Abstract
Objective
To describe an acute and chronic murine model of allergic asthma with Tp extract with no systemic sensitization and no use of adjuvant.
Methods
Mites from dust sample were cultured and a raw extract was produced. Female BALB/c mice (6-8 weeks) were challenged intranasally with Tp extract or Dulbecco's phosphate-buffered saline, for 10 consecutive days (acute protocol) or for 6 weeks (chronic protocol). Twenty-four hours after the last intranasal challenge, bronchoalveolar lavage fluid (BALF) was performed for total and differential cells count, cytokine analysis, and eosinophil peroxidase activity. Lung tissue was also removed for histopathologic analysis.
Results
Tp extract has shown a significant increase in total cells count from BALF as well as an increase in absolute eosinophils count, eosinophil peroxidase activity, interleukin (IL)-5 and IL-13 levels, in both acute and chronic protocols. Peribronchovascular infiltrate, goblet cells hyperplasia and collagen deposition were shown in the airways of acute and chronic Tp-exposed mice.
Conclusion
Our data suggest that the intranasal exposure to Tp extract, with no systemic sensitization and no use of adjuvants, induces a robust allergic inflammation in the lungs of mice, in both acute and chronic models. Our Tp extract seems to be a potent allergen extract which may be used in asthma model studies.
Asthma is a highly prevalent worldwide health problem, characterized by chronic airway inflammation and variable airflow obstruction, resulting in high morbidity to the patients [1]. Asthma is a complex disease associated with genetics and environmental factors in which many cell types and cytokines are involved in the mechanism of disease [23]. T-helper 2 (Th2) lymphocytes modulate inflammatory response in the lungs and stimulate secretion of a range of cytokines, such as interleukin (IL)-4, IL-5, and IL-13 [345]. This scenario promotes the recruitment and activation of eosinophils, mast cells, basophils, and goblet cells hyperplasia in the airways [67].
Mice have been largely used as allergic pulmonary inflammatory models, and are still necessary to better understand mechanisms of disease and for testing new therapies and primary preventive treatments. The original and more classical model used to induce allergic pulmonary response in mice is characterized by systemic sensitization with adjuvant and acute airway challenge with ovalbumin (OVA). In mice, OVA cause a robust Th2 response, but very seldom induce an allergic airway sensitization in human asthma. Moreover, OVA develop immune tolerance in chronic murine models [8910]. Hence, in the last decade, extract of house dust mites (HDMs) has been shown to be a more adequate alternative, particularly regarding to translational purposes. Unlike OVA, mites are a major natural source of allergens with the ability to cause allergic diseases, and have shown to sensitize mice with no adjuvant use, including chronic protocols [1112].
Storage mites are an important source of aeroallergens, found throughout homes around the world, which may cause allergic diseases, such as asthma and rhinitis in many individuals [1314]. Storage mites have been recognized as a risk factor to induce allergic diseases in humans, frequently sensitizing individuals after occupational ingestion of contaminated foods with these mites, which may lead to anaphylaxis in some cases [13151617]. Tyrophagus putrescentiae (T. putrescentiae, Tp) is found worldwide, particularly in contaminated food such as mushrooms, wheat flour, wheat germ, animal chow, ham, cheese and cereal. T. putrescentiae is a storage mite that has deserved especial attention as a major cause of allergic diseases, including urban settings [13151718]. One recent experimental study has shown that intraperitoneal sensitization followed by intratracheal challenge with a crude extract of Tp, administered with aluminum hydroxide, induced airway allergic inflammation in BALB/c mice [13]. However, the description of the production of Tp extract has not been described and less artificial sensitization (with no adjuvant use and limited to intranasal route) and chronic protocols were not reported. Hence, the aim of our study is: (1) to produce a Tp extract; (2) to evaluate whether this extract induces acute and chronic allergic pulmonary response in mice, similarly to well established previous existing protocols with HDM (which are more similar to the disease in humans), with no systemic sensitization and no adjuvant use, eventually suggesting a standardized protocol with an extract of Tp.
