Abstract
Purinergic receptors play an important role in regulating gastrointestinal (GI) motility. Interstitial cells of Cajal (ICCs) are pacemaker cells that regulate GI smooth muscle activity. We studied the functional roles of external adenosine 5′-triphosphate (ATP) on pacemaker activity in cultured ICCs from mouse small intestines by using the whole-cell patch clamp technique and intracellular Ca2+ ([Ca2+]i) imaging. External ATP dose-dependently depolarized the resting membrane and produced tonic inward pacemaker currents, and these effects were antagonized by suramin, a purinergic P2 receptor antagonist. ATP-induced effects on pacemaker currents were suppressed by an external Na+-free solution and inhibited by the nonselective cation channel blockers, flufenamic acid and niflumic acid. The removal of external Ca2+ or treatment with thapsigargin (inhibitor of Ca2+ uptake into endoplasmic reticulum) inhibited the ATP-induced effects on pacemaker currents. Spontaneous [Ca2+]i oscillations were enhanced by external ATP. These results suggest that external ATP modulates pacemaker activity by activating nonselective cation channels via external Ca2+ influx and [Ca2+]i release from the endoplasmic reticulum. Thus, it seems that activating the purinergic P2 receptor may modulate GI motility by acting on ICCs in the small intestine.
Gastrointestinal (GI) smooth muscles show periodic spontaneous phasic contractions without neuronal stimulation. These spontaneous GI smooth muscle contractions depend on the electrical cyclic depolarization of membrane potentials called slow waves. Slow waves determine the frequency and timing of GI smooth muscle contractions and contribute to peristaltic and segmenting motor activity.1 Many enteric neurotransmitters, endogenous substances, and drugs modulate GI motility by changing the frequency and configuration of slow waves.2 Therefore, slow waves play an important role in regulating GI motility. Slow waves are caused by the interstitial cells of Cajal (ICCs).34 ICCs are specialized enteric cells that generate slow waves by producing spontaneous inward currents, referred to as pacemaker currents, in the GI tract.5 They form networks with each other and are connected with neighboring smooth muscles through gap junctions; thus, pacemaker currents are directly transmitted to smooth muscles via gap junctions.6 In addition, enteric neuronal varicosities are very closely associated with ICCs and transduce neuronal signals to smooth muscles.7 ICCs also act as stretch receptors, causing changes in electrical slow waves upon luminal distension.8 Many studies have shown that ICC network disruption is related with several motility disorders in the GI tract, suggesting that ICCs are very important components in GI motility.910
Adenosine 5′-triphosphate (ATP) is an intracellular energy source that also acts as an extracellular signal molecule through purinergic receptors. Purinergic receptors are widely distributed in the body and their activation represents diverse physiological and pathological activities.11 Purinergic receptors are classified into two types: P1 and P2. P1 receptors are sensitive to adenosine, whereas P2 receptors are sensitive to ATP.12 Purinergic receptors are also distributed in the GI tract and they play an important role in modulating motility, secretion, and absorption.13 In the GI tract, ATP is released from enteric neurons to act on the P2 receptors of smooth muscles, inducing relaxation and contraction. ATP also acts on prejunctional nerve terminals and modulates transmitter release, thereby having a role in the control of smooth muscle activity.14 Furthermore, ATP modulates enteric synaptic transmission, and thereby is involved in controlling mucosal secretion and absorption.1516 Additionally, purinergic P2 receptors were detected by immunohistochemistry and P2 mRNA was observed in ICCs from the small intestines of mice and guinea pigs.17181920 These reports suggest that purinergic receptors can modulate GI motility by acting on ICCs. However, a functional analysis of purinergic receptors on ICC pacemaker currents has not yet been performed. Therefore, we investigated the role of external ATP on electrical pacemaker activity in cultured ICCs from the mouse small intestine.
