Abstract
Purpose
The purpose of this study is to compare the performance of the T1 3D subtraction technique and the conventional 2D dynamic contrast enhancement (DCE) technique in diagnosing Cushing's disease.
Materials and Methods
Twelve patients with clinically and biochemically proven Cushing's disease were included in the study. In addition, 23 patients with a Rathke's cleft cyst (RCC) diagnosed on an MRI with normal pituitary hormone levels were included as a control, to prevent non-blinded positive results. Postcontrast T1 3D fast spin echo (FSE) images were acquired after DCE images in 3T MRI and image subtraction of pre- and postcontrast T1 3D FSE images were performed. Inter-observer agreement, interpretation time, multiobserver receiver operating characteristic (ROC), and net benefit analyses were performed to compare 2D DCE and T1 3D subtraction techniques.
Results
Inter-observer agreement for a visual scale of contrast enhancement was poor in DCE (κ = 0.57) and good in T1 3D subtraction images (κ = 0.75). The time taken for determining contrast-enhancement in pituitary lesions was significantly shorter in the T1 3D subtraction images compared to the DCE sequence (P < 0.05). ROC values demonstrated increased reader confidence range with T1 3D subtraction images (95% confidence interval [CI]: 0.94–1.00) compared with DCE (95% CI: 0.70–0.92) (P < 0.01). The net benefit effect of T1 3D subtraction images over DCE was 0.34 (95% CI: 0.12–0.56). For Cushing's disease, both reviewers misclassified one case as a nonenhancing lesion on the DCE images, while no cases were misclassified on T1 3D subtraction images.
References
1. Juszczak A, Grossman A. The management of Cushing's disease – from investigation to treatment. Endokrynol Pol. 2013; 64:166–174.
2. Newell-Price J, Trainer P, Besser M, Grossman A. The diagnosis and differential diagnosis of Cushing's syndrome and pseudo-Cushing's states. Endocr Rev. 1998; 19:647–672.


3. Potts MB, Shah JK, Molinaro AM, et al. Cavernous and inferior petrosal sinus sampling and dynamic magnetic resonance imaging in the preoperative evaluation of Cushing's disease. J Neurooncol. 2014; 116:593–600.


4. Witek P, Zielinski G. Predictive value of preoperative magnetic resonance imaging of the pituitary for surgical cure in Cushing's disease. Turk Neurosurg. 2012; 22:747–752.


5. Davis WL, Lee JN, King BD, Harnsberger HR. Dynamic contrast-enhanced MR imaging of the pituitary gland with fast spin-echo technique. J Magn Reson Imaging. 1994; 4:509–511.


6. Bartynski WS, Lin L. Dynamic and conventional spin-echo MR of pituitary microlesions. AJNR Am J Neuroradiol. 1997; 18:965–972.
7. Ludecke DK, Flitsch J, Knappe UJ, Saeger W. Cushing's disease: a surgical view. J Neurooncol. 2001; 54:151–166.
8. Busse RF, Brau AC, Vu A, et al. Effects of refocusing flip angle modulation and view ordering in 3D fast spin echo. Magn Reson Med. 2008; 60:640–649.


9. Kato Y, Higano S, Tamura H, et al. Usefulness of contrast-enhanced T1-weighted sampling perfection with application-optimized contrasts by using different flip angle evolutions in detection of small brain metastasis at 3T MR imaging: comparison with magnetization-prepared rapid acquisition of gradient echo imaging. AJNR Am J Neuroradiol. 2009; 30:923–929.


10. Kitajima M, Hirai T, Shigematsu Y, et al. Comparison of 3D FLAIR, 2D FLAIR, and 2D T2-weighted MR imaging of brain stem anatomy. AJNR Am J Neuroradiol. 2012; 33:922–927.


11. Lien RJ, Corcuera-Solano I, Pawha PS, Naidich TP, Tanenbaum LN. Three-tesla imaging of the pituitary and parasellar region: T1-weighted 3-dimensional fast spin echo cube outperforms conventional 2-dimensional magnetic resonance imaging. J Comput Assist Tomogr. 2015; 39:329–333.
12. Wolfsberger S, Ba-Ssalamah A, Pinker K, et al. Application of three-tesla magnetic resonance imaging for diagnosis and surgery of sellar lesions. J Neurosurg. 2004; 100:278–286.