Thirty-two female BALB/c mice (6-8 weeks of age) were used, obtained from our animal center (CeMBE, Pontifícia Universidade Católica do Rio Grande do Sul [PUCRS], Porto Alegre, Brazil). Animals were housed in cages and maintained on a 12/12-hour light/dark cycle, with food and water ad libitum.
Mites were collected in a dust sample and cultured in a medium with Sacaromices cereviseae, at 25℃ and controlled humidity. T. putrescentiae were separated from their food (S. cereviseae) using a modified Berlese-Tulgreen apparatus and were subsequently stored in 70% alcohol. Mites were removed from 70% alcohol by centrifugation, and then digested in liquid nitrogen. Lipids were then removed in ethyl ether for 3 hours under stirring. Ether was after removed and the extract was dried for total evaporation, resuspended in Dulbecco's phosphate-buffered saline (DPBS), and again centrifuged. The supernatant was separated and protein concentration present on extract was determined using a Qubit Protein Assay Kit and the Qubit Fluorometer (Invitrogen, Eugene, OR, USA). The extract was kept in -80℃.
Animals were anesthetized with inhaled isoflurane (Cristália, São Paulo, Brazil). T. putrescentiae extract was administered intranasally (34.5 µg the protein extract per animal) once a day, for 10 consecutive days, in the acute protocol (Fig. 1A) and challenged 5 days a week, during 6 consecutive weeks, in the chronic protocol (Fig. 1B). In both protocols, the protein concentration of the extract used was 2.3 mg/mL. We used negative controls that received only DPBS intranasally, instead of Tp extract.
Bronchoalveolar lavage fluid (BALF) was performed trough tracheotomy, after anesthesia with ketamine (0.4 mg/g) and xylazine (0.2 mg/g) intraperitoneal injection. DPBS solution (1 mL) was instilled intratracheally and immediately aspirated after two consecutive instillations. After the procedure, animals were euthanized through exsanguination by cardiac puncture, according to institutional ethical rules. Lungs were then removed for histological analysis.
BALF was centrifuged at 2.000 rpm, for 4 minutes, and the supernatant was removed and frozen at -80℃, for cytokines analysis and eosinophilic peroxidase (EPO) activity. The pellet was resuspended in 350 µL of DPBS, and total cells count (TCC) and cell viability were determined by trypan blue exclusion test with a Neubauer chamber (BOECO, Hamburg, Germany). For the differential cell analysis, BALF suspension (80 µL) was placed in a cytospin centrifuge at 500 rpm, for 5 minutes. The cells were analyzed for their morphology in slides stained with Panótico Rápido (Laborclin, Pinhais, Brazil). Cell types observed under optical microscopy were expressed after counting 400 cells.
IL-5 and IL-13 levels were analyzed through CBA mouse Flex Set Kit (BD Biosciences, San Jose, CA, USA) from the supernatants of BALF by flow cytometer (BD FACSCanto II, BD Biosciences), according to the manufacturer's instructions. EPO activity was measured from BALF and was determined through colorimetric assay as described by Silveira et al. [19].
Lungs were perfused with 10% buffered formalin on a gravity column (20 mm Hg). Tissue specimens were embedded in paraffin blocks, cut into 4-µm sections, stained with H&E and Alcian Blue were examined by light microscopy. Slides stained with Picrosirius Red were observed under polarized light microscopy.
Statistical analysis was performed using GraphPad Prism ver. 4.0 (GraphPad Software Inc., La Jolla, CA, USA). Data were analyzed with Mann-Whitney test for nonparametric samples. Differences of p < 0.05 were considered statistically significant, and data are expressed as median values and interquartile range (25-75%).
Firstly, we investigated the effect of acute intranasal challenge with Tp extract on total and differential cells count in BALF. The group acutely exposed to the Tp extract showed a significant increase in TCC (p < 0.001) and absolute eosinophils count (p < 0.001) compared to DPBS group (Fig. 2A, B). Moreover, differential cells count were also significantly different in neutrophils (p < 0.001), lymphocytes (p < 0.01) and macrophages (p < 0.001) count (Fig. 2C-E). Mice exposed to acute Tp extract showed a significant increase in the EPO activity (p < 0.01) in relation to the DPBS group (Fig. 2F).
Next, we evaluated the effect of the chronic intranasal challenge with Tp extract. Similarly to what was observed in the acute Tp protocol, chronic Tp exposure showed a significant increase of TCC (p < 0.01) compared to the DPBS group (Fig. 3A), as well as with eosinophils (p < 0.01), neutrophils (p < 0.01), lymphocytes (p < 0.01), and macrophages (p < 0.001) in BALF, as shown in Fig. 3B-E. EPO activity was also significantly elevated (p < 0.01) in the Tp group (Fig. 3F).