The animal experiments were conducted in accordance with the Guide for the Care and Use of Laboratory Animals and were approved by the Ethics Committee of Chosun University (IACUC approval No, CIACUC 2017-A0047). Balb/C mice (3–5 days) of both sexes were used. The mice were kept in a temperature-controlled room regulated on a 12:12 hour light-dark cycle.
The cells were bathed in a solution containing 5 mM KCl, 135 mM NaCl, 2 mM CaCl2, 10 mM glucose, 1.2 mM MgCl2, and 10 mM HEPES, adjusted to pH 7.2 with Tris. NaCl was replaced with equimolar N-methyl-D-glucamine to make Na+-free solution, and CaCl2 was omitted in the bath solution for Ca2+-free solution. The pipette solution contained 20 mM K-aspartate, 120 mM KCl, 5 mM MgCl2, 2.7 mM K2ATP, 0.1 mM Na2GTP, 2.5 mM creatine phosphate disodium, 5 mM HEPES, and 0.1 mM EGTA, adjusted to pH 7.2 with Tris.
The drugs used were ATP, suramin, flufenamic acid, niflumic acid, and thapsigargin from Sigma (San Diego, CA, U.S.A.).
Mice of both sexes were anesthetized with ether and were sacrificed by cervical dislocation. The small intestines from 1 cm below the pyloric ring to the cecum were removed and opened along the mesenteric border. The luminal contents were washed away with a Krebs-Ringer bicarbonate solution. The tissues were pinned to the base of a Sylgard dish and the mucosa were removed by sharp dissection. Small strips of small intestinal muscle were equilibrated in Ca2+-free Hank's solution containing 5.36 mM KCl, 125 mM NaCl, 0.34 mM NaOH, 0.44 mM Na2HCO3, 10 mM glucose, 2.9 mM sucrose, and 11 mM HEPES for 20 min, and the cells were dispersed from these strips with an enzyme solution containing the following; 1.3 mg/mL collagenase (Worthington Biochemical Co, Lakewood, NJ, USA), 2 mg/mL bovine serum albumin (Sigma), 2 mg/mL trypsin inhibitor (Sigma), and 0.27 mg/mL ATP. Cells were plated onto sterile glass coverslips coated with murine poly L-lysine (2.5 g/mL, Sigma) in 35 mm culture dishes. Freshly dispersed cells were allowed to stabilize for 2 days in an incubator (atmosphere 95% O2-5% CO2) in a smooth muscle growth medium (Clonetics, San Diego, CA) supplemented with 2% antibiotics/antimycotics (Gibco, Grand Island, NY, USA) and stem cell factor (SCF, 5 ng/mL, Sigma).
ICCs were identified as cells with green fluorescent Kit proteins (ACK2) labeled with Alexa Fluor 488 (Molecular Probes, Eugene, OR, USA). using an inverted fluorescence microscope. The standard whole cell patch clamp configuration was employed to record membrane currents (voltage clamp) and potentials (current clamp). We recorded from small ICC clusters, as spontaneous inward currents from small groups of cells are more robust and regular than from single cells. Currents or potentials were amplified with an Axopatch 200B patch-clamp amplifier (Axon Instruments, Foster, CA, USA) and digitized with a 16-bit analog to digital converter (Digidata 1550A; Axon Instruments, Foster, CA, USA). Data was sampled at 4 kHz and filtered at 5 kHz using an eight-pole Bessel filter for whole cell experiments. All data was analyzed using clampfit (pCLAMP version, 10.2; Axon Instruments) and Graph Pad Prism (version 4.0) software. All experiments were performed at 30℃.
Changes in intracellular Ca2+ concentration ([Ca2+]i) were measured with fluo-4 AM, which was initially dissolved in dimethyl sulfoxide and stored at −20℃. Cultured ICCs on glass coverslips in 30 mm dishes were washed three times with a bath solution (5 mM KCl, 135 mM NaCl, 2 mM CaCl2, 10 mM glucose, 1.2 mM MgCl2, and 10 mM HEPES, adjusted to pH 7.4 with Tris). Then ICCs were treated with 1 µM fluo-4 AM and incubated in bath solution in incubator at 37℃ for 10 min. After incubation, ICCs were rinsed twice with bath solution and mounted on a perfusion chamber. The ICCs were scanned every 0.4 seconds with a Nikon Eclipse TE200 inverted microscope equipped with a Perkin-Elmer Ultraview confocal scanner and a Hamamatsu Orca ER 12-bit CCD camera (×200). Fluorescence was excited at a wavelength of 488 nm, and emitted light was observed at 515 nm. During Ca2+ imaging, the temperature of the perfusion chamber containing the ICCs was maintained at 30℃. Variations in the intracellular Ca2+ fluorescence emission intensity were expressed as F1/F0, where F0 is the intensity of the first image.
Data are expressed as means±standard error (SE). All statistical analyses were performed using Graphpad Prism and the sigma plot program. We used Student's t-test to compare single values under control and experimental conditions or ANOVA with Dunnett's post-hoc analysis to compare groups of data. In all statistical analyses, p<0.05 was considered statically significant. The n values reported in the text refer to the number of cells used in the patch clamp experiments.
