This article has been corrected. See "Erratum: Interferon-alpha inhibits airway eosinophila and hyperresponsiveness in an animal asthma model" in Volume 3 on page 75.
Abstract
Background
Asthma is characterized by a chronic inflammatory process involving high numbers of inflammatory cells and mediators which have multiple inflammatory effects on the airway. Interferon (IFN)-alpha, which is used widely for treating chronic hepatitis C, is reported to have an effect on patients with Churg-Strauss syndrome. Therefore, it may also be suitable for patients with severe asthma.
Objective
We studied the effect of IFN-alpha on airway eosinophilia in a guinea pig model of asthma and the expression of adhesion molecules on human eosinophils and vascular endothelial cells.
Methods
After antigen challenge, airway hyperresponsiveness and airway eosinophilia were measured in a guinea pig asthma model with or without airway IFN-alpha administration. Expression of adhesion molecules on eosinophils and cultured human umbilical vein endothelial cells (HUVECs) was also evaluated with or without IFN-alpha.
Results
IFN-alpha inhibited eosinophil recruitment into the tracheal wall and improved airway hyperresponsiveness in sensitized guinea pigs. IFN-alpha also significantly suppressed IL-1 beta-induced intercellular adhesion molecule-1 (ICAM-1) expression on HUVECs. However, IFN-alpha did not suppress platelet-activating factor-induced macrophage antigen-1 expression on human eosinophils. IFN-alpha significantly inhibited eosinophil adhesion to IL-1 beta-induced HUVECs and migration through IL-1 beta induced HUVECs.
Asthma is characterized as eosinophilic airway inflammation and hyperresponsiveness [1, 2]. T helper 2 (Th2) cytokines such as interleukin (IL)-5-activate eosinophils [3-7] and enhance eosinophil adhesion to vascular epithelial cells [8], and migration of eosinophils causes eosinophilic airway inflammation.
Interferon (IFN)-alpha has been widely used in the treatment of chronic hepatitis C [9-12]. IFN-alpha also favors both induction of Th1 cytokines and suppression of Th2 cytokines by signaling mechanisms [13-15]. IFN-alpha inhibits the production of IL-5 by CD4+ T cells [16] and inhibits eosinophils and CD4+ T cell recruitment into tissue [17]. In fact, the efficacy of therapeutic administration of IFN-alpha to patients with Churg-Strauss syndrome has been reported [18-20]. Consequently, these findings suggest that IFN-alpha may downregulate eosinophil activation and have an effect on severe asthma. To evaluate the effect of IFN-alpha for asthma, a guinea pig model of asthma was used for airway hyperresponsiveness, and human vascular endothelial cells and human eosinophils were used for the expression of adhesion molecules. IFN-alpha could be a viable treatment if its efficacy for asthma can be demonstrated, because IFN-alpha has been used widely for the treatment of chronic hepatitis C and there is ample information on IFN-alpha, including its side effects.
Human IFN-alpha (Hayashihara Lab, Osaka, Japan) composed of subtypes alpha 2, alpha 7, and alpha 8 was used for in vitro and in vivo experiments. Recombinant human (rh) IL-1 beta (Genzyme Co., MA, USA) and platelet activating factor (PAF; Sigma-Aldrich Co., MO, USA) were also used.
Anti-macrophage antigen-1 (Mac-1; CD11b, and mouse IgG2a) monoclonal antibody (mAb) and mouse isotype control mAbs (IgG1 and IgG2a) were purchased from Becton-Dickinson (CA, USA). Anti-human intercellular adhesion molecule-1 (ICAM-1; mouse IgG1) mAb and anti-human vascular adhesion molecule-1 (VCAM-1; mouse IgG1) were purchased from Genzyme Co. Fluorescein isothiocyanate (FITC)-conjugated goat anti-mouse F(ab)'2 fragments were also purchased from Becton-Dickinson.
Guinea pigs were sensitized by exposure to aerosolized 10 mg/mL of ovalbumin (OVA; Sigma-Aldrich Co.) saline solution once a day for 10 consecutive days [21]. The aerosol was generated by an ultrasonic nebulizer (NE-IOB; Omuron Co., Ltd., Kyoto, Japan) applied to the center of a round tub. Guinea pigs were placed radially with their heads directed towards the aerosol-supplying center of the tub and were each exposed to an equivalent density of OVA aerosols for 10 min on each occasion. Seven days after the end of the 10-day exposure of OVA aerosols, and 3 h before the OVA challenge, the guinea pigs were intraperitoneally (IP) injected with IFN-alpha, which were suspended 2.0 mL/animal of (a) high-(2 × 107 U/kg) or (b) low dose- (2 × 106 U/kg) or (c) saline solution alone. Then, they were exposed to 10 mg/mL aerosolized OVA saline solution for 10 min using a DeVilbiss 646 nebulizer (DeVilbiss Co., PA, USA) driven by compressed air at 5 L/min. Airway hyperresponsiveness was measured immediately before and 24 h after OVA challenge (Fig. 1).
