Abstract
Purpose
Prostaglandin (PG) E2 is an immunomodulatory lipid mediator generated mainly via the cyclooxygenase-2 (COX-2) pathway from arachidonic acid at sites of infection and inflammation. A positive feedback loop of PGE2 on COX-2 expression is critical for homeostasis during toll-like receptor (TLR)-mediated inflammatory processes. The mechanism of PGE2-regulated COX-2 expression remains poorly understood. The low-molecular-weight stress protein heme oxygenase-1 (HO-1) contributes to the anti-inflammatory, anti-oxidant and anti-apoptotic response against environmental stress.
Methods
We explored the involvement of HO-1 on PGE2 regulation of LPS-induced COX-2 expression in RAW 264.7 macrophages.
Results
LPS-induced COX-2 expression in RAW 264.7 macrophages was enhanced by exogenous PGE2 or cyclic AMP (cAMP) analogue and was suppressed by a COX inhibitor (indomethacin), a protein kinase A (PKA) inhibitor (KT5720), and A kinase anchoring protein (AKAP) disruptors (Ht31 and RIAD). This result suggests that the stimulatory effects of endogenous and exogenous PGE2 on COX-2 expression are mediated by a cAMP-PKA-AKAP-dependent pathway. The induction of HO-1 was observed in LPS-stimulated RAW 264.7 macrophages. This induction was suppressed by exogenous PGE2 and enhanced by blockage of the endogenous PGE2 effect by the PKA inhibitor or AKAP disruptors. In addition, HO-1 induction by the HO activator copper protoporphyrin suppressed LPS-induced COX-2 expression, which was restored by the addition of exogenous PGE2. The induction of HO-1 inhibited LPS-induced NF-κB p-65 nuclear expression and translocation.
Cyclooxygenase (COX) converts arachidonic acid into prostaglandin H2, which is the rate-limiting step in prostaglandin (PG) formation including prostaglandin E2 (PGE2). At least two isoforms of COX have been identified in mammalian cells: COX-1 is constitutively expressed, whereas COX-2 is inducible in response to growth factors, oncogenes, cytokines, and endotoxin.1 COX-2 expression is upregulated at sites of inflammation and in various carcinomas.2 Lipopolysaccharide (LPS), the main cell wall component of Gram-negative bacteria, activates inflammatory responses through toll-like receptor 4 (TLR4), and LPS-activated macrophages show a high expression of COX-2.3
The immunomodulatory effects of PGE2 result largely from its ability to increase intracellular cyclic AMP (cAMP) through the G protein-coupled E prostanoid (EP) receptors EP2 and EP4.4 Increases in intracellular cAMP serve as an important brake on excessive inflammatory activation in macrophages.5 The classical cAMP effector is protein kinase A (PKA), which is composed of two regulatory (R) and two catalytic (C) subunits.5 In addition, scaffold proteins, termed A-kinase anchoring proteins (AKAP), involve the assembly of cAMP-dependent pathway components into multiprotein signaling complexes.6
COX is rapidly inactivated after the conversion of arachidonic acid into PGH2. Therefore, rapid synthesis of COX would be required to sustain PG biosynthesis.7 A positive feedback loop between PGE2 and COX-2 is established in various cells.8-10 Furthermore, there is some evidence that the canonical PGE2/EP2/cAMP/PKA/CREB cascade plays a key role in the expression of COX-2 in activated macrophages.11 However, the mechanisms of PGE2 regulation of COX-2 expression remain poorly understood.
The low-molecular-weight stress protein heme oxygenase-1 (HO-1) provides an anti-inflammatory, anti-oxidant, and antiapoptotic response against environmental stress.12-14 Induction of HO-1 attenuates LPS-induced COX expression through suppression of the nuclear factor (NF)-κB pathway.15-17 Despite the critical role of HO-1 in the anti-inflammatory process, little is known about its role in the positive feedback loop of COX-2. Here, we identify an autocrine amplification loop for COX-2 expression composed of PGE2, cAMP, PKA, and AKAP in RAW 264.7 macrophages. Furthermore, suppression of HO-1 by cAMP-PKA-AKAP signaling plays an important role in the positive feedback loop through NF-κB signaling.