Under the current clamp mode (I=0), ICCs generated spontaneous pacemaker potentials. Exposure to ATP (200 µM) depolarized the resting membrane potential with a decrease in the amplitude of pacemaker potentials (Fig. 1A). Under control conditions, the resting membrane potential and the amplitude of pacemaker potentials were −58±3 mV and 23±7 mV, respectively. In the presence of ATP, the resting membrane potential and the amplitude of pacemaker potentials were −36±2 mV and 5±2 mV, respectively (n=7; Fig. 1B, C). Under a voltage clamp at a holding potential of −70 mV, exposure to ATP (50–200 µM) induced tonic inward currents (resting currents) and reduced the amplitude of pacemaker currents in a concentration-dependent manner (Fig. 2A-C). The summarized values and a bar graph of the effects of ATP on pacemaker currents are shown in Fig. 2D, E (n=5–6).
To know whether external ATP-induced actions on pacemaker currents were mediated through purinergic P2 receptors, we pretreated cells with suramin, a purinergic P2 receptor antagonist. Suramin (100 µM) itself had no effects on pacemaker currents. In the presence of suramin, ATP (200 µM)-induced tonic inward currents were markedly suppressed but frequency did not change (n=6; Fig. 3A). Under normal conditions, the resting currents of the tonic inward currents induced by ATP were −453±35 pA, and in presence of suramin, the resting currents induced by ATP were −42±18 pA (Fig. 3B). The frequency upon ATP treatment was 4±2 cycles/min and in the presence of suramin, 16±5 cycles/min (Fig. 3C).
External ATP-gated nonselective cation channels (NSCCs) act through purinergic P2 receptors.21 To determine the characteristics of the tonic inward currents induced by ATP, we tested the effects of ATP in the presence of an external Na+-free solution or NSCC blockers. Exposure to the external Na+-free solution abolished the generation of pacemaker currents. Under this condition, the tonic inward currents induced by ATP (200 µM) were reduced (Fig. 4A). Under normal conditions, the resting currents of the tonic inward currents induced by ATP were −409±27 pA, and in presence of external Na+-free solution, the resting currents induced by ATP were −89±40 pA (n=5; Fig. 4B). NSCC inhibitors, flufenamic acid (10 µM) and niflumic acid (10 µM), abolished the generation of pacemaker currents and blocked the ATP-induced tonic inward currents (Fig. 5A, B). In the presence of flufenamic acid and niflumic acid, the resting currents of tonic inward currents induced by ATP were −29±8 pA (n=5) and −40±12 pA (n=6), respectively (Fig. 5C, D). These results suggest that ATP-induced tonic inward currents are mediated by the activation of NSCCs.
To investigate the role of extracellular Ca2+ or [Ca2+]i, the ICCs were exposed to ATP under external Ca2+-free conditions and in the presence of thapsigargin, a Ca2+-ATPase inhibitor in the endoplasmic reticulum. The use of an external Ca2+-free solution completely inhibited the pacemaker currents in the voltage clamp mode at a holding potential of −70 mV. Under this condition, ATP (200 µM)-induced tonic inward currents were suppressed (n=5; Fig. 6A). Thapsigargin treatment (10 µM) also inhibited ICC pacemaker currents and blocked ATP (200 µM)-induced tonic inward currents (Fig. 6B). The resting currents during ATP treatment in the Ca2+-free solution and in the presence of thapsigargin were significantly different from control resting currents obtained in a normal solution and in the absence of thapsigargin (Fig. 6C, D).
As [Ca2+]i oscillations in ICCs are considered the primary mechanism for pacemaker activity in the GI tract,22 we examined the effect of ATP on [Ca2+]i oscillations in ICCs. In this study, we measured spontaneous [Ca2+]i oscillations of ICCs that are connected with cell clusters. Spontaneous [Ca2+]i oscillations were observed in many ICCs (low magnification; data not shown), which were loaded with fluo-3AM and spontaneous regular [Ca2+]i oscillations were seen over time (Fig. 7A). Specifically, the frequency of [Ca2+]i oscillation was 8–10 cycles/min. The difference in frequency between [Ca2+]i oscillation and pacemaker activity is the reason that we scanned every 0.4 seconds for recording of [Ca2+]i. In the presence of ATP (200 µM), the basal points of [Ca2+]i oscillations increased but the peak points of [Ca2+]i oscillations were slightly decreased. The percentage increasing of frequency by ATP is shown in Fig. 7B. These results suggest that the action of ATP in ICCs may involve the regulation of spontaneous [Ca2+]i oscillations.