Respiratory resistance (Rrs) was measured by a forced-oscillation technique (Mead's oscillation method) [22]. These measurements were carried out in inhalation-sensitized guinea pigs [23]. Conscious animals were placed in a body plethysmograph, leaving their heads outside and their necks restrained by a doughnut-shaped rubber balloon which scaled the body chamber, and an 18 Hz sine-wave oscillation was applied to their body surfaces. Oscillating pressure was generated (RC oscillator ORC 11; Kikusui Electronics Co., Kanagawa, Japan) with a 24 cm woofer speaker (Pioneer Co., Kanagawa, Japan) derived by a sine-wave generator and power amplifier (M-50; NEC Co., Tokyo, Japan). A rubber mask with a 200 mesh screen was snugly applied to the face in order to measure the flow rate. The flow rate through the mask was measured by detecting the pressure difference across the 200 mesh screen using a differential pressure transducer (DP-45-18; Validyne Engineering Co., CA, USA). Box pressure was measured by a pressure transducer (DP-45-26; Validyne Engineering Co.). The signals of the flow rate and the box pressure were amplified (Carrier Amplifier; Shizume Medical Co., Tokyo, Japan) and were then recorded on a polygraph recorder (WTR 751; Graphtec Co., Kanagawa, Japan), as well as on the X and Y axis of an oscilloscope (COS5021TM; Kikusui Electronics Co.). Rrs was calculated from the ratio of amplitude of the oscillating box pressure and flow rate fluctuations.
Airway responsiveness to inhaled histamine (Sigma-Aldrich Co.) was expressed using PC200-Rrs-histamine. Serially doubling dilutions of histamine solution of 39-20,000 µg/mL were prepared. Animals were exposed to the aerosol of each histamine solution for 10 min by increasing the concentration of each solution as described above. Rrs was measured immediately after inhalation, which was stopped when Rrs exceeded 200% of the baseline level. PC200-Rrs-histamine expressed the concentration of histamine solution to produce 100% increase in Rrs compared with baseline.
Animals were killed by IP injection of pentobarbital (100 mg/kg body weight). Trachea were removed and fixed in 10% formalin and embedded in paraffin, and 3-µm-thick sections were stained with May-Giemsa and Hansel solution. Epithelial and under basement membrane eosinophils were counted separately. The above protocol, including sensitization and measurement of airway hyperresponsiveness, was approved by the Committee of Laboratory Animal Research Center at Dokkyo Medical University (No. GP-9832).
Human eosinophils were isolated by the technique described by Tenor et al. [24]. Purity of attained eosinophils was > 99.5%, as assessed by Kimura staining after this procedure.
Human umbilical vein endothelial cells (HUVECs; Kurabou Biomedical, Osaka, Japan) were grown to confluence in a 24-well plate (Becton-Dickinson, Lincoln Park, NJ), and purified human eosinophils (2 × 105 cells/well) were added. Monolayers of HUVECs were pretreated with IFN-alpha or human albumin at 37℃ in a humidified CO2 (5%) incubator for 30 min and stimulated with 5 nM/mL of rh IL-1 beta for 4 h. After incubation, non-adherent eosinophils were collected, and the percentage of adhesion was calculated as follows: [(total cell number - non-adherent cell number)/total cell number] × 100.
A Transendothelial migration assay was prepared using a modified version of Ebisawa's method [25]. HUVECs at 2 × 104 cells from the third passage were cultured on 2% gelatin-coated Transwell culture inserts (6.5-mm diameter polycarbonate membrane with 5-µm pores, Costar, MA, USA). Fresh endothelial cell growth medium (0.2 mL EBM; Sankojunyaku, Tokyo, Japan) was added to the upper and lower transwell chambers. A monolayer of confluent HUVECs formed within 7 days. To confirm HUVEC confluency, monolayers were stained routinely with Diff-Quik (American Scientific Products, McGraw Park, IL) before experiments. After preincubation for 30 min with IFN-alpha, the HUVECs monolayer was incubated at 37℃ for 4 h with 5 ng/mL of IL-1 beta or EBM only without glucocorticoid (control). After incubation, the culture medium with IFN-alpha and IL-1 beta was removed and rinsed twice. Then, 0.2 mg eosinophil suspension (2 × 106 cells/mL) was added to each upper chamber and incubated for 2 h at 37℃. After incubation, non-adherent cells in the upper chamber were removed. Eosinophils that had migrated and fallen into the lower chamber were removed to centrifugal tubes and centrifuged at 300 g for 5 min at 4℃. Migrated eosinophils were stained with trypan blue and counted.
