Abstract
Objectives
The purpose of this study was to observe the change in the viscoelastic properties of thermoplasticized injectable root canal filling materials as a function of temperature and to compare the handling characteristics of these materials.
Materials and Methods
Three commercial gutta perchas and Resilon (Pentron Clinical Technologies) in a pellet form were heated in the Obtura-II system (Obtura Spartan) at 140℃ and 200℃, and the extrusion temperature of the thermoplasticized materials was measured. The viscoelastic properties of the materials as a function of temperature were evaluated using a rheometer. The elastic modulus G', viscous modulus G", loss tangent tan δ, and complex viscosity η* were determined. The phase transition temperature was determined by both the rheometer and a differential scanning calorimeter (DSC). The consistency of the materials was compared under compacting pressure at 60℃ and 40℃ by a squeeze test.
Results
The three gutta perchas had dissimilar profiles in viscoelastic properties with varying temperature. The phase transition of softened materials into solidification occurred at 40℃ to 50℃, and the onset temperatures obtained by a rheometer and a DSC were similar to each other. The onset temperature of phase transition and the consistency upon compaction pressure were different among the materials (p < 0.05). Resilon had a rheologically similar pattern to the gutta perchas, and was featured between high and low-flow gutta perchas.
The effectiveness of a material to adequately seal the root canal space is related to its physical properties and handling characteristics.1 Since the introduction of the warm vertical compaction technique, the thermoplastic compaction of canal spaces has been broadly advocated. Warm gutta percha can be easily injected in the prepared canal space and adapted by pressure to the canal walls with complicated anatomy.2 The Obtura-II system (Optura Spartan, Earth City, MO, USA), a high-temperature thermoplastic injection-molded gutta-percha delivery system, is a hand-held device in which pellet-typed gutta-percha is loaded, heated, and extruded into the root canal through a silver needle.
Resilon (Pentron Clinical Technologies, Wallingford, CT, USA), a polyester polymer-based material, can also be warm-plasticized and used for the backfill of root canals via the Obtura-II system.3 Manufacturers claim that Resilon has the same handling characteristics as gutta percha. Both materials have also been shown to have the same melting point (60℃).1,4,5 However, in the comparison of specific heat capacities of the two materials, Resilon revealed significantly more heat absorption for melting compared to gutta percha.1 Resilon allowed deeper penetration with a spreader than gutta percha when using the same controlled pressure during compaction.6
Polymeric materials such as gutta percha and Resilon have a combination of elastic and plastic properties that are largely dependent on temperature. During a thermoplasticized root canal filling, the heated material becomes soft and flows into the canal space. Following this, the material is pressed and packed with adequate force to integrate the material into the irregular surface of the canal walls. As the softened materials cool, they begin to resist the compaction force and are not as easily handled. If the viscoelastic properties of thermoplasticized materials with regards to temperature are well understood, materials can be adequately manipulated for the tight and homogeneous obturation of the canal space. There have been very few studies on the rheological behavior of root canal filling materials starting from softened state at a hot injection temperature achieved by a heating device and ending in a solidified state at a cooled temperature in the root canal.
The purpose of this study was to observe the change in viscoelastic properties of three commercial gutta perchas and Resilon materials used for thermoplasticized injectable root canal fillings, and to compare the handling consistency of the materials as a function of temperature.
Three commercial gutta perchas [Obtura (Obtura Spartan, Earth City, MO, USA), DiaDent (DiaDent group international, Cheongju, Korea), and Meta (Meta Dental Co., Elmhurst, NY, USA)] and Resilon were investigated in this study. Gutta percha and Resilon pellets were inserted in an Obtura-II system, a heating and delivery device, and the temperature was set at 140℃ and 200℃. To simulate the thermoplasticized root canal filling procedure, the materials were extruded from the Obtura-II system through a 23-G silver needle attached after waiting 15 seconds after the desired temperature was reached. The first 5 mm of extrusion was discarded and the following 3 mm was squeezed on the tip of the thermocouple temperature sensor of a digital thermometer (Fluke 179, Fluke Corp., Everett, WA, USA) and the peak temperature was recorded. After each measurement, the Obtura-II system was turned off and cooled down for 5 minutes. Every measurement was made using a new pellet in the same package. Three measurements were made for each material at both temperatures.
