Abstract
Purpose
Asthma is a pulmonary chronic inflammatory disease characterized by airway obstruction and hyperresponsiveness. Pattern recognition receptors are known to play a key role in the development of allergic diseases as well as host defenses against microbial infection. Receptor interacting protein 2 (RIP2), a serine/threonine kinase, is an adaptor molecule of NOD1 and NOD2, and genetic variation in this receptor is known to be associated with the severity of allergic asthma in children. In this study, we examined the role of RIP2 in the development of allergic airway inflammation in a mouse model.
Methods
Airway inflammation was induced in mice through intranasal administration of ovalbumin (OVA) after 2 intraperitoneal immunizations with OVA. Lung inflammation and mucus hypersecretion were examined histologically and total cell infiltration in bronchoalveolar (BAL) fluids was determined. Levels of the Th2-related cytokines, IL-5 and IL-13, in lung extracts were measured by ELISA. Serum antigen-specific IgE and IgG1 levels were also assessed.
Results
OVA-induced lung inflammation and mucus hypersecretion were not different between WT and RIP2-deficient mice. The IL-5 and IL-13 levels in the bronchoalveolar (BAL) fluids were also not impaired in RIP2-deficient mice compared to WT mice. Moreover, RIP2 deficiency did not affect serum OVA-specific IgG1 and IgE levels.
Asthma is the most common chronic lung disease, affecting more than 300 million people of all ages, and the number of patients is increasing by 50% per decade worldwide.1,2 This disease is characterized by airway hyperreactivity and mucous oversecretion that result in intermittent airway obstruction.3,4 Asthma is considered to be an allergic, eosinophilic, and Th2-mediated disease.5 Th2-cell-derived cytokines such as IL-5 and IL-13 play a critical role in the type 2 immune response involved in allergen-induced airway inflammation. IL-5 affects the differentiation and maturation of eosinophils, while IL-13 acts on epithelial and smooth muscle cells and plays a role in airway hyperresponsiveness in allergen-induced asthma.6,7 Serum IgE and IgG1 levels are important markers related to the pathogenesis of allergic asthma; asthma patients have increased serum levels of IgE and IgG1.8 Antigen-specific IgE can attach to receptors on mast cell, basophils, B lymphocytes, and eosinophils.9 This interaction results in the release of inflammatory mediators such as histamine, prostaglandins, leukotrienes, proteases, growth factors, cytokines, and chemokines; these are required for the development of allergic asthma.10 Interaction of antigen with IgE bound to receptors on the cell surface activates mast cells to release preformed mediators such as histamine, leukotrienes, prostaglandin D2, thromboxane B2, and platelet-activating factors. These mediators induce airway smooth muscle contraction, edema, and enhanced mucous secretion, leading to airflow limitation.11
Nod-like receptors (NLRs) belong to a family of cytosolic receptors that are responsible for the recognition of microbial molecules and danger signals. The first identified NLRs, NOD1 and NOD2, have an N-terminal caspase-recruitment domain (CARD), intermediate Nod, and C-terminal leucine-rich repeats (LRRs). They sense the bacterial peptidoglycan components, meso-diaminopimelic acid (meso-DAP) and muramyl dipeptide (MDP), respectively.12 Following recognition, NOD1 and NOD2 recruit a serine/threonine kinase, receptor interacting protein 2 (RIP2 [also known as RICK and CARDIAK]), which has an N-terminal kinase domain and C-terminal CARDs linked by an intermediate region. Association between NOD1 or NOD2 and RIP2 mediated by CARD-CARD interactions induces the activation of NF-κB and mitogen-activated protein kinases (MAPKs), subsequently leading to the production of proinflammatory mediators.13-15
Studies have shown that NOD1 and NOD2 signaling is involved in allergic disease. Genetic variations of NOD1 are associated with asthma and elevated IgE levels in humans.16 NOD1 polymorphisms are also significantly associated with alteration in the strong protective effect which exposure to a farming environment has on allergies.17 In addition, children with the polymorphic allele C2722 of the NOD2 gene are at greater risk of developing allergic rhinitis and atopic dermatitis.18 There is also evidence of an association between the adaptor molecule RIP2 and asthma. Nakashima et al.19 suggested that genetic variants of the RIP2 gene may be associated with the severity of asthma, even though these variants are not likely to be involved in asthma development. Moreover, blockade of RIP2 by the flavonoid aglycone, naringenin, contributed to the suppression of the production of thymic stromal lymphopoietin (TSLP) in mast cells, which play a pivotal role in allergic asthma.20 These findings suggest that RIP2 may be associated with the development of allergic asthma, and prompted us to determine the exact role of RIP2 in the development of allergic airway inflammation. We developed a mouse model of ovalbumin (OVA)-induced airway inflammation using WT and RIP2-deficient mice and examined the severity of lung inflammation, Th2-derived cytokine levels in lung extracts, and serum immunoglobulin levels.
