Abstract
Background
Niemann Pick disease (NP) is a rare, lysosomal storage disorder due to deficiency of the intra-lysosomal enzyme acid sphingomyelinase (ASM) resulting in intracellular accumulation of sphingomyelin. We evaluated a tandem mass spectrometry (MS/MS) method to analyze ASM activity in dried blood spots (DBS) that may be suitable for laboratory diagnosis of NP including high throughput screening of at-risk populations and potentially for newborn screening.
Methods
ASM activity was measured in 3.2 mm punches from DBS. The eluate was incubated with the ASM substrate (N-Hexanoyl-D-erythro-sphingosylphosphorylcholine [C6-sphingomyelin (C29H59N2O6P)]) and an internal standard (N-butyroyl-D-erythro-sphingosine [C4-ceramide (C22H43NO3)]). ASM product and IS were analyzed using MS/MS in multiple reaction monitoring mode for transitions m/z 370.6>264.3 (ASM internal standard) and m/z 398.6>264.3 (ASM product).
Results
ASM activities were stable for up to 2 months at or below 4℃. Position of the punch in the DBS and/or hematocrit of the DBS had a limited effect on ASM activities. Both intra- and inter-assay variability were below 10%. There was no carry-over. The median ASM activity in 2,085 newborn infants was 9.5 µmol/h/L (mean 10.6) with a SD of 5.06 µmol/h/L. Six of 2,085 (0.3%) infants were found to have ASM activities below the cut-off of 2.5 µmol/h/L. ASM activities were below the cut-off level in all 10 previously diagnosed cases with NP (range: 0.16 to 2.08 µmol/h/L).
Niemann Pick disease (NP) types A and B (A/B) is a lysosomal storage disorder caused by deficiency of acid sphingomyelinase (ASM) that catalyzes the hydrolysis of sphingomyelin (SPM) to yield ceramide and phosphorylcholine [1]. Sphingomyelin preferentially accumulates in the cells of the monocyte-macrophage system resulting in a complex, multi-organ phenotype [1]. NP is a panethnic disorder with an estimated global incidence of 1: 100,000 live births. The incidence of NP is higher in populations of Northern African, Arab or Ashkenazi Jewish descent [2].
NP A/B can be divided into two distinct subtypes based on their rate of progression and involvement of the central nervous system. Niemann Pick type C in contrast is a genetically distinct disorder resulting from defective intracellular trafficking of cholesterol with secondary accumulation of glycosphingolipids [3]. NP-A represents the neuropathic and most severe form, characterized by hepatosplenomegaly, psychomotor retardation, recurrent respiratory infections and subsequent death during or before the third decade of life. NP-B is typically of later onset and milder in manifestation, with no neurologic involvement [1]. Therapies for NP are mostly supportive in nature. Only few patients with NP-B underwent successful bone marrow transplantation [1]. A phase 2 trial evaluating enzyme replacement therapy for NP is currently under development [4].
The gold standard for diagnosis of NP is enzyme analysis in leukocytes and fibroblasts using fluorometric or colorimetric assays [5]. Assays using radioactive substrates are obsolete. Once deficiency of ASM is demonstrated molecular analysis of the sphingomyelin phosphodiesterase-1 (SMPD1) gene should be sought to identify pathogenic mutations as basis for genetic counselling and prenatal diagnosis [1].
Dried blood spots (DBS) as the source of ASM may have similar advantages as reported for other lysosomal storage disorders using a recently developed tandem mass spectrometry (MS/MS) method [6]. The aim of our work was to adapt and validate a MS/MS method to measure ASM activities in DBS in a clinical laboratory setting.
A total of 2,085 random, de-identified newborn screening samples were provided by the State Newborn Screening Laboratory New York, Wadsworth Center, Albany, NY (Joe Orsini). DBS were collected from 24 to 72 hr after birth and stored at 4℃ with desiccants until shipment at room temperature (RT). Blood samples from healthy adults were used for validation following informed consent. Blood was collected into EDTA tubes and spotted (80 µL/spot) onto filter paper (Whatman 903, Whatman plc, Kent, UK), dried at RT and stored at different temperatures (-80℃, -20℃, 4℃, RT and 37℃ with desiccants). In addition ASM activity was measured in de-identified samples from 10 confirmed cases with NP.
A 20 mmol/L potassium phosphate solution (pH 7.1, Merck, Rahway, NJ, USA) was used for blood extraction, while vials of substrate and internal standard were provided by the Centers of Disease Control and Prevention (CDC), Atlanta, USA. Each vial contained 3.37 mg (5.9 µmol) of NP substrate, N-Hexanoyl-D-erythro-sphingosylphosphorylcholine (C6-sphingomyelin [C29H59N2O6P]), and 0.04 mg (0.1 µmol) of NP internal standard, N-butyroyl-D-erythro-sphingosine (C4-ceramide [C22H43NO3]), in a molar ratio of approximately 50:1. Vials were reconstituted with 150 µL of ASM detergent solution (120 g/L sodium taurocholate in water) and 17.85 mL of ASM buffer (0.930 mol/L sodium acetate, 0.604 mmol/L zinc chloride, pH 5.7). Reagents were completely dissolved and vials stored at -20℃.