In the present study, external ATP modulated the pacemaker activity of intestinal ICCs by activating NSCCs through a [Ca2+]i release-dependent pathway.
Purinergic receptors play an important role in regulating GI motility. Purinergic receptors are classified into P1 and P2 receptors. P1 receptors are activated by adenosine whereas P2 receptors are activated by ATP. P2 receptors consist of P2X receptors and P2Y receptors. P2X receptors are ligand-gated ion channels and P2Y receptors are G-protein-coupled receptors. Generally, P2Y receptors are activated by ATP-mediated inhibitory motor control through enteric neurons or by directly acting on smooth muscles in the GI tract.23242526 In contrast, P2X receptors are activated by ATP-mediated excitatory motor control in the GI tract.142728 ICCs are enteric pacemaker cells that regulate smooth muscle contractility and also express P2X and P2Y receptors in humans.17181920 The generation of spontaneous pacemaker currents of ICCs spreads to neighboring smooth muscles via gap junctions, depolarizing the membranes, leading to smooth muscle contraction by activating voltage-dependent Ca2+ channels. In the present study, external ATP depolarized the resting membrane potential and produced tonic inward currents in ICCs. Suramin, a nonspecific P2 antagonist, blocked these ATP-induced effects on pacemaker currents. It has been reported that P2X receptor activation modifies pacemaker activity in mouse intestinal ICCs.29 Purinergic P2X and P2Y receptors have been identified by immunohistochemistry in small intestinal ICCs.1620 However, purinergic P2Y receptors inhibit GI smooth muscle contraction, promoting relaxation. In addition, recently, it was reported that PDGFRα+ cells (fibroblast-like cells) could transduce purinergic signals. P2Y receptors in PDGFRα+ cells hyperpolarize the membrane by activating apamin-sensitive Ca2+-activated K+ channels, which contribute to smooth muscle relaxation in small intestines.3031 On the contrary, in urethral smooth muscle, external ATP contracted smooth muscle by modulating pacemaker activity of urethral ICCs through P2Y receptor activation.32 Although we did not identify the purinergic P2 receptor subtypes, the data that external ATP-induced membrane depolarization and tonic inward currents in intestinal ICCs, suggests ATP action on pacemaker activity in ICCs may be mediated by purinergic P2X receptor activation. Further experiments are required to identify the P2 receptor subtypes involved in ATP-induced effects on ICCs.
NSCCs or Ca2+-dependent Cl- channels were suggested as pacemaker channels in intestinal ICCs.333435 Also, P2X receptors activated NSCCs that are highly permeable to Ca2+.213637 In the present study, external ATP-induced tonic inward currents on ICCs were suppressed or blocked by the removal of external Na+ and by treatment with NSCC blockers, flufenamic acid or niflumic acid. In the canine colon, P2X receptor activation depolarizes and excites smooth muscles via NSCC activation.38 Also, carbachol treatment showed similar effects to ATP treatment in intestinal ICCs. Carbachol depolarizes the membrane and produces tonic inward currents by activating NSCCs in ICCs, and these carbachol-induced effects were blocked by removing external Na+ or treating with flufenamic acid or niflumic acid.39 Based on these results, we hypothesize that external ATP-induced tonic inward currents in intestinal ICCs are mediated by NSCC activation.
The spontaneous pacemaker activity of intestinal ICCs is dependent on extracellular Ca2+ and release of [Ca2+]i from the endoplasmic reticulum. Particularly, IP3-dependent [Ca2+]i oscillations are the primary mechanism for generating pacemaker activity, which is well-matched with the periodic activation of pacemaker channels.22 In the present study, external ATP-induced tonic inward currents were markedly suppressed by removing extracellular Ca2+ or treating with thapsigargin, a Ca2+ uptake inhibitor in the endoplasmic reticulum. Also, as observed by Ca2+-imaging, external ATP increased [Ca2+]i oscillations. In renal vascular myocytes, Ca2+-entry following P2X receptor activation induced IP3-dependent Ca2+ release from the endoplasmic reticulum,40 whereas P2Y receptors mediated the modulation of [Ca2+]i oscillations in ICCs of guinea pigs.19 Thus, it seems that extracellular Ca2+ influx and [Ca2+]i release from endoplasmic reticulum are needed for ATP-induced action on intestinal ICCs.