We also showed that IL-5 and IL-13 levels were significantly increased in BALF (p < 0.05 and p < 0.01, respectively) of mice challenged with acute Tp protocol when compared to the DPBS group (Fig. 4A, B). In addition, Fig. 4C and D showed that chronic Tp protocol had increased levels of IL-5 (p < 0.05) and IL-13 (p < 0.01) compared to the DPBS group.
Finally, we observed the presence of peribronchial cells infiltrating the airways from acute Tp group (Fig. 5A, B) when compared to the DPBS group. In addition, acute Tp group showed airways goblet cells hyperplasia (Fig. 5E, F), and the presence of collagen deposition around the airways when stained with Picrosirius Red (Fig. 5I. J). Animals exposed to chronic Tp extract showed an intense infiltration of peribronchial inflammatory cells in the airways and also presence of goblet cells in the airway epithelia (Fig. 5C, D, G, H, respectively), and collagen deposition in the airways (Fig. 5K, L).
Asthma is a chronic airway inflammatory disease that is mostly characterized by aeroallergen sensitization, with subsequent allergic immune response. Animal models are still essential for the understanding of the disease, their mechanisms, and to test new asthma therapies. Murine models of asthma have been modified to become more similar to models of asthma in humans [8]. In this study, we have used a different mite (T. putrescentiae) for sensitizing mice in an asthma model. This mite has been associated with allergic diseases in rural and urban settings [20].
We demonstrated that the intranasal exposure to natural antigens of Tp extract, produced noncommercially, was able to induce a pulmonary allergic inflammatory response in acute (10 days) and chronic (6 weeks) asthma protocols, with no need of prior systemic allergic sensitization, which is frequently used through intraperitoneal or subcutaneous injection with adjuvant, in order to increase Th2 immune response. In a recent study, Liao et al. [13] showed that the use of intraperitoneal sensitization with a crude extract of Tp, with aluminum hydroxide, followed by Tp intratracheal challenge, also induces airway inflammation in BALB/c mice. In our study, we have successfully tested the nasal route for Tp delivery (both for sensitization and challenge), in a less artificial asthma protocol (with no use of intraperitoneal sensitization with adjuvant), including also a chronic protocol. In a similar protocol of our study, Johnson et al. [21] demonstrated that continuous intranasal exposure to a commercial HDM extract leads to airway eosinophilic inflammation, elevated levels from Th2 cytokines and structural remodeling, with goblet cells hyperplasia and increase in the collagen deposition, in agreement with our results. The animals that received Tp extract (acute and chronic protocols) showed increased TCC and eosinophils, compared to the DPBS group in BALF. In the chronic protocol, the cellular response using our extract of Tp were similar to those found in other chronic HDM asthma models, in which eosinophils were the predominant cells in BALF [2122]. The low number of neutrophils in our model seems to be the result of low contamination of bacterial products from our Tp extract.
In both acute and chronic Tp protocols, increased EPO activity in BALF was observed, supporting the eosinophilic lung response developed in this model. Previous studies with mice exposed intranasally to commercially purified HDM extract showed a strong Th2 inflammation, with eosinophil increase and higher levels from several cytokines such as IL-4, IL-5, IL-13, granulocyte-macrophage colony-stimulating factor in BALF [823], with airway remodeling associated to increased bronchial hyperactivity [921]. We evaluated the levels from two important Th2 cytokines, showing that IL-5 and IL-13 are increased in our models.
