Abstract
Glioblastoma multiforme (GBM) is the most malignant World Health Organization grade IV brain tumor. GBM patients have a poor prognosis because of its resistance to standard therapies, such as chemotherapy and radiation. Since stem-like cells have been associated with the treatment resistance of GBM, novel therapies targeting the cancer stem cell (CSC) population is critically required. However, GBM CSCs share molecular and functional characteristics with normal neural stem cells (NSCs). To elucidate differential therapeutic targets of GBM CSCs, we compared surface markers of GBM CSCs with adult human NSCs and found that GD2 and CD90 were specifically overexpressed in GBM CSCs. We further tested whether the GBM CSC specific markers are associated with the cancer stemness using primarily cultured patient-derived GBM cells. However, results consistently indicated that GBM cells with or without GD2 and CD90 had similar in vitro sphere formation capacity, a functional characteristics of CSCs. Therefore, GD2 and CD90, GBM specific surface markers, might not be used as specific therapeutic targets for GBM CSCs, although they could have other clinical utilities.
Glioblastoma multiforme (GBM) is classified as World Health Organization (WHO) grade IV brain tumors. They are the most common and most malignant tumor of the adult human central nervous system. GBM patients have a poor prognosis with the median survival of approximately below 1 year [1]. The poor prognosis in GBM patients is mainly due to the resistance to standard therapies, such as chemotherapy and radiation [2]. Recently, stem like cells were reported to increase the GBM resistance to chemotherapy and radiotherapy, and expectedly caused GBM recurrence [3, 4, 5].
In the nervous system, stem cells have the properties of self-renewal for expansion and maintenance [6]. These neural stem cells (NSCs) are differentiated into neurons, astrocytes, and oligodendrocyte in nervous system development [7]. However, the sub-population of NSCs with tumor initiating abilities could play a major role in GBM development. In fact, cancer cells displaying NSCs properties and tumor-initiating abilities comprise a small proportion in GBM [8, 9]. Cancer initiating cells known as cancer stem cells (CSCs) especially represent a subpopulation of cancer cells, and are responsible for the maintenance and growth of tumors. Recently, CSCs were identified in various cancers such as some leukemias, breast cancer, gastric cancer and brain tumor [10, 11, 12, 13]. These GBM CSCs closely resemble normal NSCs that they share the properties of self-renewal and differentiation [14, 15]. However, CSCs are distinguished by increased proliferation kinetics and abnormal differentiation [12, 16, 17, 18]. Moreover, CSCs may cause relapse and metastasis by giving rise to new tumors.
Therefore, the specific therapies targeting CSCs is critically required to overcome therapeutic resistances of GBM. Unfortunately, currently little is known about specific markers of CSCs. Although the protein levels of the CD133 cell surface marker determines success or failure of in vitro culture and in vivo tumor formation, they are also expressed in normal NSCs [11, 15, 16, 19, 20]. So, the critical goal of therapeutic development for GBM patients is to identify the specific targeted markers for GBM CSCs that are distinct from normal NSCs. Thus we performed this study to identify the specific cell surface markers of CSCs distinct from human NSCs as a GBM therapeutic targeting markers.
Surgical specimens were mechanically minced and placed in enzyme mixture solution containing papain (10 unit/ml, Sigma, St. Louis, MO, USA), DNase I (0.1 mg/ml, Roche, Mannheim, Germany), and D,L-cystein (4 mg/ml, Sigma) for 15-30 minutes at 37℃. Dissociated cells were cultured in DMEM/F12 media with % B27 (Invitrogen, Carlsbad, CA, USA), 1% penicillin/streptomycin cocktail (Invitrogen), epidermal growth factor (50 ng/ml, R&D Systems, Minneapolis, MN, USA), basic fibroblast growth factor (50 ng/ml, R&D Systems) for neurosphere culture. Alternatively, adherent culture was performed with above culture media with 0.5% fetal bovine serum (Invitrogen).