Trypan blue, Dulbecco's Modified Eagle's Medium (DMEM), fetal bovine serum (FBS), streptomycin, penicillin, and trypsin were purchased from Gibco BRL (Grand Island, NY, USA). COX-2, NF-κB p65 antibody, anti-mouse IgG, and anti-rabbit IgG horseradish peroxidase antibody were from Cell Signaling (Boston, MA, USA). The HO-1 antibody, PKA inhibitor KT5720, copper protoporphyrin (CoPP), and zinc protoporphyrin (ZnPP) were purchased from Enzo Life Sciences (Victoria, BC, USA). The β-actin antibody was from Abcam (San Francisco, CA, USA). Dibutyryl cAMP (dbcAMP: N6,2'-O-dibutyryladenosine 3':5'-cAMP) was from Calbiochem (San Diego, CA, USA). The RII/AKAP disruptor peptide Ht31 was obtained from Promega (Madison, WI, USA). The RI/AKAP disruptor peptide RIAD was purchased from Anaspec (San Jose, CA, USA). PGE2, indomethacin, Escherichia coli (055:B5) LPS, sucrose, aprotinin, phenylmethylsulfonyl fluoride (PMSF), dithiothreitol (DTT), sodium dodecyl sulfate (SDS), barium chloride (BaCl), and benzene were obtained from Sigma (St. Louis, MO, USA). All other chemicals utilized in this study were of highest purity available from commercial sources.
The murine macrophage cell line RAW 264.7 was obtained from American Type Culture Collection (ATCC). Cells were cultured in DMEM, supplemented with 100 IU/mL penicillin, 100 µg/mL streptomycin, and 10% heat-inactivated FBS, at 37℃ and 5% CO2 in a humidified chamber. The medium was changed at 3 days. The cells were allowed to reach confluence, and subcultured using trypsin.
RAW 264.7 macrophages were washed with cold PBS (pH 7.4) and harvested. The cells were homogenized in nine volumes of 0.3 M sucrose, 0.26 unit/mo aprotinin, 0.1 mM PMSF, and 1 mM DTT, using a Teflon-potter homogenizer with 10 strokes at 900-1,000 rpm. The protein samples (30 µg) were boiled at 100℃ for 10 min, and subjected to 10% SDS-polyacrylamide gel electrophoresis (SDS-PAGE). Protein content was measured using the Bradford method (Bio-Rad protein assay). The separated proteins were transferred to a polyvinylidene difluoride (PVDF) membrane (Immunobilon-P: Millipore, Bedford, MA, USA). After transfer, membranes were blocked with a solution of 5% non-fat dried milk in Tris-buffered saline (TBS) containing 10 mM Tris (pH 8.0) and 150 mM NaCl for 1 h and incubated with the respective primary antibodies (COX-2, 1:1,000; HO-1, 1:1,000; NF-κB p65, 1:1,000; β-actin, 1:10,000) overnight at 4℃. After washing three times with TBS containing 0.05% Tween 20 (TBST), the membranes were hybridized with horseradish peroxidase-conjugated secondary antibodies (1:1,000) for 1 h at room temperature and developed with an enhanced chemiluminescence detection kit (Amersham Pharmacia Biotech. Buckinghamshire, UK). Protein bands were visualized on X-ray film. Relative band densities were determined by densitometric analysis, and the ratio of COX-2 and HO-1 to β-actin was calculated. In all instances, density values of bands were corrected by subtracting the background values.
RAW 264.7 macrophages (3×106 cells/plate) were plated in 100 mm plates. Then, cells were treated with 1 µg/mL LPS in the presence or absence of a tested compound for 1 h, and washed with ice-cold PBS buffer and kept on ice for 1 min. The suspension was mixed with buffer A (10 mM HEPES, pH 7.5, 10 mM KCl, 0.1 mM EGTA, 0.1 mM EDTA, 1 mM DTT, 0.5 mM PMSF, 5 mg/mL aprotinin, 5 mg/mL pepstatin, and 10 mg/mL leupeptin) and lysed via three freeze-thaw cycles. Cytosolic fractions were obtained by centrifugation at 12,000×g for 20 min at 4℃. The pellets were resuspended in buffer B (20 mM HEPES, pH 7.5, 0.4 M NaCl, 1 mM EGTA, 1 mM EDTA, 1 mM DTT, 1 mM PMSF, 5 mg/mL aprotinin, 5 mg/mL pepstatin, and 10 mg/mL leupeptin), kept on ice for 40 min, and centrifuged at 14,000×g for 20 min at 4℃. Levels of NF-κB p65 subunit in the nuclear protein extract were determined by Western blotting analysis as described above.
