novel model approach based on linear fitting. J Immunol Res. 2015; 2015:817038. PMID: 26579547.


92. Angelidou I, Chrysanthopoulou A, Mitsios A, Arelaki S, Arampatzioglou A, Kambas K, Ritis D, Tsironidou V, Moschos I, Dalla V, Stakos D, Kouklakis G, Mitroulis I, Ritis K, Skendros P. REDD1/ autophagy pathway is associated with Neutrophil-Driven IL-1β inflammatory response in active ulcerative colitis. J Immunol. 2018; 200:3950–3961. PMID: 29712770.
93. Apostolidou E, Skendros P, Kambas K, Mitroulis I, Konstantinidis T, Chrysanthopoulou A, Nakos K, Tsironidou V, Koffa M, Boumpas DT, Ritis K. Neutrophil extracellular traps regulate IL-1β-mediated inflammation in familial Mediterranean fever. Ann Rheum Dis. 2016; 75:269–277. PMID: 25261578.


94. Iula L, Keitelman IA, Sabbione F, Fuentes F, Guzman M, Galletti JG, Gerber PP, Ostrowski M, Geffner JR, Jancic CC, Trevani AS. Autophagy mediates interleukin-1β secretion in human neutrophils. Front Immunol. 2018; 9:269. PMID: 29515581.


96. Harris J, Hartman M, Roche C, Zeng SG, O'Shea A, Sharp FA, Lambe EM, Creagh EM, Golenbock DT, Tschopp J, Kornfeld H, Fitzgerald KA, Lavelle EC. Autophagy controls IL-1beta secretion by targeting pro-IL-1beta for degradation. J Biol Chem. 2011; 286:9587–9597. PMID: 21228274.
97. Netea-Maier RT, Plantinga TS, van de Veerdonk FL, Smit JW, Netea MG. Modulation of inflammation by autophagy: consequences for human disease. Autophagy. 2016; 12:245–260. PMID: 26222012.


98. Levine B, Mizushima N, Virgin HW. Autophagy in immunity and inflammation. Nature. 2011; 469:323–335. PMID: 21248839.


99. Zhang F, Liu AL, Gao S, Ma S, Guo SB. Neutrophil dysfunction in sepsis. Chin Med J (Engl). 2016; 129:2741–2744. PMID: 27824008.


100. Segal AW, Loewi G. Neutrophil dysfunction in Crohn's disease. Lancet. 1976; 2:219–221. PMID: 59239.


101. Davis WC, Douglas SD, Fudenberg HH. A selective neutrophil dysfunction syndrome: impaired killing of staphylococci. Ann Intern Med. 1968; 69:1237–1243. PMID: 5725737.


102. Jin L, Batra S, Jeyaseelan S. Deletion of Nlrp3 augments survival during polymicrobial sepsis by decreasing autophagy and enhancing phagocytosis. J Immunol. 2017; 198:1253–1262. PMID: 28031338.
103. Haimovici A, Brigger D, Torbett BE, Fey MF, Tschan MP. Induction of the autophagy-associated gene MAP1S via PU.1 supports APL differentiation. Leuk Res. 2014; 38:1041–1047. PMID: 25043887.


104. Schläfli AM, Shan-Krauer D, Garattini E, Tschan MP. Regulation and function of autophagy in retinoic acid mediated therapy of myeloid leukemia and breast cancer. Ital J Anat Embryol. 2015; 120:9–10.
105. Britschgi A, Yousefi S, Laedrach J, Shan D, Simon H-U, Tobler A, Fey MF, Tschan MP. Blocking the autophagy gene 5 (ATG5) impairs ATRA-induced myeloid differentiation, and ATG5 is downregulated in AML. Blood. 2008; 112:309.


106. Abdel Fattah E, Bhattacharya A, Herron A, Safdar Z, Eissa NT. Critical role for IL-18 in spontaneous lung inflammation caused by autophagy deficiency. J Immunol. 2015; 194:5407–5416. PMID: 25888640.