Experimental studies have shown that the intranasal continuous exposure (chronic) to HDM results in a robust Th2 airway inflammation, with increased in the number of eosinophils, presence of massive infiltrate peribronchovascular, increased production of mucus and deposition of collagen in the airway [92124]. The airway remodeling involves several pathophysiological factors, as epithelial changes, thickening of the smooth muscle, increased sub epithelial fibrosis, hyperplasia of goblet cells and vascular changes around the airway [2526]. In our lung tissues specimens the presence of granulocytes and goblet cells hyperplasia in the animals exposed to Tp was clearly demonstrated. Increased collagen deposition around the airways is also a central feature of chronic remodeling [25]. However, in both acute and chronic protocols, we have shown deposition of collagen around the airways. The understanding of the temporal and type of collagen deposition in the airways of murine asthma models is not clear and has to be further studied. The relationships between airway hyperresponsiveness, inflammation and remodeling remain poorly understood. Saglani et al. [27], in a neonatal-induced mouse model of pulmonary allergic disease, demonstrated an increased peribronchial collagen deposition after exposure to intranasal HDM, and this alteration appeared between 2 and 4 weeks after the beginning of allergen challenge at day 3 of life. On the other hand, in agreement with our results, Johnson et al. [21] demonstrated in one adult HDM model of murine asthma that airways remodeling was not observed until 5 weeks after allergen exposure. Airways collagen deposition in asthma is still poorly understood and this question needs to be better addressed.
One limitation of our study is that we have not measured lung function and airway hyperresponsiveness. However, this study drawback does not interfere with our conclusions. We have shown that airways exposure to a Tp extract (which is easily cultured) in mice, in less artificial acute and chronic protocols, induces a prominent eosinophilic pulmonary response, which is one of the main endpoints in this type of asthma model.
In conclusion, our study demonstrated that an extract of Tp, noncommercially produced, induced both acute and chronic allergic pulmonary response in a murine model, using a less artificial protocol according to human asthma. Experimental models of asthma with Tp may expand research in mechanistic and preclinical studies in this field.
Figures and Tables
Fig. 1
Schematic representation of protocols. (A) Acute challenge to Tp extract (n=9). (B) Chronic challenge to Tp extract (n=6-8). Tp, Tyrophagus putrescentiae; DPBS, phosphate-buffered saline. The outcomes were performed 24 hours after the last challenge.
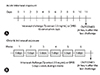
Fig. 2
Effect of acute exposure of Tp extract on total cells count (TCC) (A), differential cells count (B-E) and EPO activity (F) in BALF of mice. Results are expressed as median values and interquartile range (25-75%). Different from DPBS group: **p < 0.01, ***p < 0.001. Tp, Tyrophagus putrescentiae; DPBS, phosphate-buffered saline; EPO, eosinophil peroxidase activity; BALF, bronchoalveolar lavage fluid.
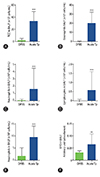
Fig. 3
Effect of chronic exposure of Tp extract on total cells count (TCC) (A), differential cells counts (B-E) and EPO activity (F) in BALF of mice. Results are expressed as median values and interquartile range (25-75%). Different from DPBS group: **p < 0.01, ***p < 0.001. Tp, Tyrophagus putrescentiae; DPBS, phosphate-buffered saline; EPO, eosinophil peroxidase activity; BALF, bronchoalveolar lavage fluid.
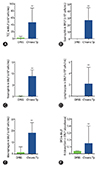
Fig. 4
Effect of acute and chronic exposure of Tp extract on cytokines levels. IL-5 levels (A, acute; C, chronic) and IL-13 levels (B, acute; D, chronic) in BALF of mice. Results are expressed as median values and interquartile range (25-75%). Different from DPBS group: *p<0.05, **p<0.01. IL, interleukin; Tp, Tyrophagus putrescentiae; DPBS, phosphate-buffered saline.
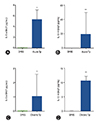
Fig. 5
Effect of acute and chronic exposure of Tp extract on histopathologic analysis. Representative photomicrographs of stained sections with H&E (A-D, ×200; arrow indicate inflammatory infiltrate cells), alcian blue (E-H, ×200 and ×1,000; arrow indicate globet cells) and picrosirius red (I-L, ×100; arrow indicate the presence of collagen). The slides from acute DPBS group are presented at the first column, acute Tp at the second, chronic DPBS group at the third and chronic Tp at the last column. Tp, Tyrophagus putrescentiae; DPBS, phosphate-buffered saline.
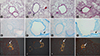
ACKNOWLEDGEMENTS
This study was supported by Conselho Nacional de Desenvolvimento Científico e Tecnológico, CNPq, Brazil.
References
2. Herbert C, Siegle JS, Shadie AM, Nikolaysen S, Garthwaite L, Hansbro NG, Foster PS, Kumar RK. Development of asthmatic inflammation in mice following early-life exposure to ambient environmental particulates and chronic allergen challenge. Dis Model Mech. 2013; 6:479–488.