The activity of HO was measured spectrophotometrically by quantifying the release of bilirubin into the culture media. RAW 264.7 macrophages (2×106 cells/plate) were incubated for varying periods of time, and 0.5 mL each culture supernatant was collected. Then, 250 mg BaCl and 0.75 mL benzene were added and the samples were vortexed for 50-60 sec and centrifuged (30 min at 13,000×g). The upper benzene layers were collected and the absorbance at 450 nm with a reference wave-length at 600 nm was measured with a spectrophotometer (excitation coefficient of 27.3 mM-1·cm-1).
RAW 264.7 cells on cover slips were fixed with 4% paraformaldehyde solution in PBS (pH 8.4; 15 min, room temperature) and permeabilized using 0.1% Triton X-100 solution in PBS (30 min, room temperature). The non-specific binding sites were blocked with 4% bovine serum albumin (BSA) in PBS for 30 min at room temperature and the NF-κB p65 subunit was detected by incubation with rabbit polyclonal antibody (1:50 dilution) for 1 h. Cells were washed three times and incubated with FITC-conjugated anti-donkey-IgG secondary antibody (1:100; Invitrogen) for 1 h. Moreover, all cells were stained with a proper dilution of 4',6'-diamidino-2-phenylindole (DAPI) for 2 min to counter-stain DNA in the nuclei. After extensive washings with PBS, coverslips were mounted onto the glass slides, and cells were photographed with fluorescence microscopy to determine NF-κB translocation. To analyze NF-κB activity, the regions of interest (ROI) were drawn around the cell and nucleus. Mean pixel intensity for each ROI was obtained using image software (i-Solution Lite, iMTechnology, Korea). The following ratio was calculated for each cell to indicate the relative levels of antibody staining in the cell and nucleus: ratio=(nuclear intensity-background intensity)/(cell intensity-background intensity).
Data is expressed as means±SD of at least 3 independent experiments. Statistical analysis was performed using SigmaPlot software version 9.0 (SigmaPlot Software Inc., San Diego, CA, USA). All results were analyzed by one-way ANOVA followed by a multiple comparison test, Scheffe's test. A P value of <0.05 was considered statistically significant.
To determine the role of endogenous prostanoids in LPS-induced COX-2 expression in RAW 264.7 macrophages, cells were challenged with LPS (1 µg/mL) in the absence or presence of the nonselective COX inhibitor indomethacin (5 µM). Indomethacin significantly reduced the LPS-induced expression of COX-2 protein (Fig. 1A), implicating that an endogenous prostanoid potentiates COX-2 expression. LPS-induced COX-2 expression was enhanced by pretreatment of macrophages with PGE2, which partially restored the inhibitory effects of indomethacin (Fig. 1A). This suggests that PGE2 involves the expression of COX-2 enzyme in response to LPS by modulating intracellular signaling events.