107. Skendros P, Chrysanthopoulou A, Rousset F, Kambas K, Arampatzioglou A, Mitsios A, Bocly V, Konstantinidis T, Pellet P, Angelidou I, Apostolidou E, Ritis D, Tsironidou V, Galtsidis S, Papagoras C, Stakos D, Kouklakis G, Dalla V, Koffa M, Mitroulis I, et al. Regulated in development and DNA damage responses 1 (REDD1) links stress with IL-1β-mediated familial Mediterranean fever attack through autophagy-driven neutrophil extracellular traps. J Allergy Clin Immunol. 2017; 140:1378–1387.e13. PMID: 28342915.


Fig. 1
Regulation of autophagy in neutrophils.
Phagocytosis-independent autophagy in neutrophils is initiated by starvation or activation of receptor by different stimuli. This induces either Beclin-1 activation or mTOR inhibition via increased ROS, PI3K, and/or AMPK activity. Activation of Beclin-1 or mTOR inhibition induces phagophore nucleation and elongation by the Atg5-Atg12 complex. Finally, autophagosome maturation is completed via incorporation of lipidated LC3-II into the phagosome membrane, and the ubiquitin protein p62/SQTM1 is degraded. mTOR, mammalian target of rapamycin; ROS, reactive oxygen species; TLR, toll like receptor.
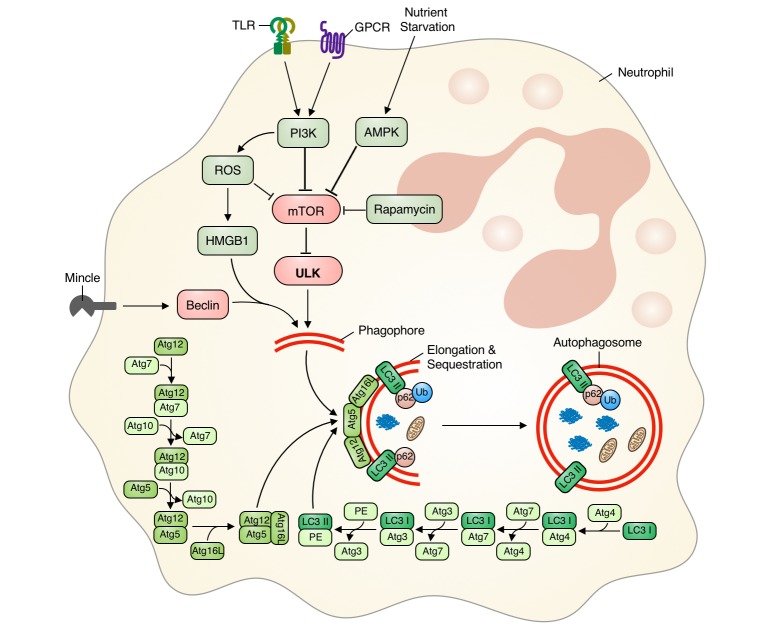
Fig. 2
Contribution of autophagy to neutrophil function.
Autophagy is involved in neutrophil differentiation and granule formation. It enhances neutrophil phagocytosis, neutrophil extracellular traps (NETs) formation, degranulation, and cytokine release. Autophagy in neutrophils also differentially modulates the outcomes of inflammation and infection in a context-dependent manner.
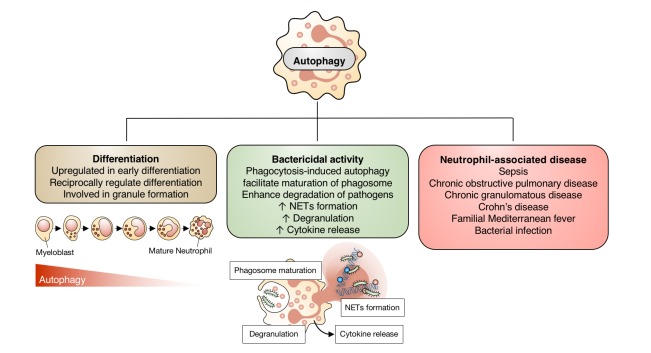