For immunocytochemistry (ICC) analysis, both 682, 779, 464T, and 532T cells (3×103 cells/well) were cultured on Nunc Lab-Tek II Chamber Slide System (Thermo Scientific, Waltham, MA, USA). The cells were fixed by 4% paraformaldehyde, permeabilized in 0.5% Triton X-100, and then blocked with 1% bovine serum albumin (Santa Cruz Biotechnology, Santa Cruz, CA, USA). Primary antibodies against Sox2 and nestin (Upstate/Millipore, Billerica, MA, USA) and Alexa-flour 488 dye-conjugated secondary antibodies (Invitrogen) were utilized. Nuclei were stained by 4',6-diamidino-2-pheylindole (DAPI, Invitrogen).
For tumor formation, we performed the intracranial injection using anesthetized BALB/c-nu mice of 6 week old. To establish these orthotopic models, the each cells of NSCs and GBM (2×105/5 µl Hank's balanced salt solution, Gibco, Eggenstein, Germany) were stereotypically injected into the left striata of mice (co-ordinates; anterior/posterior, +0.5 mm; medial/lateral, +1.7 mm, dorsal/ventral, -3.2 mm from Bregma). For tumor volume measurement the brain slices were fixed in 10% formalin/phosphate buffered saline, and embedded in paraffin. And then the paraffin blocks were sectioned into 4-µm sections, and stained with hematoxylin and eosin.
Cells were characterized using BD Lyoplate Human Cell Surface Markers Screening Panels (cat no. 560747, BD Biosciences, San Jose, CA, USA). The panels contain 242 antibodies and correspondent isotype controls in three 96-well plates, and the staining was done according to manufacturer's instruction. Two hundred forty-two antibodies for cell surface marker screening were listed in Table 1. The incubation with primary antibodies was done in a concentration of 0.5 µg per test, and secondary antibodies were used species-specific Alexa 647 (Invitrogen). The measurement was done using flow cytometry, BD Caliber & LSR II @ SNU fluorescence activated cell sorting (FACS) room, and data analysis was done by using BD Bioscience (San Jose, CA, USA).
The cultured cells were enzymatically dissociated into single cell suspensions, and seeded into 96-well plates with various seeding densities of 20, 50, 100, 200, and 500 cell per well. After seeding, the plates were incubated at 37℃ for 2-3 weeks. Wells without sphere formation was analyzed, and tumor sphere frequency was calculated using the Extreme Limiting Dilution Analysis (http://bioinf.wehi.edu.au/software/elda/index.html). The P-value was determined by chi-square test compared with control group, and P<0.05 was considered as statistically significant.
We have previously established the culture method for human multipotent neural cells, as well as the sphere culture method for retaining the stemness of GBM cells [21]. Surgical samples from temporal lobe epilepsy patients were cultured under the neurosphere culture conditions without serum. Subsequently, the NSCs, 682, and 779 were identified to express stem cell markers by ICC. The results indicated that stem cell markers, Sox2 and nestin were detected in NSCs, such as 682 and 779 (Fig. 1, two left columns). Furthermore, we previously reported that GBM patients-derived cells from surgical specimens were cultured in neurosphere culture conditions without serum for CSCs [20, 22]. The GBM cells, 532T and 464T were also confirmed for stem cell markers at the protein level. Sox2 and nestin were clearly detected in 532T and 464T cells (Fig. 1, two right columns). We additionally showed that Sox2 and nestin were predominantly expressed in nucleus and cytoplasm in both NSCs and GBM cells.
We injected animals with NSCs and GBM cells that retained stemness, and identified subsequent tumor formation. The mouse brains injected with 682 and 779 NSCs did not generate tumor mass (Fig. 2, two left columns, upper panels), but 464T and 532T GBM cells generated tumor mass within the brain (Fig. 2, two right columns, upper panels). Additionally, we observed cell proliferation in all fixed mouse brains by tissue staining with the proliferating cell nuclear antigen (PCNA) antibody. The result showed that the tumor cells generated after injection with 464T and 532T were stained with the PCNA antibodies (Fig. 2, two right columns, lower panels), but the mouse brains injected with 682 and 779 were unstained (Fig. 2, two left columns, lower panels). Active proliferation of the tumor cells only generated by injection with 464T and 532T GBM cells.