Diaz-Munoz et al.9 reported that EP2 signaling is required for the upregulation of COX-2 in activated RAW 264.7 macrophages. Because EP2 generally increases intracellular cAMP, we investigated whether the elevation of the second messenger could augment COX-2 expression. The cAMP analog dibutyryl AMP (1 µM) enhanced LPS-induced COX-2 expression in a manner similar to PGE2 itself (Fig. 1B). In macrophages, cAMP works mainly by activating PKA.5 To delineate whether PKA involves TLR4-induced COX-2 expression, we first treated cells with LPS in the presence or absence of a PKA-specific inhibitor (KT5720, 1 µM) and then added PGE2 to confirm that PKA is indeed responsible for the potentiation of COX-2 expression. As shown in Fig. 1C, the PKA inhibitor attenuated LPS-induced COX-2 expression. Indeed, KT5720 also blocked the potentiating effects of exogenous PGE2. Taken together, these results suggest that cAMP-dependent PKA is responsible for the amplification of COX-2 expression by TLR4. PKA is a holoenzyme that consists of two regulatory (R) subunits, RI and RII, and two catalytic subunits. The R subunits of PKA are important for localization inside the cell. AKAPs bind to both regulatory subunits and serve as scaffold proteins to target PKA for specific microdomains. To determine whether AKAPs are involved in the PKA-dependent enhancement of LPS-induced COX-2 expression, we disrupted the potential PKA RI-AKAP interaction (RIAD peptide) and the PKA RII-AKAP interaction (Ht31 peptide). Cells were treated with PKA RI-specific AKAP disruptor peptide (RIAD, 25 µM) and PKA RII-specific AKAP disruptor peptide (Ht31, 25 µM). Both disruptors attenuated LPS-induced COX-2 expression, although RIAD had a relatively stronger effect on LPS-induced COX-2 expression (Fig. 1D). No additive effect was observed when the two disruptors were used together (data not shown). These data suggest that the enhanced expression of LPS-induced COX-2 by endogenous cAMP signaling depends on AKAPs and the presence of multiple signaling pathways after the interaction between AKAPs and both PKAs.
In response to stress such as hypoxia, cytokines, and infection, HO-1 is induced to have an anti-oxidant and anti-inflammatory function. HO-1 regulates cellular contents with suppression of LPS-induced inflammation.13 To determine the effects of HO-1 on LPS-induced COX-2 expression in RAW 264.7 macrophages, we examined the effect of the HO inhibitor ZnPP (20 µM) or the HO activator CoPP (10 µM) at 6 or 24 h post-treatment. As shown in Fig. 2, enhancement of HO-1 expression by CoPP treatment suppressed LPS-induced COX-2 expression at both time points. In contrast, inhibition of HO-1 expression by the HO inhibitor ZnPP augmented LPS-induced COX-2 expression. Notably, COX-2 expression was not influenced by HO-1 expression level in the absence of LPS. This suggests that induction of HO-1 can inhibit the intracellular signaling events associated with cellular expression of COX-2 enzyme in response to LPS.
HO-1 is induced by TLR4 ligation.18 Because induction of HO-1 inhibits LPS-induced COX-2 expression, we hypothesized that PGE2-PKA-AKAP signaling could suppress LPS-induced HO-1 expression and cause positive feedback augmentation of COX-2 expression. As shown in Fig. 3, the HO-1 activator CoPP significantly reduced LPS-induced COX-2 expression, and exogenous PGE2 restored CoPP-inhibited responses to LPS at 6 h. Exogenous PGE2 attenuated LPS-induced HO-1 expression and modestly blocked the potentiating effects of exogenous CoPP on LPS-induced HO-1. This suggests that suppression of LPS-induced HO-1 expression by the addition of PGE2 is responsible for the amplification of COX-2 in response to ligation of TLR4. To delineate the role of endogenous PGE2-PKA-AKAP signaling on LPS-induced HO-1 expression, we challenged cells with LPS in the presence or absence of a PKA inhibitor (KT5720) or AKAP disruptors (Ht31 or RIAD). The PKA inhibitor enhanced LPS-induced HO-1 expression, and these potentiating effects were blocked by exogenous PGE2 (Fig. 4A). To determine whether AKAPs are involved in the PKA-dependent suppression of LPS-induced HO-1, we disrupted the potential PKA RI-AKAP interactions with RIAD peptide and RII-AKAP interactions with Ht31 peptide. Both AKAP disruptors augmented LPS-induced HO-1 expression to an extent similar to that observed with the PKA inhibitor KT5720 (Fig. 4B). To confirm that PKA is indeed responsible for blocking HO-1 expression, we measured bilirubin generated from HO activity. In the presence of KT5720, bilirubin increased markedly, and this effect was restored by exogenous PGE2 (Fig. 5). Taken together, these data clearly demonstrate that suppression of HO-1 expression via PGE2-PKA-AKAP signaling potentiates LPS-induced COX2 expression in RAW 264.7 macrophages.