13. Pinker K, Ba-Ssalamah A, Wolfsberger S, Mlynarik V, Knosp E, Trattnig S. The value of high-field MRI (3T) in the assessment of sellar lesions. Eur J Radiol. 2005; 54:327–334.


14. de Rotte AA, Groenewegen A, Rutgers DR, et al. High resolution pituitary gland MRI at 7.0 tesla: a clinical evaluation in Cushing's disease. Eur Radiol. 2016; 26:271–277.


15. Patronas N, Bulakbasi N, Stratakis CA, et al. Spoiled gradient recalled acquisition in the steady state technique is superior to conventional postcontrast spin echo technique for magnetic resonance imaging detection of adrenocorticotropin-secreting pituitary tumors. J Clin Endocrinol Metab. 2003; 88:1565–1569.


16. Stobo DB, Lindsay RS, Connell JM, Dunn L, Forbes KP. Initial experience of 3 tesla versus conventional field strength magnetic resonance imaging of small functioning pituitary tumours. Clin Endocrinol (Oxf). 2011; 75:673–677.


17. Lee HB, Kim ST, Kim HJ, et al. Usefulness of the dynamic gadolinium-enhanced magnetic resonance imaging with simultaneous acquisition of coronal and sagittal planes for detection of pituitary microadenomas. Eur Radiol. 2012; 22:514–518.


18. Takano S, Akutsu H, Hara T, Yamamoto T, Matsumura A. Correlations of vascular architecture and angiogenesis with pituitary adenoma histotype. Int J Endocrinol. 2014; 2014:989574.


19. Secil M, Obuz F, Altay C, et al. The role of dynamic subtraction MRI in detection of hepatocellular carcinoma. Diagn Interv Radiol. 2008; 14:200–204.
20. Yu JS, Kim YH, Rofsky NM. Dynamic subtraction magnetic resonance imaging of cirrhotic liver: assessment of high signal intensity lesions on nonenhanced T1-weighted images. J Comput Assist Tomogr. 2005; 29:51–58.
21. Tay KL, Yang JL, Phal PM, Lim BG, Pascoe DM, Stella DL. Assessing signal intensity change on well-registered images: comparing subtraction, color-encoded subtraction, and parallel display formats. Radiology. 2011; 260:400–407.


22. Yu JS, Rofsky NM. Dynamic subtraction MR imaging of the liver: advantages and pitfalls. AJR Am J Roentgenol. 2003; 180:1351–1357.


23. Sundarakumar DK, Wilson GJ, Osman SF, Zaidi SF, Maki JH. Evaluation of image registration in subtracted 3D dynamic contrast-enhanced MRI of treated hepatocellular carcinoma. AJR Am J Roentgenol. 2015; 204:287–296.


24. Chenevert TL, Malyarenko DI, Newitt D, et al. Errors in quantitative image analysis due to platform-dependent image scaling. Transl Oncol. 2014; 7:65–71.


25. Feldmar J, Ayache N. Rigid, affine and locally affine registation of free-form surfaces. Int J Comput Vis. 1996; 18:99–119.
26. Harrigan CJ, Peters DC, Gibson CM, et al. Hypertrophic cardiomyopathy: quantification of late gadolinium enhancement with contrast-enhanced cardiovascular MR imaging. Radiology. 2011; 258:128–133.


27. Saade C, El-Merhi F, Mayat A, Brennan PC, Yousem D. Comparison of standard and quadruple-phase contrast material injection for artifacts, image quality, and radiation dose in the evaluation of head and neck cancer metastases. Radiology. 2016; 279:571–577.


28. Halligan S, Altman DG, Mallett S. Disadvantages of using the area under the receiver operating characteristic curve to assess imaging tests: a discussion and proposal for an alternative approach. Eur Radiol. 2015; 25:932–939.