Differentially overexpressed stem cell markers in GBM cells were identified by staining 779, 682, 464T, and 532T cells with 242 cell surface markers antibodies. The results obtained in 682 and 779 NSCs cells, or in 464T and 532T GBM cells were displayed in Fig. 3. All data were normalized to the average value with buffer solution. We selected 11 molecules including GD2, CD98, CD91, CD90, CD81, CD59, CD57, CD56, CD47, CD151, and CD146, which showed positive expression of >50% in 464T and 532T GBM. The 11 selected molecules were quantified by fold changes to the corresponding average value of NSCs. The results showed that the protein levels of GD2 and CD90 were increased 10 fold and 9.8 fold respectively, in GBM cells than NSCs (Fig. 4). However, 9 molecules, i.e., CD98, CD91, CD81, CD59, CD57, CD56, CD47, CD151, and CD146 had significant differences of positive expression in GBM cells compared with NSCs.
Cancer contains its own stem cells for maintenance and expansion of the tumor. CSCs generate tumor by self-renewal and differentiation into multiple cell types, and comprise only a small proportion of cancer cells. Recently, sphere culture was introduced in various stem cell researches including nerve and prostate [23, 24]. Sphere formation is especially excellent to enrich the CSCs subpopulations. We performed limiting dilution analysis by measuring the frequency of tumor sphere formation to identify the self-renewing capacity of CSCs.
Firstly, to determine whether the overexpression of GD2 and CD90 in cancer cells have characteristics of cancer stemness, we performed the cell sorting of tumor cells with or without the protein expression levels of GD2 and CD90, respectively in 464T GBM cells. The results indicated that the cells with or without GD2 and CD90 were 10% and 5%, respectively, in 464T (Fig. 5A). We then immediately performed a limiting dilution assay using these sorted cells for sphere formation. The cells with GD2 were not significantly different compared with the cells without GD2 (P=0.073) (Fig. 5C). Additionally, the cells with or without CD90 were also not significantly different (P=0.165) (Fig. 5D).
GBM comprises 55% of primary brain tumors, and it is well known as the primary malignant brain tumor [25]. The prognosis of GBM patients is very poor, and recurrence is common [1, 2]. According to recent reports, one of the causes of GBM recurrence may be CSCs with self-renewal potential, which exists as a small fraction of GBM. CD133 cell surface marker-positive cells, in comparison to CD133-negative cells, were shown to contribute to anti-cancer drug and radiation resistance in several studies on brain tumors. Singh et al. [11, 19] showed that the FASC sorted CD133-positive cancer cells drive tumor formation, but CD133-negative cancer cells fail to generate tumor mass in mouse brains. These findings suggest that CSCs have a critical role in tumor formation, as well as tumor recurrence by increasing resistance to strong external stresses. Many researchers have recognized the importance of specific markers of CSCs for tumor-initiating cells target therapy. However, CSCs share properties of normal NSCs, such as long term self-renewal and the ability to differentiate into the various neural cell lineages. CD133 plays a critical role in tumor formation and resistance to various stresses in cancer cells, but it is overexpressed in normal NSCs, as well as CSCs. So, we attempted to identify specific markers that are differentially expressed in CSCs as compared to NSCs in this study. In this study, we identified that GD2 and CD90 were significantly increased in GBM cells than NSC cells (Fig. 4).