TLR4 responses are mainly mediated via NF-κB activation.19,20 To evaluate whether induction of HO-1 involves LPS-induced NF-κB activation, we stimulated cells for 1 h with LPS, then observed NF-κB p65 expression in the nuclear fraction via Western blotting. As shown in Fig. 6A, NF-κB p65 proteins translocated to the nucleus only with LPS treatment, and PGE2 augmented the p65 activation via TLR4 ligation. In addition, the HO-1 activator CoPP suppressed NF-κB p65 expression, and exogenous PGE2 partially restored its effect. These findings were confirmed by assessing the nuclear translocation of NF-κB p65 subunit by immunofluorescence microscopy and by quantifying the nuclear colocalization of p65 with DAPI. LPS enhanced p65 nuclear translocation in macrophages; however, LPS-induced p65 translocation was attenuated with the HO-1 activator CoPP (Fig. 6B and C). Taken together, these results demonstrate that the induction of HO-1 has an inhibitory effect on LPS-induced COX-2 expression through the attenuation of LPS-induced p65 translocation.
This study elucidates the role of HO-1 in the positive feedback regulation of COX-2 expression by TLR4 stimulation and characterizes some of the molecular mechanisms involved in this phenomenon. Our results show that (1) endogenous and exogenous PGE2 enhance COX-2 expression in macrophages stimulated with the TLR4 ligand LPS; (2) PKA-AKAP signaling has an important role in PGE2 potentiation of LPS-induced COX-2 expression; (3) suppression of HO-1 expression by LPS-induced endogenous PKA-AKAP signaling is essential to augment COX-2 expression; (4) induction of HO-1 attenuates LPS-induced COX-2 expression through the inhibition of LPS-induced NF-κB p65 activation and translocation. Suppression of HO-1 by endogenous PKA signaling is involved in amplifying the expression of COX-2 induced by LPS. The proposed mechanism by which PGE2, cAMP, PKA, AKAP, and HO-1 interrelate in potentiating LPS-induced COX-2 expression is depicted in the model illustrated in Fig. 7.
In this study, we extended the exploration of the mechanisms by which PGE2 modulates LPS-induced COX-2 expression by focusing on its ability to modulate HO-1 expression and activity. A role for PGE2 in the positive feedback regulation of COX-2 expression has been suggested by studies documenting enhanced COX-2 expression by autonomous EP2-cAMP-PKA signaling in various cells, such as renal medullary cells,11 monocytes,10 and macrophages.9 However, there is a paucity of information about the involvement of various AKAPs.
To examine these issues, our first approach was to inhibit macrophages with a PKA inhibitor and AKAP disruptors and to assess their individual effects on COX-2 expression. Our results indicate that both PKA RI- and RII-AKAP disruptors inhibit LPS-induced COX-2 expression. This finding is of interest because the PKA RI- or RII-AKAP complex provides a means for spatial and substrate specificity of PKA signaling. RII-AKAP mediated the PGE2 suppression of LPS-induced tumor necrosis factor alpha (TNF-α) and macrophage inflammatory protein (MIP)-1α, whereas RI-AKAP provided PGE2 enhancement of LPS-induced granulocyte stimulating factor (G-CSF) in RAW 264.7 macrophages.21 Likewise, PGE2 potentiated LPS-induced nitric oxide via RI-interacting AKAPs.22 Taken together, our results suggest that various AKAPs may involve LPS-induced COX-2 expression through different signaling pathways. In addition, PKA RI and RII AKAP disruptors exhibited no additive effects on LPS-induced HO-1 expression (data not shown). This result could be explained by the likely convergence of signaling pathways downstream from PKA RI or RII and AKAP binding.
We investigated the specific role of HO-1 in the positive feedback loop of COX-2 expression induced by LPS. HO-1 was chosen because it represents a major inducible cellular and tissue defense protein against oxidative stress including LPS.12 In addition, induction of HO-1 generally inhibits COX-2 expression, and HO-1 involvement in LPS-induced COX-2 expression has been reported in various cells such as J774 macrophages,23 mouse brain endothelial cells,16 and human umbilical vein endothelial cells.24 In this study, HO-1 protein expression was observed at 6 h in macrophages after binding with TLR4 ligand. Furthermore, our results with the HO-1 inhibitor and activator confirmed that LPS-induced COX-2 expression was attenuated by HO-1 induction. However, the relative importance of HO-1 in positive signaling loops of COX-2 expression and the molecular mechanism evoked by PKA in these processes are unclear.