Wild-type (WT) C57BL/6 mice, 6- to 8-weeks-old, were purchased from KOATECH (Pyeongtaek, Gyeonnggi-do, Korea). RIP2-deficient C57BL/6 mice were purchased from The Jackson Laboratory (Bar Harbor, ME, USA). All animal experiments were approved by the Institutional Animal Care and Use Committee of Konyang University.
Protocols are depicted schematically in Fig. 1A. Both WT and RIP2-deficient mice were sensitized with 40 µg OVA (Sigma-Aldrich, St. Louis, MO, USA) and 2 mg of adjuvant (Imject® Alum, Thermo scientific, Rockford, IL, USA) in 200 µL of PBS, or with PBS alone by intraperitoneal (i.p.) injection on days 0, 1, 7, and 8. On days 14, 15, 21, and 22, anesthetized mice were challenged intranasally (i.n.) with 200 µg of OVA in PBS or with PBS alone in a volume of 50 µL. Animals were sacrificed 2 days after the last challenge and bronchoalveolar lavage (BAL) fluids, serum, and lung tissues were collected for analysis.
Lung extracts were obtained using a tissue homogenizer. Homogenates were centrifuged at 1,000×g for 10 min. Supernatants were collected, and then stored at -70℃ for analysis. IL-5 and IL-13 levels were measured using a commercial enzyme-linked immunosorbent assay (ELISA) kit (R&D Systems, Abingdon, U.K.). For the measurement of OVA-specific IgE levels, 96-well ELISA plates were coated with OVA (10 µg/mL) at 4℃ overnight. Nonspecific binding was blocked with 1% BSA in PBS, and serum samples (at 1:20 dilution) were added to the plate. After incubation for 2 h at room temperature, biotin rat anti-mouse IgE (BD Biosciences, San Jose, CA, USA) was applied, followed by streptavidin HRP (BD Biosciences). After washing, the TMB substrate reagent set (BD Biosciences) was applied according to the manufacturer's instructions and optical density (OD) at 450 nm was measured. OVA-specific IgG1 levels were determined using the methods described above, except that peroxidase-conjugated rat anti-mouse IgG1 (Southern Biotech, Birmingham, AL, USA) was used.
After anesthesia by intraperitoneal injection of Zoletile (Virbac Laboratories, Carros, France), BAL fluid was obtained by lavage with 0.8 mL of PBS via a tracheal catheter. The BAL fluid was centrifuged at 200×g for 3 min at 4℃. After discarding the supernatant, we resuspended the cell pellet in cold RPMI 1640 medium. Total cell numbers in the lavage fluid were counted using a hemocytometer. A differential cell count was performed using Diff-Quick staining on the basis of morphological criteria.
To evaluate tissue inflammation, the left lung of each mouse was fixed in 10% neutral-buffered formalin for 48 h and then embedded in paraffin. Tissue sections (2 µm thick) were prepared and stained with hematoxylin and eosin (HE) or periodic acid-Schiff (PAS), and examined under a light microscope. Tissue inflammation scoring was based on the presence or abundance of inflammatory lesions as follows: 0, non-specific; 1, mild; 2, mild to moderate; 3, moderate; 4, moderate to severe; 5, severe. To quantitate mucus staining, PAS-positive cells in the airways were counted, and the length of the basement membrane (BM) in each airway was measured using ImageJ version 1.44 (National Institutes of Health, Bethesda, MD, USA). The results are reported as the mean numbers of PAS-positive cells per 100 micrometers of BM.21
The significance of differences in mean values of the groups was evaluated by t-tests, and values are expressed as means±SD. All statistical calculations were performed using GraphPad Prism version 4 (GraphPad Software, San Diego, CA, USA). Value of P<0.05 was considered to indicate significance.