In conclusion, external ATP depolarized the membrane and induced tonic inward currents in intestinal ICCs. These effects were mediated by activation of purinergic P2 receptors, which activated NSCCs through extracellular Ca2+ influx and [Ca2+]i release. Thus, it seems that external ATP can regulate smooth muscle activity indirectly by acting on ICCs and can be therapeutic targets for disorders of altered GI motility.
Figures and Tables
FIG. 1
Effects of external ATP on pacemaker potentials in cultured ICCs from mouse small intestine. (A) The treatment with 200 µM ATP depolarized the membranes and decreased the amplitude of pacemaker potentials. The effects of ATP on pacemaker potentials are summarized in (B) and (C). Bars represent mean±SE values. *p<0.05: significantly different from control. RMP: resting membrane potential.
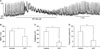
FIG. 2
Concentration-dependent effects of ATP on pacemaker currents in cultured ICCs from mouse small intestine. Pacemaker currents of ICCs recorded at a holding potential of −70 mV and exposed to various concentrations of ATP (50–200 µM). (A-C) External ATP produced tonic inward currents and reduced the amplitude of pacemaker currents in a concentration-dependent manner. Responses to ATP are summarized in (D) and (E). Bars represent mean±SE values. *p<0.05: significantly different from control.
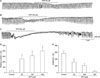
FIG. 3
Effects of purinergic P2 receptor antagonism on ATP-induced tonic inward currents of ICCs from mouse small intestine. (A) Pretreatment with a purinergic P2 receptor antagonist, suramin (200 µM) alone, had no effect on pacemaker currents. In the presence of suramin, ATP-induced tonic inward currents were essentially blocked. Responses to ATP in the presence of suramin are summarized in (B) and (C). Bars represent mean±SE values. *p<0.05: significantly different from control.
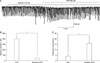
FIG. 4
The effects of external Na+-free solution on the ATP-induced tonic inward currents of ICCs from mouse small intestine. (A) Application of an external Na+-free solution abolished the generation of pacemaker currents. The tonic inward currents induced by ATP (200 µM) were markedly suppressed. Responses to ATP in the external Na+-free solution are summarized in (B). Bars represent mean±SE values. *p<0.05: significantly different from control.
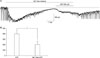
FIG. 5
The effects of nonselective cation channel (NSCC) blockage on the ATP-induced tonic inward currents of ICCs from mouse small intestine. (A) NSCC inhibitors, flufenamic acid (50 µM) and (B) niflumic acid (50 µM), abolished the generation of pacemaker currents. The tonic inward currents induced by ATP (200 µM) were essentially blocked. Responses to ATP in the presence of flufenamic acid and niflumic acid are summarized in (C) and (D). Bars represent mean±SE values. *p<0.05: significantly different from control.
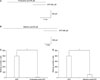
FIG. 6
The effects of external Ca2+-free solution and thapsigargin treatment on the ATP-induced tonic inward currents of ICCs from mouse small intestine. (A) Application of an external Ca2+-free solution or (B) thapsigargin, (10 µM), abolished the generation of pacemaker currents. The tonic inward currents induced by ATP (200 µM) were markedly suppressed. Responses to ATP in the external Ca2+-free solution and in the presence of thapsigargin are summarized in (C) and (D). Bars represent mean±SE values. *p<0.05: significantly different from control.
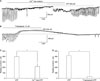
ACKNOWLEDGEMENTS
This work was supported by a grant from the Clinical Research Center of the Chosun University Hospital (2015).
References
1. Szurszewski JH. Electrical basis for gastrointestinal motility. In : Johnson LR, editor. Physiology of the Gastrointestinal Tract. 2nd ed. New York: Ravin Press;1987. p. 384–422.
2. Olsson C, Holmgren S. The control of gut motility. Comp Biochem Physiol A Mol Integr Physiol. 2001; 128:481–503.