GD2 disialoganglioside is a sialic-containing glycosphingolipid, which is expressed primarily on the cell membrane, and restricted to neurons in normal tissue [26]. However, high expression of GD2 has been found in neuroblastoma, melanoma, bone, small cell lung cancers, and soft-tissue sarcoma, as well as brain tumors [27, 28, 29, 30, 31, 32]. In addition, GD2 is overexpressed in the malignant tumors and exhibits molecular and functional properties of CSCs in breast cancer cell. Battula et al. [33] reported that ganglioside GD2 was identified in breast CSCs and promotes tumorigenesis. According to their research, the GD2-positive cells isolated by FACS sorted steps formed 2 fold higher single cell-derived mammospheres as compared with GD2-negative cells. Moreover, the in vivo limiting dilution assay to determine the tumor initiating potential of GD2-positive cells was used for identification of tumor generation by sorted GD2-positive and GD2-negative cells. GD2-positive cells generated a maximum 5 fold more tumor compared with the GD2-negative cells. Their results suggested that GD2 is a marker of cells capable of initiating tumors at a higher frequency than GD2-negative cells in breast cancer. So, this overexpression of GD2 is taken notice as a therapeutic marker in a wide spectrum of tumors [34, 35]. Currently, several GD2 targeting antibodies have already been developed and studied by many researchers for molecular targeting therapy, and are in clinical trial for neuroblastoma and metastatic melanoma [36, 37].
CD90 is a heavily glycosylated, glycophosphatidylinositol anchored cell membrane protein. It is already known as a major marker for human stem cells in liver CSCs, as well as various CSCs, such as hematopoietic and mesenchymal stem cells [38, 39, 40, 41]. According to recent studies, while CD90 is hardly expressed in the normal brain and most low grade astrocytomas, it is dramatically increased in high grade (WHO grade III and IV) glioma [42, 43]. Thus CD90 is reported by Yang et al. [40] that it is a unique surface marker for high grade glioma CSCs. Yang et al. [40] reported the significance of CD90-positive CSCs in liver cancer. They showed the sorted CD90-positive cells from 6 HCC cell lines, i.e., HepG2, Hep3B, PLC, Huh7, MHCC97L, and MHCC97H generated tumor nodules in nude mice. Conversely, the CD90-negative cells from all cell lines did not induce tumor formation in mice. Their results that CD90-positive cells positively correlated with tumorigenicity, revealed that CD90 is a potential marker for liver CSCs.
These many reports strongly suggest that GD2 and CD90 may be the potential therapeutic targets for CSCs. In addition, we also obtained the results that GD2 and CD90 may be the potential specific targets for GBM CSCs, because they were prominently overexpressed in GBM CSCs (Fig. 4). So, we performed in vitro experiments to determine whether cells sorted with the GD2 and CD90 antibodies had the characteristic of CSCs since the method was particularly appropriate to observe the characteristics of cancer stemness at the single cell level from heterogeneous GBM patient-derived cells (Fig. 5). To elucidate our theories, we choose the limiting dilution analysis to determine the frequency of cells with a particular function that were present in a heterogeneous cell population. However, we could not find that GD2- or CD90-positive cells have in vitro sphere formation capacity, a functional characteristics of CSCs distinct from NSCs (Fig. 5C, D). As the final outcome, this study indicated that GD2 and CD90 cell surface markers were highly expressed in GBM cells in contrast to NSCs, but did not have a particular characteristic of GBM CSCs. Therefore, we think that the specific overexpressed GD2 and CD90 in GBM cells might not be uses as critical therapeutic targets for GBM CSCs, although they could have other clinical utilities.
Figures and Tables
Fig. 1
Sox2 and nestin were highly regulated in both normal neural stem cells (NSCs) and glioblastoma multiforme (GBM) cells. 464T and 532T GBM cells, as well as 682 and 779 normal NSCs were stained by anti-Sox2 (upper, green) and anti-nestin (lower, green) antibodies for immunocytochemistry. Nuclei of all cells were stained by DAPI (blue).
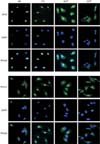
Fig. 2
Glioblastoma multiforme cells unlike neural stem cells generated tumor mass in orthotopic brain xenograft models. Hematoxylin and eosin (H&E) and immunohistochemistry staining for proliferating cell nuclear antigen (PCNA) expression were performed in representative whole brains of the orthotopic xenograft. Bar chart summary of average staining intensity of PCNA in 3 randomly selected hot spot regions (*P<0.05).