In order to measure the viscoelasticity change of the heated canal filling materials as a function of temperature, a dynamic oscillatory shear test with temperature sweep was performed. For each type of material, 0.2 g was placed on the Peltier plate of a rotational rheometer (AR 2000, TA Instrument, New Castle, DE, USA) set at 120℃. The upper plate, which was 8 mm in diameter, was positioned at a distance of 1.0 mm above the lower Peltier plate, and the excess material was removed. The dynamic oscillatory shear test was undertaken at a frequency of 2 Hz and strain amplitude of 1% with temperature decreasing from 120℃ to 20℃ in increments of 1℃.
In the dynamic oscillatory shear test, when a material is subjected to a sinusoidal oscillatory shear strain with frequency ω, the shear strain and shear stress are given by7:
The δ is the phase angle between the stress and strain. From these expressions, two moduli can be defined:
Where G', the shear storage (elastic) modulus, with no phase lag between the stress and strain, is a measure of the stored energy. G", the shear loss (viscous) modulus, with a phase lag of π/2, is a measure of the energy lost as heat. The G' represents the elastic part of a material, while the G" represents the viscous part. The G"/G' (loss tangent, tan δ) represents the ratio of the viscous part to the elastic part (lost energy/stored energy) of the material.
Alternatively, when complex variables are used,
The complex shear modulus G* represents the combined aspects of the elastic part, G', and the viscous part, G". Its magnitude is given by:
The complex viscosity is defined as η* = G*/ω, where ω is the oscillation frequency in radians. For each material, G', G", tan δ, and η* as a function of temperature were obtained from the output responses of the rheometer. From the curve of η* with decreasing temperature, the onset point of the abrupt increase in viscosity (phase transition temperatures) of the materials were determined.
Each 0.05 g of the three gutta percha and Resilon materials was placed in an aluminum sample pan and heated up to 80℃ using a differential scanning calorimeter (DSC 204, Mettler-Toledo Inc. Giessen, Germany). The specimens were then cooled down to 30℃ at a rate of 0.1℃/min. From the thermogram of the DSC, which showed the exothermic reaction of materials with decreasing temperature, the onset and peak temperatures of the thermal transition of the materials were determined.
0.2 g of each of the gutta percha and Resilon materials was placed on the Peltier plate of the rheometer set at 60℃. The upper plate was brought to within 1 mm of the lower plate and the excess material was removed. After waiting for the relief of the residual normal stress, the upper plate was moved downward at a speed of 0.1 mm/sec to squeeze the material for 5 seconds. The resultant stress was measured 3 seconds after squeezing. The same measurement was repeated at 40℃.
The above measurements were repeated three times for every experimental setting. The data were analyzed with ANOVA and Tukey's multiple comparison test (α = 0.05) using SPSS 12.0 (SPSS, Inc., Chicago, IL, USA).
The temperatures of the materials extruded from the 23-G needle attached to the Obtura-II set at 140℃ and 200℃ are listed in Table 1. The mean temperature of each material was between 55.0℃ and 65.1℃, when it was extruded from the Obtura-II system set at 140℃. The mean temperature ranged from 79.2℃ to 84.4℃ when the Obtura-II system was set at 200℃. Resilon had a significantly lower temperature than the other three gutta perchas at 140℃ (p < 0.05), but a significantly higher temperature than the gutta percha materials at 200℃ (p < 0.05).
The viscoelastic changes of the four materials with varying temperature are shown in Figure 1. The shear moduli G' and G" increased with decreasing temperature, and each material showed differences in magnitude (Figures 1a and 1b). At temperatures of 50 - 120℃, the G' and G" ranged 104-105 Pa order. As the cooling process continued, both G' and G" started to increase steeply at 40 - 50℃ and finally reached 107-108 Pa order at 25 - 35℃.
The tan δ decreased slowly with the cooling process, began to abruptly decrease at 35 - 45℃, and exhibited a dissimilar behavior among materials based on decreasing temperature (Figure 1c). The increase in elasticity was more magnified than that in viscosity, considering that the value of tan δ changed from 0.8 (Obtura) - 2.6 (Resilon) to 0.08 (Resilon) - 0.32 (Meta) with decreasing temperature. The complex viscosity η* sharply increased in the order of Obtura, Resilon, Diadent, and Meta at the transition temperature of 40 - 50℃ (Figure 1d).