Previous reports have proposed that cAMP-PKA signaling is involved in HO-1 induction.25-27 In contrast, our data suggest that suppression of HO-1 by cAMP-PKA signaling is critical for the positive feedback loop of LPS-induced COX-2 expression because a magnifying effect on LPS-induced HO-1 expression was observed in the presence of a PKA inhibitor or AKAP disruptors. These observations are indicative of modulatory effects of cAMP-PKA on HO-1 induction that are context-dependent.
LPS activates NF-κB through TLR4 and leads to the expression of COX-2.28 In the present study, we verified that NF-κB expression and translocation were inhibited by HO-1 induction, suggesting that the effect of this small molecule precedes the inhibition of COX-2 expression in LPS-activated macrophages.
Our data show that PKA RI and RII AKAPs involve feedback enhancement of LPS-induced COX-2 expression. However, we did not identify the responsible AKAPs because little is known about AKAP-related COX-2 expression induced by LPS stimulation. Additional work is necessary to clarify this issue.
In conclusion, this study describes the important role that PGE2-PKA-AKAP signaling plays in enhancing COX-2 expression induced by LPS through positive feedback in RAW 264.7 macrophages. Furthermore, the attenuation of LPS-induced HO-1 expression achieved by PKA-AKAP signaling contributes to the subsequent augmentation of LPS-induced COX-2 expression through the magnification of LPS-induced NF-κB activation and nuclear translocation in RAW 264.7 macrophages. Whereas previous studies have established separately that PGE2 enhances COX-2 expression and that PGE2 inhibits LPS-induced HO-1 expression in macrophages, our study directly links these two phenomena for the first time.
Figures and Tables
Fig. 1
The cyclic AMP/protein kinase A/A-kinase anchoring protein (cAMP/PKA/AKAP) axis is responsible for prostaglandin E2 (PGE2)-potentiated lipopolysaccharide (LPS)-induced COX-2 expression in RAW 264.7 macrophages. (A) Cells were pretreated with the COX inhibitor indomethacin (Indo, 5 µM) for 30 min followed by PGE2 (1 µM) for 10 min and incubated with LPS (1 µg/mL) for another 24 h. (B) Cells were incubated with dibutyryl cAMP (1 µM) for 10 min followed by LPS for 24 h. (C) Cells were pretreated with PKA inhibitor KT5720 (1 µM) for 30 min followed by PGE2 for 10 min before incubation for 24 h with LPS. (D) Cells were pretreated for 20 min with the AKAP/PKA RII-specific disruptor peptide Ht31 (25 µM) or the AKAP/PKA RI-specific disruptor RIAD (25 µM) followed by LPS for 24 h. In all experiments, cell lysates (30 µg protein) were subjected to Western blot analysis of COX-2 and β-actin, upper. Results from one experiment of three are shown. Relative expression of COX-2 was determined by densitometric analysis of immunoblots from three different experiments, normalized for β-actin expression, and expressed as percent of LPS alone, lower. The data are the means±S.E. values of three independent experiments, each performed in duplicate. *P<0.05.
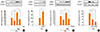
Fig. 2
Heme oxygenase-1 (HO-1) activation results in decreased LPS-induced COX-2 expression. Cells were pretreated with the HO activator copper protoporphyrin (CoPP, 10 µM) or the HO inhibitor zinc protoporphyrin (ZnPP, 20 µM) for 10 min followed by LPS (1 µg/mL) or vehicle for 6 h (left) and 24 h (right). Cell lysates at the indicated time points were subject to Western blot analysis of COX-2, HO-1, and β-actin. The blots are representative of three independently performed experiments.