Eosinophils resuspended in RPMI-1640 supplemented with 1% fetal bovine serum at a cell concentration of 1 × 106/mL were incubated for 30 min with IFN-alpha and added platelet-activating factor (PAF; 1 × 10-5 M/mL) for 15 min. HUVECs were detached from the culture flasks using 0.025% trypsin and 0.01% EDTA solution and were counted and resuspended at 1 × 106 cells/mL in EBM without glucocorticoid. After preincubation for 30 min with IFN-alpha, HUVECs were incubated with 5 ng/mL of rh IL-1 beta for 4 h. Cells were then washed twice with phosphate buffered saline and kept on ice until analyzed.
Eosinophils were evaluated by immunofluorescence and flow cytometry for expression of CD11b, LFA-1a, and VLA4 before and after PAF stimulation. In addition, HUVECs were evaluated for expression of ICAM-1. Cells were labeled with saturating amounts of each mAb (~10-50 µL), and volume was made up to 100 µL where necessary with ice-cold flow cytometry buffer. After incubation for 30 min at 4℃, the cells were washed once with 2 mL of cold flow cytometry buffer, resuspended in PBS, and kept on ice until analyzed. When unlabeled mAbs were used, cells were mixed with an excess of FITC-conjugated F(ab')2 fragments of goat antibody to mouse immunoglobulins. After incubation for 15 min at 4℃, the cells were washed, resuspended in PBS, and kept on ice until analyzed.
Flow cytometry was performed on a FACScan instrument (Becton-Dickinson) equipped with an electronic (Coulter) volume sensor, which was standardized with fluorescent microbeads. The fluorescence intensity of 5,000 cells was determined from each sample by logarithmic amplification, which was converted to the linear equivalent by means of a Hewlett-Packard Consort 30 computer. Cell populations and debris were defined by gating the volume and 90° light scatter parameters. Dead cells were excluded from analysis by gating out propidium iodide-positive cells. The mean fluorescence of the population was calculated using the computer, and the value for the control antibody was subtracted from the value for the specific mAb to give a specific mean fluorescence value.
All statistical analyses were performed using Microsoft Excel®, StatView®, or JMP® for the t-test and Wilcoxon signed rank test. Differences with a p value < 0.05 were considered significant. Results are expressed as mean ± SEM values. PC200-Rrs-histamine values were converted into a logarithm for statistical calculation.
High- (2 × 107 U/kg) and low- (2 × 106 U/kg) dose IFN-alpha significantly inhibited eosinophil accumulation (1132 ± 70/mm2 and 1305 ± 68/mm2, respectively) compared with no treatment (1501 ± 64/mm2) (p < 0.01 and p < 0.05, respectively) (Fig. 2). Similarly, high- and low-dose IFN-alpha significantly decreased airway hyperresponsiveness (Rrs: 5000 ± 945 µg/mL histamine and Rrs: 1228 ± 365 µg/mL histamine, respectively) compared with no treatment (Rrs: 279 ± 68 µg/mL histamine) (p < 0.01, p < 0.05, respectively). High-dose IFN-alpha significantly decreased airway hyperresponsiveness compared with models before OVA challenge (1651 ± 325 µg/mL, respectively) (p < 0.05) (Fig. 3). Histological examination and measurement of airway hyperresponsiveness were performed separately, because histamine might induce airway inflammation.
In vitro, IFN-alpha was suspended in culture medium at 1 × 105 U/mL (high-dose), 1 × 104 U/mL (middle-dose), or 1 × 103 U/mL (low-dose), and no treatment was used as a control. Mean fluorescence of ICAM-1 expression on HUVACs activated by 5 ng/mL of IL-1 beta was 221.7 ± 15.0, 237.8 ± 14.5, and 271.7 ± 28.7 for high-dose, middle-dose, low-dose pretreatment, respectively, compared with 279.3 ± 15.1 for no treatment (Fig. 4A). ICAM-1 expression was inhibited dose-dependently by IFN-alpha (p < 0.01, p < 0.05). Mean fluorescence of VCAM-1 expression on HUVACs activated by 5 ng/mL of IL-1 beta was 189.0 ± 29.7, 266.9 ± 23.0, and 256.5 ± 22.8 for high-dose, middle-dose, low-dose pretreatment, respectively, compared with 283.4 ± 30.9 for no treatment (Fig. 4B). VCAM-1 expression was inhibited by high-dose IFN-alpha only (p < 0.01). However, IFN-alpha did not suppress PAF-induced Mac-1 expression on human eosinophils (data not shown).