Airway inflammation in mice was achieved by challenge with OVA 4 times i.n. after 4 immunizations by i.p. injection, as described in Fig. 1A. We first examined whether RIP2 affected the severity of airway inflammation. Intra-nasal challenge with OVA induced severe infiltration of inflammatory cells, consisting mostly of lymphocytes and granulocytes, around the bronchus, and increased the thickness of the alveolar walls in both WT and RIP2-deficient mice (Fig 1B). However, when histopathological scores were assessed, RIP2 deficiency did not appear to affect the severity of lung inflammation induced by OVA (Fig. 1C). In addition, total infiltrating cells were counted in the BAL fluids of mice with and without i.n. challenge by OVA. Compared to the PBS-treated mice, OVA challenge increased the number of infiltrating cells in the BAL fluids of both WT and RIP2-deficient mice, with no significant difference between the WT and RIP2-deficient mice (Fig. 1D). When a differential cell count was performed using Diff-Quick staining, intranasal challenge with OVA led to a decrease in the percentage of macrophages and an increase in eosinophils and neutrophils in the BAL fluids of mice (Fig. 1E). However, there was no significant difference between WT and RIP2-deficient mice (Fig. 1E).
OVA-induced allergic airway inflammation is characterized by hyperplasia of goblet cells and mucus hypersecretion in the bronchus. To determine whether RIP2 deficiency influenced these phenomena, slide sections were stained with PAS and observed under a light microscope. As shown in Fig. 2 A and B, the number of PAS-positive cells as well as mucus secretion in the airway epithelial layer of mice was increased by intranasal challenge with OVA. However, there was no significant difference in the number of PAS-positive cells or mucus secretion between WT and RIP2-deficient mice (Fig. 2 A and B).
We examined OVA-induced IL-5 and IL-13 levels in lung extracts of WT and RIP2-deficient mice. Results showed that i.n. challenge with OVA increased the IL-5 and IL-13 levels in the lungs of mice (Fig. 3 A and B). However, RIP2 deficiency did not affect OVA-induced IL-5 and IL-13 levels in lung extracts (Fig. 3 A and B).
Finally, we measured the serum antigen-specific IgE and IgG1 levels. As expected, antigen-specific IgE and IgG1 levels were increased by OVA challenge (Fig. 4 A and B). However, there were no significant differences in OVA-specific IgE and IgG1 levels between WT and RIP2-deficient mice (Fig. 4 A and B).
Allergic asthma is a chronic airway inflammatory disease related to dysfunction of the airway caused by the release of inflammatory mediators, and remodeling of the airway wall.22 In allergic asthma, exacerbation of the underlying airway inflammation is associated with symptoms and airway limitations.11 Pattern recognition receptors (PRRs) initiate immune responses by recognizing structures of microorganisms and endogenous molecules released from damaged cells, and regulate the transcription of genes involved in inflammatory responses.23 In addition to their protective role in bacterial or viral infection, PRRs have been reported to be involved in allergic asthma. Toll-like receptors (TLRs), the best characterized PRRs, are known to mediate the induction of allergic airway inflammation. There is also evidence of a close association between polymorphisms in TLR genes and asthma.24-26 In addition, several studies have revealed that TLR stimulation exacerbates or alleviates allergen-induced asthma depending on the TLR type.27,28 Immunization with OVA and a TLR2 agonist (Pam3Cys) induced Th2 immune responses such as the production of antigen-specific IgE in the serum, and IL-13 secretion by splenocytes.27 TLR2 activation promoted airway hyperresponsiveness.27 Double-stranded RNA (dsRNA) increased lung inflammation, airway hyperresponsiveness, and antigen-specific Th2 responses in OVA-sensitized mice through the TLR3-TRIF (Toll/IL-1R domain-containing adaptor-inducing IFN-β) pathway.28 In contrast, intranasal administration of the TLR7 agonist, R848, suppressed experimental asthma by inducing type ? interferon production and inhibiting Th2 responses.29 Oral administration of CpG-ODN, a TLR9 agonist, prevented eosinophilic airway inflammation.30 These findings suggest that innate immune responses mediated by PRRs play a critical role in the development of allergic airway inflammation.