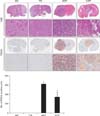
Fig. 3
Cell surface markers of 242 kinds were analyzed in normal neural stem cells (NSCs) and glioblastoma multiforme (GBM) cells. 464T (B) and 532T (D) GBM cells, as well as 682 (A) and 779 (C) normal NSCs were stained by BD Lyoplate Human Cell Surface Marker Screening Panel containg 242 antibodies. All cells were counted by flow cytometer BD Caliber & LSR II. Data were analyzed by BD FACSDive software.
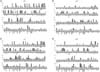
Fig. 4
Positive expression of GD2 and CD90 were significantly increased in glioblastoma multiforme (GBM) cells than neural stem cells (NSCs). BD Lyoplate Human Cell Surface Marker Screening showing high regulated markers of >50% were selected in GBM cells, and analyzed with the expressed changes in NSCs. Data was expressed by the fold changes of each value in GBM cells as compared with NSCs for the selected 11 markers.
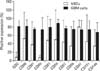
Fig. 5
GBM cells with high levels of GD2 or CD90 protein do not have characteristics of cancer stemness. 464T cells were stained by anti-GD2 (A) and anti-CD90 (B) antibodies. The high-regulated and low-regulated cells of GD2 or CD90, were sorted by fluorescence activated cell sorting analysis. The sorted cells and parent cells were tested for the ability of neurosphere formation according to the expressed levels of GD2 (C) or CD90 (D) by the limiting dilution assay.
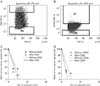
Table 1
List of 242 cell surface markers in BD Lyoplate Human Cell Surface Markers Screening Panels
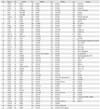
Acknowledgements
This research was supported by Basic Science Research Program through the National Research Foundation of Korea (NRF) funded by the Ministry of Education (2013R1A1A2058169).
References
1. Kohler BA, Ward E, McCarthy BJ, Schymura MJ, Ries LA, Eheman C, Jemal A, Anderson RN, Ajani UA, Edwards BK. Annual report to the nation on the status of cancer, 1975-2007, featuring tumors of the brain and other nervous system. J Natl Cancer Inst. 2011; 103:714–736.
2. Mangum R, Nakano I. Glioma stem cells and their therapy resistance. J Carcinog Mutagen. 2011; S1:002.
3. Kang MK, Kang SK. Tumorigenesis of chemotherapeutic drug-resistant cancer stem-like cells in brain glioma. Stem Cells Dev. 2007; 16:837–847.
4. Bao S, Wu Q, McLendon RE, Hao Y, Shi Q, Hjelmeland AB, Dewhirst MW, Bigner DD, Rich JN. Glioma stem cells promote radioresistance by preferential activation of the DNA damage response. Nature. 2006; 444:756–760.
5. Lomonaco SL, Finniss S, Xiang C, Decarvalho A, Umansky F, Kalkanis SN, Mikkelsen T, Brodie C. The induction of autophagy by gamma-radiation contributes to the radioresistance of glioma stem cells. Int J Cancer. 2009; 125:717–722.
6. McKay R. Stem cells in the central nervous system. Science. 1997; 276:66–71.
7. Temple S. The development of neural stem cells. Nature. 2001; 414:112–117.
8. Goffart N, Kroonen J, Rogister B. Glioblastoma-initiating cells: relationship with neural stem cells and the micro-environment. Cancers (Basel). 2013; 5:1049–1071.
9. Stiles CD, Rowitch DH. Glioma stem cells: a midterm exam. Neuron. 2008; 58:832–846.
10. Reya T, Morrison SJ, Clarke MF, Weissman IL. Stem cells, cancer, and cancer stem cells. Nature. 2001; 414:105–111.
11. Singh SK, Clarke ID, Hide T, Dirks PB. Cancer stem cells in nervous system tumors. Oncogene. 2004; 23:7267–7273.
12. Nicolis SK. Cancer stem cells and "stemness" genes in neuro-oncology. Neurobiol Dis. 2007; 25:217–229.
13. Han ME, Oh SO. Gastric stem cells and gastric cancer stem cells. Anat Cell Biol. 2013; 46:8–18.
14. Ignatova TN, Kukekov VG, Laywell ED, Suslov ON, Vrionis FD, Steindler DA. Human cortical glial tumors contain neural stem-like cells expressing astroglial and neuronal markers in vitro. Glia. 2002; 39:193–206.