For the adhesion investigation, the percent of adherent eosinophils was 52.6 ± 4.1%, 63.4 ± 4.1%, and 71.6 ± 4.1% for high-dose, middle-dose, low-dose pretreatment, respectively, compared with 83.8 ± 4.9% for no treatment (Fig. 5). IFN-alpha significantly and dose dependently inhibited eosinophil adhesion to HUVECs activated by 5 ng/mL of IL-1 beta (p < 0.01). Furthermore, the percent of migrated eosinophils was 6.7 ± 1.0%, 8.0 ± 1.3%, and 8.5 ± 1.4% for high-dose, middle-dose, and low-dose pretreatment, respectively, compared with 10.3 ± 1.3% for no treatment (Fig. 6). High dose IFN-alpha significantly inhibited eosinophil migration through IL-1 beta-activated HUVECs compared with controls (p < 0.02).
We found that IFN-alpha inhibited both airway eosinophilia and hyperresponsiveness in a guinea pig model of asthma. IFN-alpha inhibited the expression of ICAM-1 on vascular endothelial cells and the adherence of eosinophils to vascular endothelial cells. IFN-alpha also inhibited migration of eosinophils via vascular endothelial cells.
Several studies that evaluated the relationship between IFN-alpha and pulmonary function have been published and are in agreement with the present findings. For example, for severe corticosteroid-dependent asthma without chronic hepatitis C, IFN-alpha improved pulmonary function, including forced expiratory volume in 1 second (FEV1) and peak expiratory flow (PEF) [26-28]. On the other hand, patients with chronic hepatitis C did not show the same results. It was reported that two patients with both asthma and chronic hepatitis C experienced exacerbation of asthma promptly after the use of IFN-alpha [29]. In non-asthmatic patients with chronic hepatitis C, 3 of 17 experienced enhanced airway hyperresponsiveness after IFN-alpha treatment, although other pulmonary functions, including FEV1, showed no change between before and after treatment in any patients [30]. Another publication reported that the IFN response to chronic hepatitis C was associated with impaired responses to inhaled corticosteroid therapy in patients with both asthma and chronic hepatitis C, but no responders showed improved FEV1 by inhaled corticosteroid therapy [31]. Although IFN-alpha improves asthma in patients without chronic hepatitis C, we have to understand that IFN-alpha may cause exacerbation of asthma in patients with chronic hepatitis C.
In our study, low-dose IFN-alpha inhibited the expression of ICAM-1 but not VCAM-1. However, high-dose IFN-alpha inhibited expression of both ICAM-1and VCAM-1. Several studies have reported that treatment of IFN-alpha decreased the serum levels of soluble ICAM-1, but not soluble VCAM-1, in patients with chronic hepatitis C [32, 33]. Such results suggest that the main target of adhesion molecules will be ICAM-1, which can be inhibited by low-dose IFN-alpha. ICAM-1 expression on human endothelial cells is enhanced by IL-1 beta and tumor necrosis factor [34]. IFN-alpha inhibits ICAM-1 expression, because IFN-alpha inhibits IL-1 induced by endothelial cells [35]. Accordingly, eosinophil migration into tissue is inhibited.
In our study, the same results were shown both in vivo and in vitro, indicating that ICAM-1 may play an important role in airway eosinophilic inflammation. On the other hand, ICAM-1 expression is not always inhibited by IFN-alpha therapy. It was reported that 10 of 23 patients with metastatic malignant melanoma showed high expression of ICAM-1 after IFN-alpha therapy [36]. In addition, patients with metastatic malignant melanoma who developed depression had higher soluble ICAM-1 levels than non-depressed patients after treatment with IFN-alpha, compared with baseline levels [37]. IFN-alpha treatment may also contribute to neurotoxic effects. Furthermore, ICAM-1 expression in malignant diseases is increased and other signaling pathways in malignant diseases will be constructed to be increased ICAM-1 expression by IFN-alpha treatment.
IFN-alpha dose-dependently reduced the number of infiltrating eosinophils in airway tissue in our study. Two processes are candidate mechanisms through which IFN-alpha inhibits airway eosinophilic inflammation. The first process is inhibition of eosinophils by IFN-alpha via downregulation of T helper type 2 (Th2) cells. Here, IFN-alpha and IL-12 jointly promote differentiation of T helper type 0 (Th0) toward the T helper type 1 (Th1) phenotype, and IFN-alpha selectively stimulates Th1 cells to release IFN-gamma [38-40]. The activated Th1 cells downregulate Th2 cells by Th1-Th2 balance and inhibit the release of cytokines, which in turn activates eosinophils and mast cells, such as IL-4 and IL-5, from Th2 cells. Airway eosinophilic inflammation is subsequently inhibited by downregulation of Th2 cells. The second process is inhibition of the migration of eosinophils into airway tissue by IFN-alpha via ICAM-1 