The transition temperatures indicated the point at which the exothermic change of the materials was initiated during cooling (Table 2). The onset temperatures measured by the DSC were 0.4 - 3.0℃ lower than those obtained by the rheometer. Obtura had the highest onset temperature and Meta had the lowest (p < 0.05). The peak phase transition temperatures determined by the DSC were 2.7 - 3.5℃ lower than the onset temperatures. In all cases, Obtura showed the highest temperature and Meta had the lowest (p < 0.05). The transition temperatures of Resilon and Diadent were in between, and the two groups were not significantly different from each other.
The consistency of the filling materials under squeezing pressure was compared at 60℃ and 40℃ (Figure 2). Obtura exhibited the highest normal stress value at 60℃, followed by Diadent, Resilon, and Meta in descending order (p < 0.05). For all materials, the normal stress measured at 40℃ was higher than two times the value measured at 60℃. Obtura had the highest stress value and Diadent was ranked as the second highest (p < 0.05). Resilon and Meta were not significantly different from each other.
The purpose of this study was to evaluate the rheological behaviors of three different gutta perchas and Resilon materials during a transition of temperature. The manufacturer's instructions suggest that Resilon pellets can be used with the Obtura-II system in a low temperature setting (140℃). Therefore, we applied two different setting temperatures: 140℃ with regards to the recommendation for Resilon and 200℃ based on a regular protocol for standard gutta percha pellets.
The importance of extrusion temperature is that it greatly affects the viscoelastic properties of filling materials during the injection, cooling, and compaction process in root canals, and potentially causes thermal damage to the peri-radicular tissue. If the extrusion temperature is higher, the material flows better in the root canal and seals the wall with better adaptability, but the possibility of tissue damage from the heat may also be increased. On the other hand, too low of an extrusion temperature decreases the flow resulting in the incomplete seal of the canal space.
Our study showed that the extruded Resilon material had a lower temperature (55℃) than the three gutta perchas (61.4 - 65.1℃) when warmed to 140℃. The extrusion temperature of Resilon was just 9℃ above the phase transition temperature (46℃), which means that the viscosity of the materials started to increase steeply in a few seconds by cooling. Karr et al.9 reported that the injection of Resilon using a 23-G needle with the Obtura-II system set at 140℃ required greater force than gutta percha at the same temperature. This effect could be explained from the thermoplasticized Resilon having a higher specific heat and absorbing more heat during melting than gutta percha. Therefore, Resilon may not be warmed as much as gutta perchas in the low temperature setting (140℃).1
When Resilon was heated with the Obtura-II system set at 200℃, its extrusion temperature (84.4℃) was higher than that of the gutta perchas. This observation can be attributed to its higher specific heat. The heat loss from the Resilon during extrusion through the 23-G needle was lower than that of the gutta perchas.
As shown in Figure 1, the magnitudes of G', G", tan δ, and η* at a specific temperature, and the onset temperatures of the rheological phase transition of the filling materials were significantly different among the materials. Meta showed the lowest values of G', G", and η* within a range of 39 - 120℃, and had the lowest onset transition temperature (39℃). These values characterized Meta as the highest flowing gutta percha during injection and indicate that it can be molded into the canal space until the temperature cools to just 2℃ above body temperature. The G', G", and η* values of Obtura in the range of 49.5 - 120℃ were the highest and Obtura exhibited the highest onset transition temperature (49.5℃). These findings mean that Obtura flows least during injection and starts to harden the earliest at a relatively high temperature. Therefore, Obtura material should be quickly packed into the canal before the temperature reaches 49.5℃. The viscoelastic profile of Resilon resembled the profiles of the three gutta perchas.