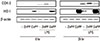
Fig. 3
Exogenous PGE2 suppresses LPS-induced HO-1 expression. Cells were pretreated with CoPP (10 µM) for 10 min followed by PGE2 (1 µM) for 10 min and then incubated with LPS (1 µg/mL) for 6 h. Lysates were subjected to Western blot analysis for COX-2, HO-1, and β-actin. Results from one experiment of three are shown. Relative expression levels of COX-2 and HO-1 were determined by densitometry analysis of immunoblots from three different experiments, normalized for β-actin expression, and expressed as percent of LPS alone. Data are the means±SEM of three independent experiments. *P<0.05.
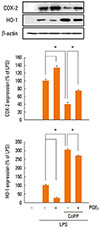
Fig. 4
PKA/AKAP interaction is required for PGE2-suppressed effects on LPS-induced HO-1 expression. (A) Cells were pretreated with the PKA inhibitor KT5720 (1 µM) for 30 min followed by PGE2 for 10 min before incubation for 6 h with LPS. (B) Cells were pretreated with Ht31 (25 µM) or RIAD (25 µM) for 20 min and then incubated with LPS (1 µg/mL) for 6 h. In all experiments, lysates were subjected to Western blot analysis for HO-1 and β-actin. Results from one experiment of three are shown (upper). Relative expression of COX-2 and HO-1 were determined by densitometry analysis of immunoblots from three different experiments, normalized for β-actin expression, and expressed as percent of LPS alone (lower). Data represent the means±SEM of three separate experiments. *P<0.05.
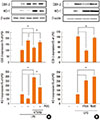
Fig. 5
Inhibition of PKA induces an increase in HO-1 activity. Cells were pretreated with the PKA inhibitor KT5720 (1 µM) for 20 min followed by PGE2 (1 µM) or vehicle for 10 min and then incubated with LPS for 6 h. Supernatants were harvested, and HO-1 activity was measured as described in the Materials and Methods. Data represent the means±SEM of three separate experiments. *P< 0.05.
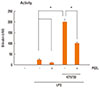
Fig. 6
Enhancement of HO-1 activity suppresses NF-κB signaling. (A) Cells were pretreated with vehicle control, or the HO activator CoPP (10 µM) for 10 min and then treated with PGE2 (1 µM) for 10 min before the addition of LPS (1 µg/mL). After 1 h, cells were harvested and the nuclear proteins were extracted as described in the Material and Methods. Immunoblotting of nuclear extracts was performed using anti-nuclear factor (NF)-κB p65. Results are representative of those from three experiments. *P<0.05. (B) Immunostaining for nuclear p65 localization in cells after 1 h exposure to: control, LPS only, LPS plus PGE2 (1 µM), LPS plus PGE2 plus CoPP, and LPS plus CoPP. Images depict nuclear translocation of NF-κB (arrows at green nuclei depict the translocation of NF-κB). (C) NF-κB immunofluorescence density determined by normalization with respect to translocation fluorescence. The nonfluorescent control cells were treated with second antibody only. Data are summarized and expressed as the means±SEM of three independent experiments. *P<0.05.
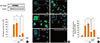
Fig. 7
Schematic representation of the proposed mechanism by which PGE2 signaling amplifies COX-2 expression through an autocrine manner in LPS-stimulated macrophages. Upon LPS binding to the toll-like receptor 4 (TLR4), the NF-κB signaling pathway becomes activated, leading to an increase in COX-2 expression, which in turn activates cAMP/PKA/AKAP through the production of PGE2. The PKA-AKAP complex blocks HO-1 activity, which results in enhanced translocation of NF-κB p65 to the nucleus.
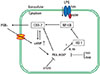
ACKNOWLEDGMENTS
We thank Dr. Marc Peters-Golden (University of Michigan Health Systems), and Carlos H. Serezani (Indiana University School of Medicine) for scientific input. Special thanks for Dr. You-Young Kim for thoughtful support. This research was supported by EMBRI Grants 2010-SN-04 from Eulji University.
References
1. Hwang D. Modulation of the expression of cyclooxygenase-2 by fatty acids mediated through toll-like receptor 4-derived signaling pathways. FASEB J. 2001; 15:2556–2564.
2. Harris RE. Cyclooxygenase-2 (cox-2) blockade in the chemoprevention of cancers of the colon, breast, prostate, and lung. Inflammopharmacology. 2009; 17:55–67.