on HUVECs. The expression of HUVEC surface ICAM-1 is induced by tumor necrosis factor-alpha (TNF-alpha) and IL-1 [41], and the expression of ICAM-1 stimulated by IL-1 was inhibited by IFN-alpha in our study. The experiment for signaling pathways has not been performed, and we cannot explain the reason. Because ICAM-1 expression in malignant diseases is increased by IFN-alpha treatment, signaling pathways may not be simple. But, in an animal model of asthma without malignant diseases, IFN-alpha inhibits the expression of ICAM-1 on HUVECs. Thus, it is thought that IFN-alpha inhibits airway eosinophilic inflammation via both of these two processes, leading to inhibition of airway hyperresponsiveness.
In conclusion, IFN-alpha inhibits airway eosinophilic inflammation and airway hyperresponsiveness possibly by inhibition of ICAM-1 expression on endothelial cells. IFN-alpha is used widely for patients with chronic hepatitis C and can be used safely. Therefore, IFN-alpha treatment should be considered for uncontrolled patients with glucocorticoid-dependent severe asthma. However, when patients have malignant diseases or chronic virus infections, such as chronic hepatitis C, caution must be taken when using IFN-alpha because pulmonary function or airway hyperresponsiveness may be decreased when coexisting with those diseases.
Figures and Tables
Fig. 2
Effect of IFN-alpha on eosinophil infiltration into tracheal wall of guinea pig. OVA-sensitized guinea pigs were intraperitoneally injected with IFN-alpha or saline 3 h before OVA challenge. Trachea were removed 24 h after antigen challenge and fixed in 10% formalin and embedded in paraffin, and 3-µm-thick sections were stained with May-Giemsa and Hansel solution. Eosinophils which had infiltrated into the trachea were counted. Eosinophil infiltration was inhibited dose dependently by IFN-alpha. Mean ± SEM values of 15 animals are presented.
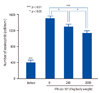
Fig. 3
Effect of IFN-alpha on airway hyperresponsiveness in guinea pigs. OVA-sensitized guinea pigs were intraperitoneally injected with IFN-alpha or saline 3 h before antigen challenge. The airway hyperresponsiveness was examined 24 h after antigen challenge. Data are presented as a logarithm of PC200-Rrs-histamine. Airway hyperresponsiveness was inhibited dose dependently by IFN-alpha. Mean ± SEM values of 7 animals are presented. Rrs, respiratory resistance.
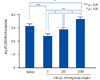
Fig. 4
Effect of IFN-alpha on IL-1 beta-induced ICMA-1 (A) and VCAM-1 (B) expression on HUVECs. HUVECs were pretreated with IFN-alpha for 30 min and stimulated with IL-1 beta for 4 h. Cells were stained with anti-ICAM-1 or anti-VCAM-1, and mean specific fluorescence was determined by flow cytometry. ICAM-1 expression was inhibi ted dose dependently by IFN-alpha, while VCAM-1 expression was inhibited by high-dose of IFN-alpha only. Mean ± SEM values of 8 samples are presented.
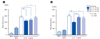
Fig. 5
Effect of IFN-alpha on human eosinophil adhesion to HUVECs. HUVECs were grown to confluence in 24-well plates. HUVEC monolayers were pretreated with IFN-alpha for 30 min before IL-1 beta for 4 h. Then, purified eosinophil suspensions were added to each well. After 1 h incubation at room temperature, supernatants were collected and counted as non-adherent cells. Data show adherent cells (%), which were calculated based on the number of eosinophils in the supernatants. Adherent eosinophils were inhibited dose dependently by IFN-alpha. Mean ± SE values of 6 samples are presented.
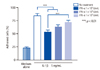
Fig. 6
Effect of IFN-alpha on human eosinophil transmigration through HUVECs. HUVECs were pretreated with IFN-alpha and activated with IL-1 beta. Purified human eosinophils were added to upper wells. After 2 h incubation at 37℃ in a humidified CO2 (5%) incubator, lower well supernatants were collected and counted as transmigrated wells. Data are shown as migrated cells (%), which were calculated based on the number of eosinophils in the lower chamber. High-dose IFN-alpha significantly inhibited migration of eosinophils. Mean ± SE values of 10 samples are presented.
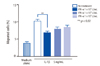
ACKNOWLEDGEMENTS
Authors thank Dr. Takashi Ueda, Dr. Yoshinori Terashi, and Dr. Hironori Sagara for technical advice and Dr. Cheng Gang for technical assistant. In addition, we thank Miss Ayano Takeda for her assistance preparing the manuscript.
References
1. Barnes PJ. New concepts in the pathogenesis of bronchial hyperresponsiveness and asthma. J Allergy Clin Immunol. 1989. 83:1013–1026.