3. Ishmael FT. The inflammatory response in the pathogenesis of asthma. J Am Osteopath Assoc. 2011; 111:11 Suppl 7. S11–S17.
4. Panganiban RP, Pinkerton MH, Maru SY, Jefferson SJ, Roff AN, Ishmael FT. Differential microRNA epression in asthma and the role of miR-1248 in regulation of IL-5. Am J Clin Exp Immunol. 2012; 1:154–165.
5. Barnes PJ. Immunology of asthma and chronic obstructive pulmonary disease. Nat Rev Immunol. 2008; 8:183–192.


6. Araujo MI, Junior MM, Cardoso LS, Oliveira RR, Carvalho EM. Sistema de regulacao da resposta imune alérgica. Gaz Méd Bahia. 2008; 78(Suplemento 2):18–25.
7. Koyasu S, Moro K. Type 2 innate immune responses and the natural helper cell. Immunology. 2011; 132:475–481.


8. Cates EC, Fattouh R, Wattie J, Inman MD, Goncharova S, Coyle AJ, Gutierrez-Ramos JC, Jordana M. Intranasal exposure of mice to house dust mite elicits allergic airway inflammation via a GM-CSF-mediated mechanism. J Immunol. 2004; 173:6384–6392.


9. Rydell-Törmanen K, Johnson JR, Fattouh R, Jordana M, Erjefalt JS. Induction of vascular remodeling in the lung by chronic house dust mite exposure. Am J Respir Cell Mol Biol. 2008; 39:61–67.


10. Gualdi LP, Pereira AC, Masiero L, Nunez NK, Cao R, Pitrez PM. Murine models for asthma research: an updated critical analysis. Sci Med (Porto Alegre). 2010; 20:236–242.
11. Dust mite allergens and asthma: a worldwide problem. J Allergy Clin Immunol. 1989; 83(2 Pt 1):416–427.
12. Caraballo L, Puerta L, Martinez B, Moreno L. Identification of allergens from the mite Blomia tropicalis. Clin Exp Allergy. 1994; 24:1056–1060.
13. Liao EC, Ho CM, Yin SC, Tsai JJ. Immune responses to tyrophagus putrescentiae-induced airway inflammation in mice. J Investig Allergol Clin Immunol. 2013; 23:20–29.
14. Fernandez-Caldas E, Puerta L, Caraballo L. Mites and allergy. Chem Immunol Allergy. 2014; 100:234–242.
15. Yu SJ, Liao EC, Tsai JJ. House dust mite allergy: environment evaluation and disease prevention. Asia Pac Allergy. 2014; 4:241–252.


16. Iglesias-Souto J, Sanchez-Machin I, Iraola V, Poza P, Gonzalez R, Matheu V. Oral mite anaphylaxis by Thyreophagus entomophagus in a child: a case report. Clin Mol Allergy. 2009; 7:10.


17. Liao EC, Lin YH, Chiu CL, Lin TC, Tsai JJ. Identification of allergenic component Tyr p 8 from Tyrophagus putrescentiae and cross-reactivity with Der p 8. Clin Vaccine Immunol. 2013; 20:506–512.


18. Arlian LG, Geis DP, Vyszenski-Moher DL, Bernstein IL, Gallagher JS. Antigenic and allergenic properties of the storage mite Tyrophagus putrescentiae. J Allergy Clin Immunol. 1984; 74:166–171.


19. Silveira MR, Nunes KP, Cara DC, Souza DG, Correa A Jr, Teixeira MM, Negrao-Correa D. Infection with Strongyloides venezuelensis induces transient airway eosinophilic inflammation, an increase in immunoglobulin E, and hyperresponsiveness in rats. Infect Immun. 2002; 70:6263–6272.
20. Park JW, Ko SH, Yong TS, Ree HI, Jeoung BJ, Hong CS. Cross-reactivity of Tyrophagus putrescentiae with Dermatophagoides farinae and Dermatophagoides pteronyssinus in urban areas. Ann Allergy Asthma Immunol. 1999; 83(6 Pt 1):533–539.


21. Johnson JR, Wiley RE, Fattouh R, Swirski FK, Gajewska BU, Coyle AJ, Gutierrez-Ramos JC, Ellis R, Inman MD, Jordana M. Continuous exposure to house dust mite elicits chronic airway inflammation and structural remodeling. Am J Respir Crit Care Med. 2004; 169:378–385.


22. Ulrich K, Hincks JS, Walsh R, Wetterstrand EM, Fidock MD, Sreckovic S, Lamb DJ, Douglas GJ, Yeadon M, Perros-Huguet C, Evans SM. Anti-inflammatory modulation of chronic airway inflammation in the murine house dust mite model. Pulm Pharmacol Ther. 2008; 21:637–647.


23. Hongjia L, Qingling G, Meiying L, Weixuan W, Lihong Z, Yongsheng G, Yanli L, Jinxiang W, Liang D. House dust mite regulate the lung inflammation of asthmatic mice through TLR4 pathway in airway epithelial cells. Cell Biochem Funct. 2010; 28:597–603.


24. Fattouh R, Midence NG, Arias K, Johnson JR, Walker TD, Goncharova S, Souza KP, Gregory RC Jr, Lonning S, Gauldie J, Jordana M. Transforming growth factor-beta regulates house dust mite-induced allergic airway inflammation but not airway remodeling. Am J Respir Crit Care Med. 2008; 177:593–603.
25. Al-Muhsen S, Johnson JR, Hamid Q. Remodeling in asthma. J Allergy Clin Immunol. 2011; 128:451–462.