15. Hemmati HD, Nakano I, Lazareff JA, Masterman-Smith M, Geschwind DH, Bronner-Fraser M, Kornblum HI. Cancerous stem cells can arise from pediatric brain tumors. Proc Natl Acad Sci U S A. 2003; 100:15178–15183.
16. Singh SK, Clarke ID, Terasaki M, Bonn VE, Hawkins C, Squire J, Dirks PB. Identification of a cancer stem cell in human brain tumors. Cancer Res. 2003; 63:5821–5828.
17. Galli R, Binda E, Orfanelli U, Cipelletti B, Gritti A, De Vitis S, Fiocco R, Foroni C, Dimeco F, Vescovi A. Isolation and characterization of tumorigenic, stem-like neural precursors from human glioblastoma. Cancer Res. 2004; 64:7011–7021.
18. Tunici P, Bissola L, Lualdi E, Pollo B, Cajola L, Broggi G, Sozzi G, Finocchiaro G. Genetic alterations and in vivo tumorigenicity of neurospheres derived from an adult glioblastoma. Mol Cancer. 2004; 3:25.
19. Singh SK, Hawkins C, Clarke ID, Squire JA, Bayani J, Hide T, Henkelman RM, Cusimano MD, Dirks PB. Identification of human brain tumour initiating cells. Nature. 2004; 432:396–401.
20. Joo KM, Kim SY, Jin X, Song SY, Kong DS, Lee JI, Jeon JW, Kim MH, Kang BG, Jung Y, Jin J, Hong SC, Park WY, Lee DS, Kim H, Nam DH. Clinical and biological implications of CD133-positive and CD133-negative cells in glioblastomas. Lab Invest. 2008; 88:808–815.
21. Joo KM, Kang BG, Yeon JY, Cho YJ, An JY, Song HS, Won JH, Kim SJ, Hong SC, Nam DH. Experimental and clinical factors influencing long-term stable in vitro expansion of multipotent neural cells from human adult temporal lobes. Exp Neurol. 2013; 240:168–177.
22. Kim KH, Seol HJ, Kim EH, Rheey J, Jin HJ, Lee Y, Joo KM, Lee J, Nam DH. Wnt/beta-catenin signaling is a key downstream mediator of MET signaling in glioblastoma stem cells. Neuro Oncol. 2013; 15:161–171.
23. Bez A, Corsini E, Curti D, Biggiogera M, Colombo A, Nicosia RF, Pagano SF, Parati EA. Neurosphere and neurosphere-forming cells: morphological and ultrastructural characterization. Brain Res. 2003; 993:18–29.
24. Shi X, Gipp J, Bushman W. Anchorage-independent culture maintains prostate stem cells. Dev Biol. 2007; 312:396–406.
25. Legler JM, Ries LA, Smith MA, Warren JL, Heineman EF, Kaplan RS, Linet MS. Cancer surveillance series [corrected]: brain and other central nervous system cancers: recent trends in incidence and mortality. J Natl Cancer Inst. 1999; 91:1382–1390.
26. Ritter G, Livingston PO. Ganglioside antigens expressed by human cancer cells. Semin Cancer Biol. 1991; 2:401–409.
27. Chang HR, Cordon-Cardo C, Houghton AN, Cheung NK, Brennan MF. Expression of disialogangliosides GD2 and GD3 on human soft tissue sarcomas. Cancer. 1992; 70:633–638.
28. Heiner JP, Miraldi F, Kallick S, Makley J, Neely J, Smith-Mensah WH, Cheung NK. Localization of GD2-specific monoclonal antibody 3F8 in human osteosarcoma. Cancer Res. 1987; 47:5377–5381.
29. Cheung NK, Saarinen UM, Neely JE, Landmeier B, Donovan D, Coccia PF. Monoclonal antibodies to a glycolipid antigen on human neuroblastoma cells. Cancer Res. 1985; 45:2642–2649.