2. Bousquet J, Chanez P, Lacoste JY, Barnéon G, Ghavanian N, Enander I, Venge P, Ahlstedt S, Simony-Lafontaine J, Godard P, Michel FB. Eosinophilic inflammation in asthma. N Engl J Med. 1990. 323:1033–1039.


3. Lopez AF, Sanderson CJ, Gamble JR, Campbell HD, Young IG, Vadas MA. Recombinant human interleukin 5 is a selective activator of human eosinophil function. J Exp Med. 1988. 167:219–224.


4. Wang JM, Rambaldi A, Biondi A, Chen ZG, Sanderson CJ, Mantovani A. Recombinant human interleukin 5 is a selective eosinophil chemoattractant. Eur J Immunol. 1989. 19:701–705.


5. Fukuda T, Nakajima H, Fukushima Y, Akutsu I, Numao T, Majima K, Motojima S, Sato Y, Takatsu K, Makino S. Detection of interleukin-5 messenger RNA and interleukin-5 protein in bronchial biopsies from asthma by nonradioactive in situ hybridization and immunohistochemistry. J Allergy Clin Immunol. 1994. 94:584–593.


6. Adachi T, Motojima S, Hirata A, Fukuda T, Makino S. Eosinophil viability-enhancing activity in sputum from patients with bronchial asthma, Contributions of interleukin-5 and granulocyte/macrophage colony-stimulating factor. Am J Respir Crit Care Med. 1995. 151:618–623.


7. Sur S, Kita H, Gleich GJ, Chenier TC, Hunt LW. Eosinophil recruitment is associated with IL-5, but not with RANTES, twenty-four hours after allergen challenge. J Allergy Clin Immunol. 1996. 97:1272–1278.


8. Sanmugalingham D, De Vries E, Gauntlett R, Symon FA, Bradding P, Wardlaw AJ. Interleukin-5 enhances eosinophil adhesion to bronchial epithelial cells. Clin Exp Allergy. 2000. 30:255–263.