Similar to TLRs, NOD1 and NOD2 stimulation triggers the activation of NF-κB and MAPKs, which are critical factors for the production of proinflammatory cytokines; the adaptor molecule RIP2 is required for this.15 However, in contrast to TLRs, the association between NOD1 and NOD2 signaling and allergic asthma is poorly understood. Based on several indirect lines of evidence of its involvement in allergic asthma,19,20 we sought to determine the role of RIP2 in OVA-induced airway inflammation. We found no significant differences between WT and RIP2-deficient mice in terms of the severity of lung inflammation, total cell infiltration in BAL fluid, IL-5 and IL-13 levels in lung extracts, or serum antigen-specific IgE and IgG1 levels of mice challenged intranasally with OVA. A recent study showed that serum samples from normal mice, but not antibiotic-treated mice, had NOD1- and NOD2-stimulating activity,31 suggesting that microbiota may steadily release NOD1- and NOD2-stimulatory factors (e.g., peptidoglycans) into the body fluid. Therefore, in this study, we compared various parameters between WT and RIP2-deficient mice in the absence of NOD1 and NOD2 stimulation to mimic physiological conditions. Consistent with the findings of a previous study,32 we found that WT and RIP2-deficient mice immunized with OVA and alum without NOD1 and NOD2 ligands, did not show differences in serum antigen-specific IgG levels. Eosinophilic infiltration into the lung by OVA was also not impaired in RIP2-deficient mice compared with WT mice.33 In addition, RIP2 is not essential for T-cell proliferation and differentiation.34 Taken together, our results indicate that under normal conditions, RIP2 deficiency is not associated with the development of allergic airway inflammation. Nevertheless, it is necessary to define the role of RIP2 in the development of allergic disease under NOD1 and NOD2 activation; Magalhaes et al.32 showed that RIP2 is required for NOD1- and NOD2-induced Th2 immunity. NOD1 and NOD2 ligands increased the number of OVA-specific cells producing IL-4 and IL-5 in the splenocytes of WT mice, but not in RIP2-deficient mice.32 In addition, RIP2 is essential for OVA-specific IgG1 production, mediated by NOD1 and NOD2 stimulation.32 These findings suggest that RIP2 may affect the development of allergic disease mediated by NOD1 and NOD2 activation.
Microbial infections are thought to affect the development or severity of allergic asthma through TLR-mediated signaling.33,35 NOD1 and NOD2 cooperate with TLRs to induce the innate immune response against microbial infections.36 Listeria-induced production of cytokines was impaired in NOD1/2 double- or RIP2-deficient macrophages after LPS exposure.37 RIP2 deficiency also led to decreased production of cytokines in TLR-tolerized macrophages in response to Pseudomonas infection, and protected mice from lethality induced by the bacterial infection.38 Therefore, it is necessary to clarify whether NOD1/2 and RIP2 contribute to the control of the development of allergic diseases mediated by microbial infection.
Figures and Tables
Fig. 1
OVA-induced airway inflammation in WT and RIP2-deficient mice. A schematic diagram of the experimental design (A). Mice were sensitized by i.p. administration of OVA mixed with adjuvant at days 0, 1, 7, and 8. On days 14, 15, 21, and 22, mice were challenged with OVA or PBS. Photographs of lung tissues were obtained from H&E-stained sections (B) and histopathological scores were determined semi-quantitatively by microscopic examination (C). Total cell numbers in the BAL fluids were counted (D) and a differential cell count was performed using Diff-Quick staining (E). Data are expressed as means±SD.
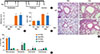
Fig. 2
Hyperplasia of goblet cells and mucus hypersecretion in the bronchus of lung tissue. Lung sections were stained with PAS and examined by light microscopy. Areas in the black boxes are shown at a higher magnification on the right of each picture (A). Numbers of PAS-positive cells in the airway epithelium were counted. In each airway studied, the length of the basement membrane (BM) was measured using image analysis software. The results are presented as mean numbers of PAS-positive cells per micrometer of BM (B). Data are expressed as means±SD.
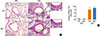
ACKNOWLEDGMENTS
This study was supported by a grant from the Korean Health Technology R&D project, the Ministry of Health & Welfare, Republic of Korea (Grant No. A111025).
References
1. Masoli M, Fabian D, Holt S, Beasley R. Global Initiative for Asthma (GINA) Program. The global burden of asthma: executive summary of the GINA Dissemination Committee report. Allergy. 2004; 59:469–478.
2. To T, Stanojevic S, Moores G, Gershon AS, Bateman ED, Cruz AA, Boulet LP. Global asthma prevalence in adults: findings from the cross-sectional world health survey. BMC Public Health. 2012; 12:204.