When the materials were cooled down to 40 - 50℃, there was a drastic increase in G' and G" by 100 - 1,000 times (Figures 1a and 1b). The loss tangent (G"/G') decreased from 0.8 - 2.6 to 0.08 - 0.32, which meant that the increase in G' was more magnified than that of G" (Figure 1c). It implied that the visco-plastic and soft materials became more elastic and stiffer over time under a cooling process. Resilon showed the greatest decrease in tan δ values at the transition temperature near 40℃, which means that the phase transition of Resilon was maximized among the materials.
During condensation of the injected materials, if the filling material is too warm and highly plasticized, a condenser will simply penetrate it. On the other hand, when the material is already cooled before compaction, it will resist plastic deformation. Without sufficient compaction, voids will be created inside the obturated canal.10 When too much excessive force is delivered to the stiffened material, it will result in iatrogenic vertical root fractures.9 A combination of controlled compaction stress and timely manipulation needs to be in accordance with the material consistency, particularly when the material rapidly undergoes phase transition with decreasing temperature.
The G' and G" of warm gutta percha and Resilon indicates the behavior of a partially crystalline viscoelastic polymer that largely depends on the molecular weight and distribution.11 The composition of commercial gutta percha has been shown to be 18 - 22% gutta-percha polymer and 37 - 75% zinc oxide.12 Resilon is composed of 57% polycaprolactone and 42% inorganic fillers.13 A low percentage of gutta percha polymer composition resulted in a decreased flow rate3,14 such that the viscosity of the resin composite increased with increasing filler contents and was also influenced by the filler size and configuration.15
The squeeze test was performed to compare the consistency of materials which is related to handling characteristics in a clinical situation. In a squeeze test, 60℃ and 40℃ were chosen as the representative temperatures before and after the major rheological phase transition of the four filling materials during cooling.16 The normal stress caused by squeezing pressure greatly differed between the temperatures of 60℃ and 40℃. For all materials, the normal stress measured at 40℃ was more than double that measured at 60℃. Obtura manifested the highest viscosity and the greatest stress with compaction force (Figures 1d and 2). The material also exhibited the highest onset and peak temperature among the four canal filling materials during the phase transition (Table 2). The clinical implication that comes from these findings may be that the injection of Obtura needs to be followed by quicker and harder compaction compared to the other canal filling materials. Resilon was similar in consistency to Meta, both of which were relatively high-flow materials in this study.
The onset temperature determined by the DSC thermogram indicated the point at which the exothermic reaction commenced as temperature decreased. Before the peak temperature was reached, which was lower than the onset temperature by 2 - 3℃, the enthalpy change promoted the phase transition. We determined the onset temperature through two methodologies, rheometry and calorimetry. In a dynamic mechanical technique used in rheometry, a small oscillating mechanical strain is exercised on a viscoelastic material, allowing stress and strain to be resolved into their in-phase (storage) and out-of-phase (loss) components.17 Our measurement results from the rheometer and DSC differed by less than 3℃. These results meant that the onset of molecular motion accompanied by energy transfer corresponded with the enthalpy change of the material.
Based on our measurements, the three commercial thermoplasticized gutta perchas had different viscoelastic properties with varied temperature, which would be clinically related to the handling characteristics and compaction efficacy. Resilon was similar to the gutta perchas in rheological behaviors over varied temperatures when they were used with the Obtura- II system. The rhelogical properties of Resilon were located between the properties of high and low-flow gutta perchas.
Figures and Tables
Figure 1
Rheological characteristics of the three gutta perchas and Resilon as a function of temperature. (a) Storage (elastic) modulus, G'; (b) Loss (viscous) modulus, G"; (c) Loss tangent, tan δ; (d) Complex viscosity, η*. OBT, Obtura; DIA, Diadent; MET, Meta; RES, Resilon.
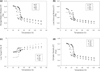
Figure 2
Comparison of the consistency of the canal filling materials using a squeeze test at 60℃ and 40℃. OBT, Obtura; DIA, Diadent; MET, Meta; RES, Resilon.
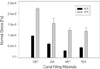
References
1. Miner MR, Berzins DW, Bahcall JK. A comparison of thermal properties between gutta-percha and a synthetic polymer based root canal filling material (Resilon). J Endod. 2006. 32:683–686.