9. Manns MP, McHutchison JG, Gordon SC, Rustgi VK, Shiffman M, Reindollar R, Goodman ZD, Koury K, Ling M, Albrecht JK. Peginterferon alfa-2b plus ribavirin compared with interferon alfa-2b plus ribavirin for initial treatment of chronic hepatitis C: a randomised trial. Lancet. 2001. 358:958–965.


10. Fried MW, Shiffman ML, Reddy KR, Smith C, Marinos G, Gonçales FL Jr, Häussinger D, Diago M, Carosi G, Dhumeaux D, Craxi A, Lin A. Peginterferon alfa-2a plus ribavirin for chronic hepatitis C virus infection. N Engl J Med. 2002. 347:975–982.


11. Poordad F, McCone J Jr, Bacon BR, Bruno S, Manns MP, Sulkowski MS, Jacobson IM, Reddy KR, Goodman ZD, Boparai N, DiNubile MJ, Sniukiene V, Brass CA, Albrecht JK, Bronowicki JP. SPRINT-2 Investigators. Boceprevir for untreated chronic HCV genotype 1 infection. N Engl J Med. 2011. 364:1195–1206.


12. Jacobson IM, McHutchison JG, Dusheiko G, Di Bisceglie AM, Reddy KR, Bzowej NH, Marcellin P, Muir AJ, Ferenci P, Flisiak R, George J, Rizzetto M, Shouval D, Sola R, Terg RA, Yoshida EM, Adda N, Bengtsson L, Sankoh AJ, Kieffer TL, George S, Kauffman RS, Zeuzem S. ADVANCE Study Team. Telaprevir for previously untreated chronic hepatitis C virus infection. N Engl J Med. 2011. 364:2405–2416.


13. Shibuya H, Hirohata S. Differential effects of IFN-alpha on the expression of various TH2 cytokines in human CD4+ T cells. J Allergy Clin Immunol. 2005. 116:205–212.
14. Tilg H, Kaser A. Type I interferons and their therapeutic role in Th2-regulated inflammatory disorders. Expert Opin Biol Ther. 2004. 4:469–481.


16. Schandené L, Del Prete GF, Cogan E, Stordeur P, Crusiaux A, Kennes B, Romagnani S, Goldman M. Recombinant interferon-alpha selectively inhibits the production of interleukin-5 by human CD4+ T cells. J Clin Invest. 1996. 97:309–315.


17. Nakajima H, Nakao A, Watanabe Y, Yoshida S, Iwamoto I. IFN-alpha inhibits antigen-induced eosinophil and CD4+ T cell recruitment into tissue. J Immunol. 1994. 153:1264–1270.
18. Tatsis E, Schnabel A, Gross WL. Interferon-alpha treatment of four patients with the Churg-Strauss syndrome. Ann Intern Med. 1998. 129:370–374.
19. Termeer CC, Simon JC, Schöpf E. Low-dose interferon alfa-2b for the treatment of Churg-Strauss syndrome with prominent skin involvement. Arch Dermatol. 2001. 137:136–138.
20. Hellmich B, Gross WL. Recent progress in the pharmacotherapy of Churg-Strauss syndrome. Expert Opin Pharmacother. 2004. 5:25–35.


21. Cheng G, Ueda T, Sugiyama K, Toda M, Fukuda T. Compositional and functional changes of pulmonary surfactant in a guinea-pig model of chronic asthma. Respir Med. 2001. 95:180–186.


23. Toda M, Motojima S, Fukuda T, Makino S. Effect of an herbal preparation, Saiboku-to (TJ-96), on antigen-induced airway hyperresponsiveness and eosinophil infiltration in actively sensitized guinea pigs. off. Ann N Y Acad Sci. 1993. 685:561–571.
24. Tenor H, Hatzelmann A, Church MK, Schudt C, Shute JK. Effects of theophylline and rolipram on leukotriene C4 (LTC4) synthesis and chemotaxis of human eosinophils from normal and atopic subjects. Br J Pharmacol. 1996. 118:1727–1735.