30. Mujoo K, Cheresh DA, Yang HM, Reisfeld RA. Disialoganglioside GD2 on human neuroblastoma cells: target antigen for monoclonal antibody-mediated cytolysis and suppression of tumor growth. Cancer Res. 1987; 47:1098–1104.
31. Wu ZL, Schwartz E, Seeger R, Ladisch S. Expression of GD2 ganglioside by untreated primary human neuroblastomas. Cancer Res. 1986; 46:440–443.
32. Tsuchida T, Saxton RE, Morton DL, Irie RF. Gangliosides of human melanoma. J Natl Cancer Inst. 1987; 78:45–54.
33. Battula VL, Shi Y, Evans KW, Wang RY, Spaeth EL, Jacamo RO, Guerra R, Sahin AA, Marini FC, Hortobagyi G, Mani SA, Andreeff M. Ganglioside GD2 identifies breast cancer stem cells and promotes tumorigenesis. J Clin Invest. 2012; 122:2066–2078.
34. Yu AL, Uttenreuther-Fischer MM, Huang CS, Tsui CC, Gillies SD, Reisfeld RA, Kung FH. Phase I trial of a human-mouse chimeric anti-disialoganglioside monoclonal antibody ch14.18 in patients with refractory neuroblastoma and osteosarcoma. J Clin Oncol. 1998; 16:2169–2180.
35. Zeytin HE, Tripathi PK, Bhattacharya-Chatterjee M, Foon KA, Chatterjee SK. Construction and characterization of DNA vaccines encoding the single-chain variable fragment of the anti-idiotype antibody 1A7 mimicking the tumor-associated antigen disialoganglioside GD2. Cancer Gene Ther. 2000; 7:1426–1436.
36. Yu AL, Gilman AL, Ozkaynak MF, London WB, Kreissman SG, Chen HX, Smith M, Anderson B, Villablanca JG, Matthay KK, Shimada H, Grupp SA, Seeger R, Reynolds CP, Buxton A, Reisfeld RA, Gillies SD, Cohn SL, Maris JM, Sondel PM. Anti-GD2 antibody with GM-CSF, interleukin-2, and isotretinoin for neuroblastoma. N Engl J Med. 2010; 363:1324–1334.
37. Simon T, Hero B, Faldum A, Handgretinger R, Schrappe M, Niethammer D, Berthold F. Infants with stage 4 neuroblastoma: the impact of the chimeric anti-GD2-antibody ch14.18 consolidation therapy. Klin Padiatr. 2005; 217:147–152.
38. Dennis JE, Esterly K, Awadallah A, Parrish CR, Poynter GM, Goltry KL. Clinical-scale expansion of a mixed population of bone-marrow-derived stem and progenitor cells for potential use in bone-tissue regeneration. Stem Cells. 2007; 25:2575–2582.
39. Baum CM, Weissman IL, Tsukamoto AS, Buckle AM, Peault B. Isolation of a candidate human hematopoietic stem-cell population. Proc Natl Acad Sci U S A. 1992; 89:2804–2808.
40. Yang ZF, Ho DW, Ng MN, Lau CK, Yu WC, Ngai P, Chu PW, Lam CT, Poon RT, Fan ST. Significance of CD90+ cancer stem cells in human liver cancer. Cancer Cell. 2008; 13:153–166.
41. Bayati V, Hashemitabar M, Gazor R, Nejatbakhsh R, Bijannejad D. Expression of surface markers and myogenic potential of rat bone marrow- and adipose-derived stem cells: a comparative study. Anat Cell Biol. 2013; 46:113–121.
42. He J, Liu Y, Zhu T, Zhu J, Dimeco F, Vescovi AL, Heth JA, Muraszko KM, Fan X, Lubman DM. CD90 is identified as a candidate marker for cancer stem cells in primary high-grade gliomas using tissue microarrays. Mol Cell Proteomics. 2012; 11:M111.010744.
43. Parry PV, Engh JA. CD90 is identified as a marker for cancer stem cells in high-grade gliomas using tissue microarrays. Neurosurgery. 2012; 70:N23–N24.