3. Karabucak B, Kim A, Chen V, Iqbal MK. The comparison of gutta-percha and Resilon penetration into lateral canals with different thermoplastic delivery systems. J Endod. 2008. 34:847–849.


4. Combe EC, Cohen BD, Cummings K. Alpha- and beta-forms of gutta-percha in products for root canal filling. Int Endod J. 2001. 34:447–451.


5. Elzubair A, Elias CN, Suarez JC, Lopes HP, Vieira MV. The physical characterization of a thermoplastic polymer for endodontic obturation. J Dent. 2006. 34:784–789.


6. Nielsen BA, Baumgartner JC. Spreader penetration during lateral compaction of resilon and gutta-percha. J Endod. 2006. 32:52–54.


7. Lee IB, Cho BH, Son HH, Um CM. Rheological characterization of composites using a vertical oscillation rheometer. Dent Mater. 2007. 23:425–432.


8. Venturi M, Di Lenarda R, Breschi L. An ex vivo comparison of three different gutta-percha cones when compacted at different temperatures: rheological considerations in relation to the filling of lateral canals. Int Endod J. 2006. 39:648–656.


9. Alicia Karr N, Baumgartner JC, Marshall JG. A comparison of gutta-percha and Resilon in the obturation of lateral grooves and depressions. J Endod. 2007. 33:749–752.


10. Venturi M, Pasquantonio G, Falconi M, Breschi L. Temperature change within gutta-percha induced by the System-B Heat Source. Int Endod J. 2002. 35:740–746.


11. Tsukada G, Tanaka T, Torii M, Inoue K. Shear modulus and thermal properties of gutta percha for root canal filling. J Oral Rehabil. 2004. 31:1139–1144.


12. Gurgel-Filho ED, Andrade Feitosa JP, Teixeira FB, Monteiro de Paula RC, Araujo Silva JB, Souza-Filho FJ. Chemical and X-ray analyses of five brands of dental gutta-percha cone. Int Endod J. 2003. 36:302–307.


13. Williams C, Loushine RJ, Weller RN, Pashley DH, Tay FR. A comparison of cohesive strength and stiffness of Resilon and gutta-percha. J Endod. 2006. 32:553–555.


14. Gurgel-Filho ED, Feitosa JP, Gomes BP, Ferraz CC, Souza-Filho FJ, Teixeira FB. Assessment of different gutta-percha brands during the filling of simulated lateral canals. Int Endod J. 2006. 39:113–118.


15. Lee JH, Um CM, Lee IB. Rheological properties of resin composites according to variations in monomer and filler composition. Dent Mater. 2006. 22:515–526.


16. Schilder H, Goodman A, Aldrich W. The thermomechanical properties of gutta-percha. 3. Determination of phase transition temperatures for gutta-percha. Oral Surg Oral Med Oral Pathol. 1974. 38:109–114.