3. Sanders KM. A case for interstitial cells of Cajal as pacemakers and mediators of neurotransmission in the gastrointestinal tract. Gastroenterology. 1996; 111:492–515.


4. Thomsen L, Robinson TL, Lee JC, Farraway LA, Hughes MJ, Andrews DW, et al. Interstitial cells of Cajal generate a rhythmic pacemaker current. Nat Med. 1998; 4:848–851.


5. Koh SD, Sanders KM, Ward SM. Spontaneous electrical rhythmicity in cultured interstitial cells of cajal from the murine small intestine. J Physiol. 1998; 513:203–213.


6. Hanani M, Farrugia G, Komuro T. Intercellular coupling of interstitial cells of cajal in the digestive tract. Int Rev Cytol. 2005; 242:249–282.


7. Sanders KM, Hwang SJ, Ward SM. Neuroeffector apparatus in gastrointestinal smooth muscle organs. J Physiol. 2010; 588:4621–4639.


8. Won KJ, Sanders KM, Ward SM. Interstitial cells of Cajal mediate mechanosensitive responses in the stomach. Proc Natl Acad Sci U S A. 2005; 102:14913–14918.


9. Vanderwinden JM, Rumessen JJ. Interstitial cells of Cajal in human gut and gastrointestinal disease. Microsc Res Tech. 1999; 47:344–360.


10. Jain D, Moussa K, Tandon M, Culpepper-Morgan J, Proctor DD. Role of interstitial cells of Cajal in motility disorders of the bowel. Am J Gastroenterol. 2003; 98:618–624.


12. Ralevic V, Burnstock G. Receptors for purines and pyrimidines. Pharmacol Rev. 1998; 50:413–492.
13. Giaroni C, Knight GE, Ruan HZ, Glass R, Bardini M, Lecchini S, et al. P2 receptors in the murine gastrointestinal tract. Neuropharmacology. 2002; 43:1313–1323.


14. Bornstein JC. Purinergic mechanisms in the control of gastrointestinal motility. Purinergic Signal. 2008; 4:197–212.


15. Christofi FL. Purinergic receptors and gastrointestinal secretomotor function. Purinergic Signal. 2008; 4:213–236.


16. Burnstock G. The journey to establish purinergic signalling in the gut. Neurogastroenterol Motil. 2008; 20:Suppl 1. 8–19.


17. Burnstock G, Lavin S. Interstitial cells of Cajal and purinergic signalling. Auton Neurosci. 2002; 97:68–72.


18. Vanderwinden JM, Timmermans JP, Schiffmann SN. Glial cells, but not interstitial cells, express P2X7, an ionotropic purinergic receptor, in rat gastrointestinal musculature. Cell Tissue Res. 2003; 312:149–154.


19. Van Nassauw L, Costagliola A, Van Op den Bosch J, Cecio A, Vanderwinden JM, Burnstock G, et al. Region-specific distribution of the P2Y4 receptor in enteric glial cells and interstitial cells of Cajal within the guinea-pig gastrointestinal tract. Auton Neurosci. 2006; 126-127:299–306.


20. Chen H, Redelman D, Ro S, Ward SM, Ordög T, Sanders KM. Selective labeling and isolation of functional classes of interstitial cells of Cajal of human and murine small intestine. Am J Physiol Cell Physiol. 2007; 292:C497–C507.


21. Jiang R, Taly A, Grutter T. Moving through the gate in ATP-activated P2X receptors. Trends Biochem Sci. 2013; 38:20–29.


22. Ward SM, Ordog T, Koh SD, Baker SA, Jun JY, Amberg G, et al. Pacemaking in interstitial cells of Cajal depends upon calcium handling by endoplasmic reticulum and mitochondria. J Physiol. 2000; 525:355–361.