29. Jagannathan J, Dumont A, Jane JA Jr. Diagnosis and management of pediatric sellar lesions. In Laws ER Jr, Sheehan JP, eds. Pituitary surgery – a modern approach. Front Horm Res. Basel: Karger. 2006. 83–104.
30. Jagannathan J, Dumont AS, Jane JA Jr, Laws ER Jr. Pediatric sellar tumors: diagnostic procedures and management. Neurosurg Focus. 2005; 18:E6.


31. Ikeda H, Abe T, Watanabe K. Usefulness of composite methionine-positron emission tomography/3.0-tesla magnetic resonance imaging to detect the localization and extent of early-stage Cushing adenoma. J Neurosurg. 2010; 112:750–755.


32. Colao A, Boscaro M, Ferone D, Casanueva FF. Managing Cushing's disease: the state of the art. Endocrine. 2014; 47:9–20.


33. Elster AD. High-resolution, dynamic pituitary MR imaging: standard of care or academic pastime? AJR Am J Roentgenol. 1994; 163:680–682.


34. Kucharczyk W, Bishop JE, Plewes DB, Keller MA, George S. Detection of pituitary microadenomas: comparison of dynamic keyhole fast spin-echo, unenhanced, and conventional contrast-enhanced MR imaging. AJR Am J Roentgenol. 1994; 163:671–679.


35. Rossi Espagnet MC, Bangiyev L, Haber M, et al. High-Resolution DCE-MRI of the pituitary gland using radial k-space acquisition with compressed sensing reconstruction. AJNR Am J Neuroradiol. 2015; 36:1444–1449.
36. Kartal MG, Algin O. Evaluation of hydrocephalus and other cerebrospinal fluid disorders with MRI: An update. Insights Imaging. 2014; 5:531–541.


37. Wang J, Wu Y, Yao Z, Yang Z. Assessment of pituitary microlesions using 3D sampling perfection with application-optimized contrasts using different flip-angle evolutions. Neuroradiology. 2014; 56:1047–1053.


38. Fritz J, Fritz B, Thawait GG, Meyer H, Gilson WD, Raithel E. Three-dimensional CAIPIRINHA SPACE TSE for 5-minute high-resolution MRI of the knee. Invest Radiol. 2016; 51:609–617.


39. Fritz J, Ahlawat S, Demehri S, et al. Compressed sensing SEMAC: 8-fold accelerated high resolution metal artifact reduction MRI of cobalt-chromium knee arthroplasty implants. Invest Radiol. 2016; 51:666–676.


40. Schulze M, Reimann K, Seeger A, Klose U, Ernemann U, Hauser TK. Improvement in imaging common temporal bone pathologies at 3 T MRI: small structures benefit from a small field of view. Clin Radiol. 2017; 72:267 e261–267 e212.


41. Seeger A, Schulze M, Schuettauf F, Klose U, Ernemann U, Hauser TK. Feasibility and evaluation of dual-source transmit 3D imaging of the orbits: comparison to high-resolution conventional MRI at 3T. Eur J Radiol. 2015; 84:1150–1158.