25. Ebisawa M, Bochner BS, Georas SN, Schleimer RP. Eosinophil transendothelial migration induced by cytokines. I. Role of endothelial and eosinophil adhesion molecules in IL-1 beta-induced transendothelial migration. J Immunol. 1992. 149:4021–4028.
26. Gratzl S, Palca A, Schmitz M, Simon HU. Treatment with IFN-alpha in corticosteroid-unresponsive asthma. J Allergy Clin Immunol. 2000. 105:1035–1036.
27. Simon HU, Seelbach H, Ehmann R, Schmitz M. Clinical and immunological effects of low-dose IFN-alpha treatment in patients with corticosteroid-resistant asthma. Allergy. 2003. 58:1250–1255.
28. Kroegel C, Bergmann N, Foerster M, Workalemahu G, Machnik A, Mock B, Reissig A. Interferon-alphacon-1 treatment of three patients with severe glucocorticoid-dependent asthma. Effect on disease control and systemic glucocorticosteroid dose. Respiration. 2006. 73:566–570.
29. Bini EJ, Weinshel EH. Severe exacerbation of asthma: a new side effect of interferon-alpha in patients with asthma and chronic hepatitis C. Mayo Clin Proc. 1999. 74:367–370.
30. Shirai T, Honjo Y, Takashima M, Takayanagi S, Chida K, Nakamura H. Effect of interferon-α on pulmonary function and airway responsiveness in patients with chronic hepatitis C. Allergol Int. 2001. 50:331–335.


31. Kanazawa H, Mamoto T, Hirata K, Yoshikawa J. Interferon therapy induces the improvement of lung function by inhaled corticosteroid therapy in asthmatic patients with chronic hepatitis C virus infection: a preliminary study. Chest. 2003. 123:600–603.
32. Capra F, De Maria E, Lunardi C, Marchiori L, Mezzelani P, Beri R, Gabrielli GB. Serum level of soluble intercellular adhesion molecule 1 in patients with chronic liver disease related to hepatitis C virus: A prognostic marker for responses to interferon treatment. J Infect Dis. 2000. 181:425–431.


33. Dejica D, Grigorescu M, Dejica V, Radu C, Neculoiu D. Serum levels of soluble intercellular-1 and vascular cell-1 adhesion molecules in chronic hepatitis C and the influence of interferon-alpha + ribavirin therapy. Rom J Gastroenterol. 2002. 11:277–283.
34. Pober JS, Gimbrone MA Jr, Lapierre LA, Mendrick DL, Fiers W, Rothlein R, Springer TA. Overlapping patterns of activation of human endothelial cells by interleukin 1, tumor necrosis factor, and immune interferon. J Immunol. 1986. 137:1893–1896.
35. Schindler R, Ghezzi P, Dinarello CA. Interferons as inhibitors of interleukin 1 induced interleukin 1 synthesis. Lymphokine Res. 1989. 8:275–280.
36. Håkansson A, Gustafsson B, Krysander L, Hjelmqvist B, Rettrup B, Håkansson L. Expression of ICAM-1 during IFN-alpha-based treatment of metastatic malignant melanoma: relation to tumor-infiltrating mononuclear cells and regressive tumor changes. J Interferon Cytokine Res. 1999. 19:171–177.
37. Schaefer M, Horn M, Schmidt F, Schmid-Wendtner MH, Volkenandt M, Ackenheil M, Mueller N, Schwarz MJ. Correlation between sICAM-1 and depressive symptoms during adjuvant treatment of melanoma with interferon-alpha. Brain Behav Immun. 2004. 18:555–562.
38. Mosmann TR, Sad S. The expanding universe of T-cell subsets: Th1, Th2 and more. Immunol Today. 1996. 17:138–146.


39. Fearon DT, Locksley RM. The instructive role of innate immunity in the acquired immune response. Science. 1996. 272:50–53.


40. Trinchieri G. Interleukin-12 and the regulation of innate resistance and adaptive immunity. Nat Rev Immunol. 2003. 3:133–146.


41. Bochner BS, Luscinskas FW, Gimbrone MA Jr, Newman W, Sterbinsky SA, Derse-Anthony CP, Klunk D, Schleimer RP. Adhesion of human basophils, eosinophils, and neutrophils to interleukin 1-activated human vascular endothelial cells: contributions of endothelial cell adhesion molecules. J Exp Med. 1991. 173:1553–1557.