The final NP assay cocktail contained 0.33 mmol/L substrate, 6.67 µmol/L internal standard, 1.0 g/L sodium taurocholate, 0.922 mol/L sodium acetate and 0.6 mmol/L zinc chloride. We used multichannel pipettors (Brand, Wertheim, Germany) and Eppendorf single-channel pipettes for reagent preparation (Eppendorf, Hamburg, Germany).
A 3.2 mm spot was punched from individual DBS directly into 96 well plates (Greiner Bio-one, Monroe, NC, USA) that contained 5 blanks, 2 positive and 2 negative controls. Punches were eluted for 1 hr with 80 µL of a 20 mmol/L potassium phosphate solution at 37℃ while shaking in a Heidolph incubator (Titramax 1000; Elk Grove Village, IL, USA). 10 µL of elution were mixed with 15 µL of a cocktail containing the ASM substrate and the ASM internal standard. Samples were incubated for 21 hr at 37℃, shaking at 750 rpm. The second day, 100 µL of ethylacetate :MeOH (1 :1) (Merck; BDH Prolabo Chemicals, Leicestershire, UK) were added to each well to stop the reaction; after mixing twice we transferred 100 µL of solution to a 96-deep well plate (Brand, Wertheim, Germany) and brought it to the final volume of 500 µL. For the organic extraction 400 µL of ethylacetate (Merck) and 400 µL distilled water (BDH Prolabo Chemicals) were added. After a 5 min centrifugation (3,000 rpm) 300 µL of the upper phase were transferred to another plate and dried using a Minivap (Porvair Science, Leatherhead, UK). Samples were reconstituted in 100 µL of 1:19 methanol : ethylacetate and shaken for 5 min. In the meantime the 96-well filter plate (Pall Corporations, Port Washington, NY, USA) was charged with silica gel 60 (Merck) and equilibrated with 2 washes of 400 µL of 1:19 methanol :ethylacetate.
Samples were then passed through the resin and collected together with two washes in a new 96-deep well plate and dried. Samples were reconstituted with 200 µL of 80 :20 :0.01 acetonitrile:water : formic acid (Merck; BDH Prolabo Chemicals) prior to analysis with MS/MS.
An API 3000 triple-quadruple MS/MS (PE Sciex, Perkin Elmer, MA, USA) in positive ion mode and analyst v.1.5 software (Applied Biosystems, MDS Sciex, Ontario, Canada) was used for analysis. Instrument settings were as follows :declustering potential of 20 V, entrance potential of 10 V, collision energy of 25 and 30 V (internal standard and product, respectively), collision cell exit potential of 15 V, focusing potential of 250 V, source temperature of 150℃, and ion spray voltage of 5,500 V. Analyses of ASM internal standard and product were done with multiple-reaction monitoring mode. MS/MS parameters were optimized to give the highest sensitivity for transitions m/z 370.6>264.3 (ASM internal standard) and m/z 398.6>264.3 (ASM product).
Statistical analysis was done using the software Statgraphic Centurion XVI (www.statlets.com) for enzyme activities at different hematocrit and position of the punch. Statistical significance was assumed for P<0.05.
ASM activity in DBS was analyzed in six distinct 3.2 mm punches after 7, 14, 21, 28 days and 2 months of storage at 37℃, RT, 4℃, -20℃ and -80℃. DBS were stored in plastic bags with desiccants. Activities were expressed as percentage of the ASM activity at -80℃ (Fig. 1). ASM activities were stable for 2 months when stored at or below 4℃ but decreased by about 20% when stored for 7 days at either RT or 37℃ (Fig. 1).
A sample stored at -20℃ was used to investigate the effect of hematocrit and position of punch on ASM activity. EDTA blood from a healthy adult was spun down. The cellular component was mixed with the plasma at different ratios to generate DBS with a range of different hematocrit (30-80%). In general the position of the punch in the DBS (central versus peripheral) did not show any effect on ASM activity, with the exception of activities measured at 30% (P=0.043) and 50% (P=0.003) hematocrit respectively (Fig. 2). Statistical significance was calculated using the software Statgraphic Centurion XVI.
ASM activities from peripheral and central punches at 50% hematocrit were combined and compared to combined ASM activities at 30%, 40%, 60%, 70%, and 80% hematocrit respectively. Only ASM activities between 50% and 70% were significantly different from each other (P=0.02). Statistical significance was calculated using the software Statgraphic Centurion XVI.
Ten punches of the same DBS were analyzed simultaneously to assess intra-assay variability while 4 DBS from the same individual were analyzed during 6 consecutive days to assess inter-assay variability. The CV was below 10% for both inter-assay (7.7%) and intra-assay variability (8.6%) (Table 1). No carry-over was observed (Table 2).
The median ASM activity in 2,085 newborn infants was 9.5 µmol/h/L (mean 10.6) with a SD of 5.06 µmol/h/L (Fig. 3, Table 3). Using the 0.5th population percentile the cut-off value was 2.5 µmol/h/L. Six of 2,085 (0.3%) infants were found to have ASM activities below or equal to the cut-off. Due to the nature of the study we were not able to confirm these cases. ASM activities were below this cut-off level in all 10 previously diagnosed cases with NP (range:0.16 to 2.08 µmol/h/L) (Table 3).