23. Gallego D, Vanden Berghe P, Farré R, Tack J, Jiménez M. P2Y1 receptors mediate inhibitory neuromuscular transmission and enteric neuronal activation in small intestine. Neurogastroenterol Motil. 2008; 20:159–168.
24. Hwang SJ, Blair PJ, Durnin L, Mutafova-Yambolieva V, Sanders KM, Ward SM. P2Y1 purinoreceptors are fundamental to inhibitory motor control of murine colonic excitability and transit. J Physiol. 2012; 590:1957–1972.


25. Hedlund H, Fändriks L, Delbro D, Fasth S. Effect of alpha, beta-methylene ATP on distal colonic and rectal motility--a possible involvement of P2-purinoceptors in pelvic nerve mediated non-adrenergic, non-cholinergic contraction. Acta Physiol Scand. 1986; 127:425–432.


26. Martínez-Cutillas M, Gil V, Gallego D, Mañé N, Clavé P, Martín MT, et al. α,β-meATP mimics the effects of the purinergic neurotransmitter in the human and rat colon. Eur J Pharmacol. 2014; 740:442–454.


27. Bian X, Ren J, DeVries M, Schnegelsberg B, Cockayne DA, Ford AP, et al. Peristalsis is impaired in the small intestine of mice lacking the P2X3 subunit. J Physiol. 2003; 551:309–322.


28. Matsuo K, Katsuragi T, Fujiki S, Sato C, Furukawa T. ATP release and contraction mediated by different P2-receptor subtypes in guinea-pig ileal smooth muscle. Br J Pharmacol. 1997; 121:1744–1748.


29. Furuzono S, Nakayama S, Imaizumi Y. Purinergic modulation of pacemaker Ca2+ activity in interstitial cells of Cajal. Neuropharmacology. 2005; 48:264–273.


30. Kurahashi M, Mutafova-Yambolieva V, Koh SD, Sanders KM. Platelet-derived growth factor receptor-α-positive cells and not smooth muscle cells mediate purinergic hyperpolarization in murine colonic muscles. Am J Physiol Cell Physiol. 2014; 307:C561–C570.


31. Peri LE, Sanders KM, Mutafova-Yambolieva VN. Differential expression of genes related to purinergic signaling in smooth muscle cells, PDGFRα-positive cells, and interstitial cells of Cajal in the murine colon. Neurogastroenterol Motil. 2013; 25:e609–e620.


32. Bradley E, Kadima S, Kyle B, Hollywood MA, Thornbury KD, McHale NG, et al. P2X receptor currents in smooth muscle cells contribute to nerve mediated contractions of rabbit urethral smooth muscle. J Urol. 2011; 186:745–752.


33. Kim BJ, Lim HH, Yang DK, Jun JY, Chang IY, Park CS, et al. Melastatin-type transient receptor potential channel 7 is required for intestinal pacemaking activity. Gastroenterology. 2005; 129:1504–1517.


34. Nakayama S, Kajioka S, Goto K, Takaki M, Liu HN. Calcium-associated mechanisms in gut pacemaker activity. J Cell Mol Med. 2007; 11:958–968.


35. Hwang SJ, Blair PJ, Britton FC, O'Driscoll KE, Hennig G, Bayguinov YR, et al. Expression of anoctamin 1/TMEM16A by interstitial cells of Cajal is fundamental for slow wave activity in gastrointestinal muscles. J Physiol. 2009; 587:4887–4904.


36. Alvarez J, Coulombe A, Cazorla O, Ugur M, Rauzier JM, Magyar J, et al. ATP/UTP activate cation-permeable channels with TRPC3/7 properties in rat cardiomyocytes. Am J Physiol Heart Circ Physiol. 2008; 295:H21–H28.


37. Koshimizu TA, Van Goor F, Tomić M, Wong AO, Tanoue A, Tsujimoto G, et al. Characterization of calcium signaling by purinergic receptor-channels expressed in excitable cells. Mol Pharmacol. 2000; 58:936–945.


38. Monaghan KP, Koh SD, Ro S, Yeom J, Horowitz B, Sanders KM. Nucleotide regulation of the voltage-dependent nonselective cation conductance in murine colonic myocytes. Am J Physiol Cell Physiol. 2006; 291:C985–C994.