Fig. 1.
Images of a 37-year-old female patient diagnosed with Cushing disease. On conventional dynamic contrast enhanced images (a), both reviewers recorded the visual scale as 1 (suspicious enhancement) (arrows). On subtraction image (b), there is definite contrast enhancement of the lesion, which was defined as having a higher value compared to the subtracted temporal white matter. Both reviewers recorded the visual scale as 2 (definite contrast enhancement) (arrow).
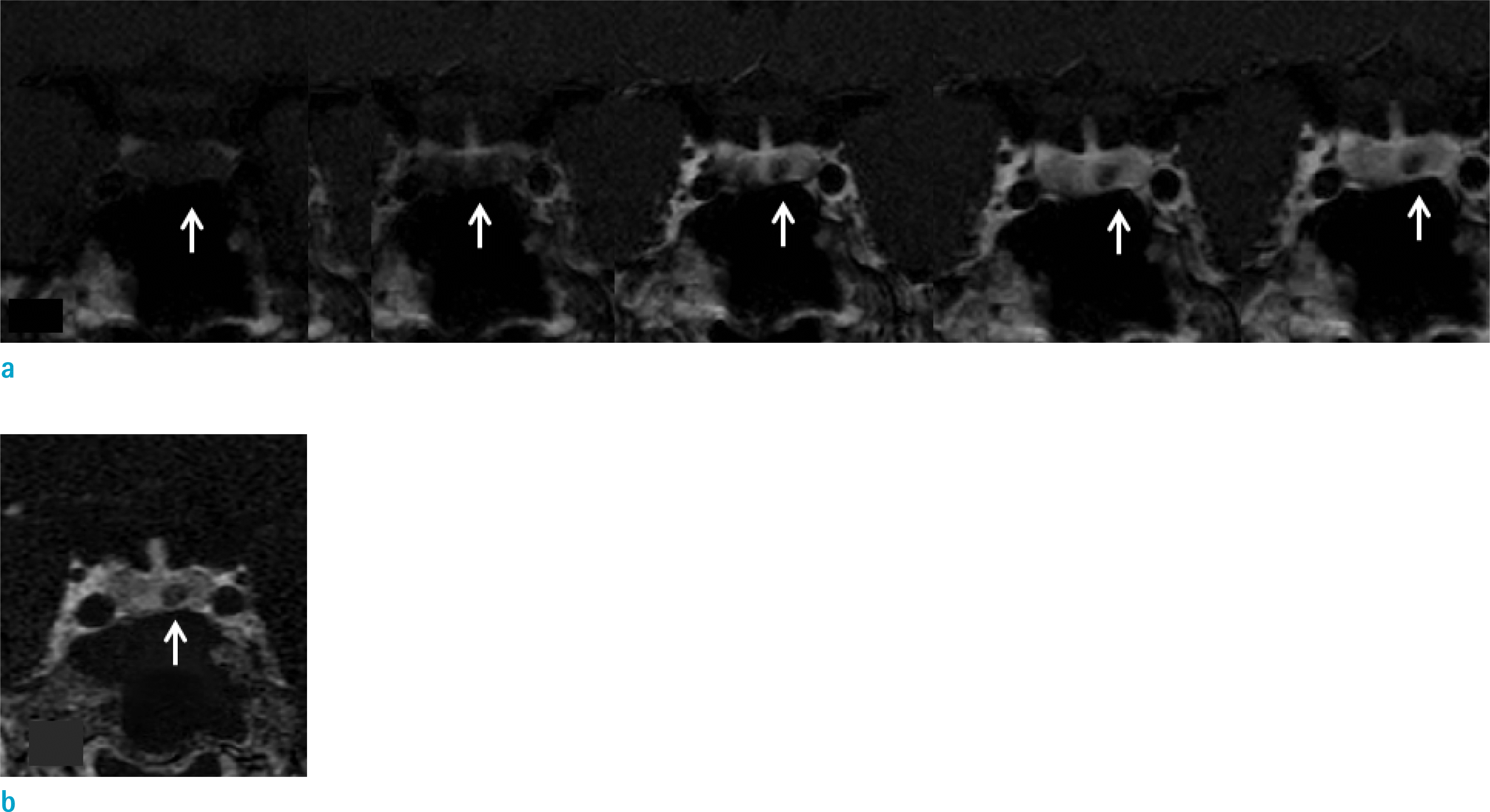
Fig. 2.
Images of a 32-year-old female patient diagnosed with Rathke's cleft cyst. On conventional dynamic images (a), reviewer 1 recorded the visual scale as 1 (suspicious contrast enhancement) and reviewer 2 recorded the visual scale as 2 (definite contrast enhancement) (arrows). On subtraction image (b), there is no contrast enhancement of the lesion and both reviewers recorded the visual scales as 0 (no contrast enhancement) (arrow).
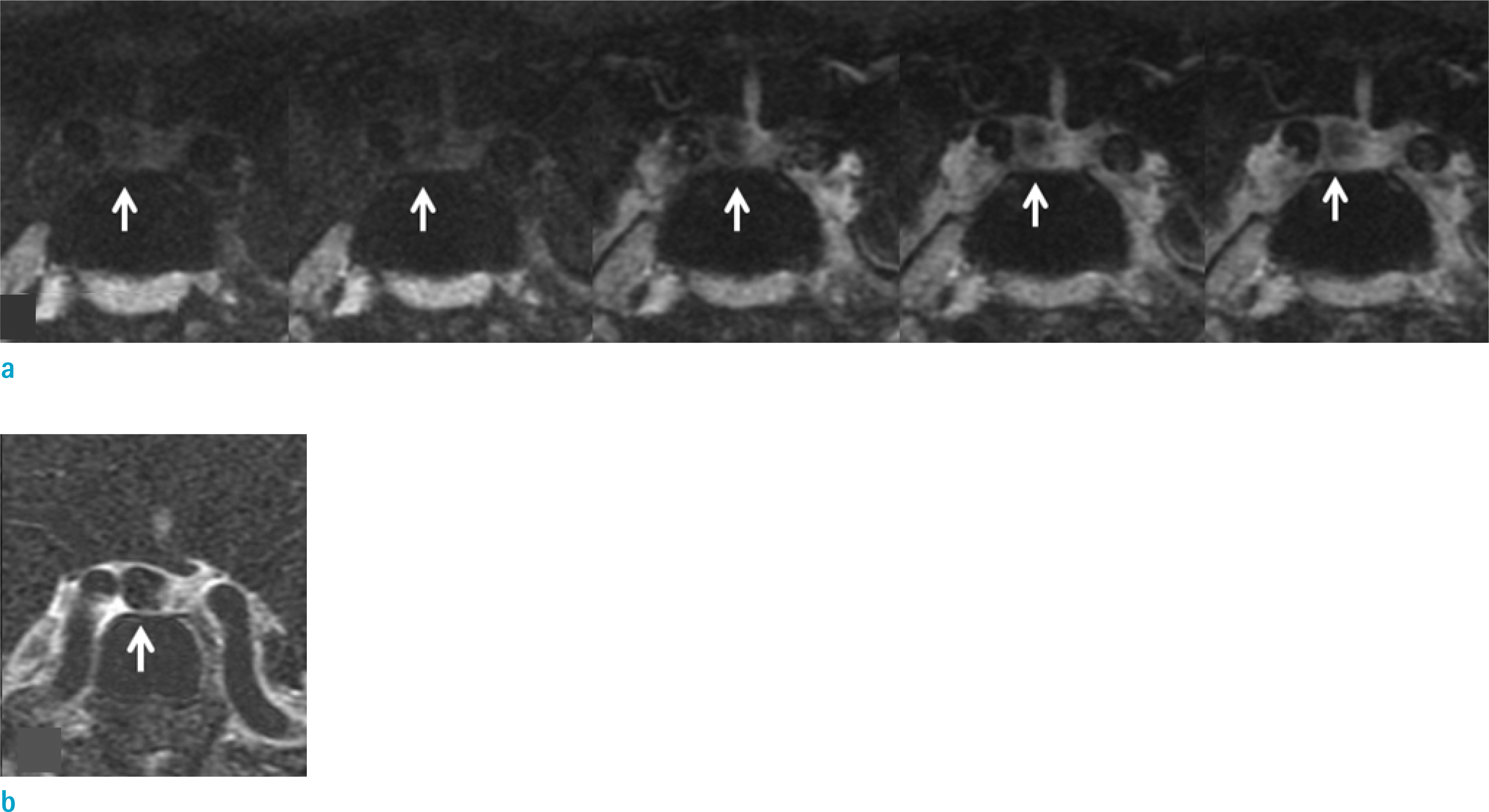
Table 1.
Imaging Parameters for the Conventional Dynamic Enhancement and T1 3D Fast Spin Echo Sequences
Table 2.
Interpretati of Contrast Enhance on Time (second) Taken for Deterrmination
DCE | T1 3D subtraction | P value | |
---|---|---|---|
Cushing + RCC (n = 3 | 35) 17.9 ± 12.0 | 8.1 ± 5.2 | < 0.001 |
Cushing (n = 12) | 23.5 ± 15.2 | 9.5 ± 6.7 | < 0.001 |
RCC (n = 23) | 15.0 ± 8.7 | 7.4 ± 4.2 | < 0.001 |
Table 3.
Interpretation Results for Diagnosis of Cushing Disease and RCC on DCE and Subtraction Images