NP is a multiorgan lysosomal storage disorder with significant morbidity and in the case of NP-A, mortality [1]. Early diagnosis may increase the chances of improved outcome following therapeutic intervention, particularly in light of enzyme replacement therapy that is currently developed [3]. It may also provide the basis for timely genetic counselling so that affected families can make an informed decision on future pregnancies considering the recurrence risk for NP of 25% [1].
Access to enzyme and/or molecular analysis for NP may be limited or only available in specialized laboratories at present. Current techniques rely on blood and/or fibroblasts for analysis that may further hamper diagnosis of NP due to invasiveness, limitations of shipping and related expenses. DBS that are routinely used for newborn screening are relatively simple to handle and can be safely shipped over long distances to the diagnostic laboratory. ASM activities in DBS are stable for up to 2 months when the DBS are stored at or below 4℃, although enzyme activities decrease by 20% when stored at temperatures up to 37℃. ASM activities in neonatal DBS have been analysed using MS/MS simultaneously with activities of alpha-glucosidase for Pompe Disease, alpha-galactosidase for Fabry Disease and glucocerebrosidase for Gaucher Disease in 2 recent pilot studies in Austria [7] and in Hungary [8] respectively. Although used in a multiplex format for high throughput screening, to the best of our knowledge MS/MS has not been formally evaluated for analysis of ASM activities in DBS in the setting of a clinical diagnostic laboratory.
Mechtler and co-workers reported newborn screening results of 34,736 infants for Gaucher, Fabry, Pompe and NP respectively [7]. Although the cut-off for all enzymes tested, was set at the 0.1th percentile based on enzyme activities in 5,000 newborn samples, the cut-off for ASM activity was not validated against a larger number of known patients with NP [7]. However they used a two-tier analysis with retesting of positive samples in duplicate followed by molecular confirmatory testing. Their initial screen identified 28/34,736 (0.08%) individuals with ASM activities below the cut-off which is comparable to our positive rate of 0.29% considering the fact that our cut-off was set at the 0.5th percentile in an effort not to miss a diagnosis of NP. Due to a limited number of DBS per infant we were not able to retest any positive samples or do confirmatory molecular testing.
Another population based study reported 114/40,024 (0.28%) Hungarian newborn infants with low ASM among which 2 cases of NP patients were confirmed molecularly [8].
The performance characteristics demonstrate that this analytical approach is robust, reproducible and with acceptable intra- and inter-run variability. All of the 10 adult patients with known NP had ASM activities in DBS that were in the diagnostic range compared to those found in more than 2,000 randomly selected newborn infants. Unfortunately we did not have access to DBS from healthy adult controls, although ASM activities in adults are known to be higher than in newborn infants.
In conclusion, ASM activities can be reliably measured in DBS with MS/MS technology for clinical diagnosis of NP.
Figures and Tables
Fig. 1
Stability of ASM (median +/- SD) in DBS for 7, 14, 21, 28 days and 2 months at -20℃, 4℃, room temperature and at 37℃.
Abbreviations: ASM, acid sphingomyelinase; DBS, dried blood spots.
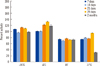
Fig. 2
Correlation between activity of ASM (median +/-SD) and hematocrit and location of the punch (peripheral versus central) respectively.
Abbreviation: ASM, acid sphingomyelinase.
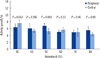
Fig. 3
ASM activity in anonymous newborn screening filter cards (N=2,085).
Abbreviation: ASM, acid sphingomyelinase.
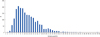
References
1. Schuchman EH, Desnick RJ. Scriver CR, Beaudet AL, Sly WS, Valle D, Childs B, Kinzler KW, Vogelstein B, editors. Niemann-Pick Disease Types A and B: acid sphingomyelinase deficiencies. The metabolic and molecular bases of inherited disease. 2001. 8th ed. New York: McGraw-Hill;3589–3610.
2. Simonaro CM, Desnick RJ, McGovern MM, Wasserstein MP, Schuchman EH. The demographics and distribution of type B Niemann-Pick disease: novel mutations lead to new genotype/phenotype correlations. Am J Hum Genet. 2002. 71:1413–1419.


4. Schuchman EH. The pathogenesis and treatment of acid sphingomyelinase-deficient Niemann-Pick disease. Int J Clin Pharmacol Ther. 2009. 47:Suppl 1. S48–S57.


5. He X, Chen F, Dagan A, Gatt S, Schuchman EH. A fluorescence-based, high-performance liquid chromatographic assay to determine acid sphingomyelinase activity and diagnose types A and B Niemann-Pick disease. Anal Biochem. 2003. 314:116–120.


6. Gelb MH, Turecek F, Scott CR, Chamoles NA. Direct multiplex assay of enzymes in dried blood spots by tandem mass spectrometry for the newborn screening of lysosomal storage disorders. J Inherit Metab Dis. 2006. 29:397–404.