3. Busse WW, Lemanske RF Jr. Asthma. N Engl J Med. 2001; 344:350–362.
4. Shifren A, Witt C, Christie C, Castro M. Mechanisms of remodeling in asthmatic airways. J Allergy (Cairo). 2012; 2012:316049.
5. Wenzel SE. Asthma phenotypes: the evolution from clinical to molecular approaches. Nat Med. 2012; 18:716–725.
6. Nakajima H, Takatsu K. Role of cytokines in allergic airway inflammation. Int Arch Allergy Immunol. 2007; 142:265–273.
7. Wills-Karp M, Luyimbazi J, Xu X, Schofield B, Neben TY, Karp CL, Donaldson DD. Interleukin-13: central mediator of allergic asthma. Science. 1998; 282:2258–2261.
8. Shakib F, Sihoe J, Smith SJ, Wilding P, Clark MM, Knox A. Circulating levels of IgG1 and IgG4 anti-IgE antibodies and asthma severity. Allergy. 1994; 49:192–195.
9. Platts-Mills TA. The role of immunoglobulin E in allergy and asthma. Am J Respir Crit Care Med. 2001; 164:S1–S5.
10. Kuhl K, Hanania NA. Targeting IgE in asthma. Curr Opin Pulm Med. 2012; 18:1–5.
11. Jarjour NN, Kelly EA. Pathogenesis of asthma. Med Clin North Am. 2002; 86:925–936.
12. Hasegawa M, Fujimoto Y, Lucas PC, Nakano H, Fukase K, Núñez G, Inohara N. A critical role of RICK/RIP2 polyubiquitination in Nod-induced NF-kappaB activation. EMBO J. 2008; 27:373–383.
13. Inohara N, Koseki T, Lin J, del Peso L, Lucas PC, Chen FF, Ogura Y, Núñez G. An induced proximity model for NF-kappa B activation in the Nod1/RICK and RIP signaling pathways. J Biol Chem. 2000; 275:27823–27831.
14. Kobayashi K, Inohara N, Hernandez LD, Galán JE, Núñez G, Janeway CA, Medzhitov R, Flavell RA. RICK/Rip2/CARDIAK mediates signalling for receptors of the innate and adaptive immune systems. Nature. 2002; 416:194–199.
15. Chen G, Shaw MH, Kim YG, Nuñez G. NOD-like receptors: role in innate immunity and inflammatory disease. Annu Rev Pathol. 2009; 4:365–398.
16. Hysi P, Kabesch M, Moffatt MF, Schedel M, Carr D, Zhang Y, Boardman B, von Mutius E, Weiland SK, Leupold W, Fritzsch C, Klopp N, Musk AW, James A, Nunez G, Inohara N, Cookson WO. NOD1 variation, immunoglobulin E and asthma. Hum Mol Genet. 2005; 14:935–941.
17. Eder W, Klimecki W, Yu L, von Mutius E, Riedler J, Braun-Fahrländer C, Nowak D, Holst O, Martinez FD. ALEX-Team. Association between exposure to farming, allergies and genetic variation in CARD4/NOD1. Allergy. 2006; 61:1117–1124.
18. Kabesch M, Peters W, Carr D, Leupold W, Weiland SK, von Mutius E. Association between polymorphisms in caspase recruitment domain containing protein 15 and allergy in two German populations. J Allergy Clin Immunol. 2003; 111:813–817.
19. Nakashima K, Hirota T, Suzuki Y, Akahoshi M, Shimizu M, Jodo A, Doi S, Fujita K, Ebisawa M, Yoshihara S, Enomoto T, Shirakawa T, Kishi F, Nakamura Y, Tamari M. Association of the RIP2 gene with childhood atopic asthma. Allergol Int. 2006; 55:77–83.
20. Moon PD, Choi IH, Kim HM. Naringenin suppresses the production of thymic stromal lymphopoietin through the blockade of RIP2 and caspase-1 signal cascade in mast cells. Eur J Pharmacol. 2011; 671:128–132.
21. Mäkelä MJ, Kanehiro A, Dakhama A, Borish L, Joetham A, Tripp R, Anderson L, Gelfand EW. The failure of interleukin-10-deficient mice to develop airway hyperresponsiveness is overcome by respiratory syncytial virus infection in allergen-sensitized/challenged mice. Am J Respir Crit Care Med. 2002; 165:824–831.
22. Holgate ST. Innate and adaptive immune responses in asthma. Nat Med. 2012; 18:673–683.
23. Takeuchi O, Akira S. Pattern recognition receptors and inflammation. Cell. 2010; 140:805–820.
24. Basu S, Fenton MJ. Toll-like receptors: function and roles in lung disease. Am J Physiol Lung Cell Mol Physiol. 2004; 286:L887–L892.
25. Cook DN, Pisetsky DS, Schwartz DA. Toll-like receptors in the pathogenesis of human disease. Nat Immunol. 2004; 5:975–979.
26. Eder W, Klimecki W, Yu L, von Mutius E, Riedler J, Braun-Fahrländer C, Nowak D, Martinez FD. ALEX Study Team. Toll-like receptor 2 as a major gene for asthma in children of European farmers. J Allergy Clin Immunol. 2004; 113:482–488.
27. Redecke V, Häcker H, Datta SK, Fermin A, Pitha PM, Broide DH, Raz E. Cutting edge: activation of Toll-like receptor 2 induces a Th2 immune response and promotes experimental asthma. J Immunol. 2004; 172:2739–2743.
28. Torres D, Dieudonné A, Ryffel B, Vilain E, Si-Tahar M, Pichavant M, Lassalle P, Trottein F, Gosset P. Double-stranded RNA exacerbates pulmonary allergic reaction through TLR3: implication of airway epithelium and dendritic cells. J Immunol. 2010; 185:451–459.
29. Xirakia C, Koltsida O, Stavropoulos A, Thanassopoulou A, Aidinis V, Sideras P, Andreakos E. Toll-like receptor 7-triggered immune response in the lung mediates acute and long-lasting suppression of experimental asthma. Am J Respir Crit Care Med. 2010; 181:1207–1216.
30. Kitagaki K, Businga TR, Kline JN. Oral administration of CpG-ODNs suppresses antigen-induced asthma in mice. Clin Exp Immunol. 2006; 143:249–259.
31. Clarke TB, Davis KM, Lysenko ES, Zhou AY, Yu Y, Weiser JN. Recognition of peptidoglycan from the microbiota by Nod1 enhances systemic innate immunity. Nat Med. 2010; 16:228–231.
32. Magalhaes JG, Lee J, Geddes K, Rubino S, Philpott DJ, Girardin SE. Essential role of Rip2 in the modulation of innate and adaptive immunity triggered by Nod1 and Nod2 ligands. Eur J Immunol. 2011; 41:1445–1455.
33. Nembrini C, Sichelstiel A, Kisielow J, Kurrer M, Kopf M, Marsland BJ. Bacterial-induced protection against allergic inflammation through a multicomponent immunoregulatory mechanism. Thorax. 2011; 66:755–763.
34. Hall HT, Wilhelm MT, Saibil SD, Mak TW, Flavell RA, Ohashi PS. RIP2 contributes to Nod signaling but is not essential for T cell proliferation, T helper differentiation or TLR responses. Eur J Immunol. 2008; 38:64–72.
35. Conrad ML, Ferstl R, Teich R, Brand S, Blümer N, Yildirim AO, Patrascan CC, Hanuszkiewicz A, Akira S, Wagner H, Holst O, von Mutius E, Pfefferle PI, Kirschning CJ, Garn H, Renz H. Maternal TLR signaling is required for prenatal asthma protection by the nonpathogenic microbe Acinetobacter lwoffii F78. J Exp Med. 2009; 206:2869–2877.
36. Kim YG, Park JH, Reimer T, Baker DP, Kawai T, Kumar H, Akira S, Wobus C, Núñez G. Viral infection augments Nod1/2 signaling to potentiate lethality associated with secondary bacterial infections. Cell Host Microbe. 2011; 9:496–507.
37. Kim YG, Park JH, Shaw MH, Franchi L, Inohara N, Núñez G. The cytosolic sensors Nod1 and Nod2 are critical for bacterial recognition and host defense after exposure to Toll-like receptor ligands. Immunity. 2008; 28:246–257.
38. Park JH, Kim YG, Núñez G. RICK promotes inflammation and lethality after gram-negative bacterial infection in mice stimulated with lipopolysaccharide. Infect Immun. 2009; 77:1569–1578.