3. Ren W, Hu L, Hua F, Jin J, Wang Y, Zhu L. Myeloid differentiation protein 2 silencing decreases LPS-induced cytokine production and TLR4/MyD88 pathway activity in alveolar macrophages. Immunol Lett. 2011; 141:94–101.
4. Vancheri C, Mastruzzo C, Sortino MA, Crimi N. The lung as a privileged site for the beneficial actions of PGE2. Trends Immunol. 2004; 25:40–46.
5. Serezani CH, Ballinger MN, Aronoff DM, Peters-Golden M. Cyclic AMP: master regulator of innate immune cell function. Am J Respir Cell Mol Biol. 2008; 39:127–132.
6. Jarnaess E, Taskén K. Spatiotemporal control of cAMP signalling processes by anchored signalling complexes. Biochem Soc Trans. 2007; 35:931–937.
7. Bos JL. Epac proteins: multi-purpose cAMP targets. Trends Biochem Sci. 2006; 31:680–686.
8. Hinz B, Brune K, Pahl A. Prostaglandin E(2) upregulates cyclooxygenase-2 expression in lipopolysaccharide-stimulated RAW 264.7 macrophages. Biochem Biophys Res Commun. 2000; 272:744–748.
9. Díaz-Muñoz MD, Osma-García IC, Fresno M, Iñiguez MA. Involvement of PGE2 and the cAMP signalling pathway in the upregulation of COX-2 and mPGES-1 expression in LPS-activated macrophages. Biochem J. 2012; 443:451–461.
10. Hinz B, Brune K, Pahl A. Cyclooxygenase-2 expression in lipopolysaccharide-stimulated human monocytes is modulated by cyclic AMP, prostaglandin E(2), and nonsteroidal anti-inflammatory drugs. Biochem Biophys Res Commun. 2000; 278:790–796.
11. Steinert D, Küper C, Bartels H, Beck FX, Neuhofer W. PGE2 potentiates tonicity-induced COX-2 expression in renal medullary cells in a positive feedback loop involving EP2-cAMP-PKA signaling. Am J Physiol Cell Physiol. 2009; 296:C75–C87.
12. Paine A, Eiz-Vesper B, Blasczyk R, Immenschuh S. Signaling to heme oxygenase-1 and its anti-inflammatory therapeutic potential. Biochem Pharmacol. 2010; 80:1895–1903.
13. Ryter SW, Alam J, Choi AM. Heme oxygenase-1/carbon monoxide: from basic science to therapeutic applications. Physiol Rev. 2006; 86:583–650.
14. Chin BY, Otterbein LE. Carbon monoxide is a poison... to microbes! CO as a bactericidal molecule. Curr Opin Pharmacol. 2009; 9:490–500.
15. Suh GY, Jin Y, Yi AK, Wang XM, Choi AM. CCAAT/enhancer-binding protein mediates carbon monoxide-induced suppression of cyclooxygenase-2. Am J Respir Cell Mol Biol. 2006; 35:220–226.
16. Shih RH, Yang CM. Induction of heme oxygenase-1 attenuates lipopolysaccharide-induced cyclooxygenase-2 expression in mouse brain endothelial cells. J Neuroinflammation. 2010; 7:86.
17. Leung PO, Wang SH, Lu SH, Chou WH, Shiau CY, Chou TC. Simvastatin inhibits pro-inflammatory mediators through induction of heme oxygenase-1 expression in lipopolysaccharide-stimulated RAW264.7 macrophages. Toxicol Lett. 2011; 207:159–166.
18. Waltz P, Carchman EH, Young AC, Rao J, Rosengart MR, Kaczorowski D, Zuckerbraun BS. Lipopolysaccaride induces autophagic signaling in macrophages via a TLR4, heme oxygenase-1 dependent pathway. Autophagy. 2011; 7:315–320.
19. Rhee SH, Hwang D. Murine TOLL-like receptor 4 confers lipopolysaccharide responsiveness as determined by activation of NF kappa B and expression of the inducible cyclooxygenase. J Biol Chem. 2000; 275:34035–34040.
20. Chhikara M, Wang S, Kern SJ, Ferreyra GA, Barb JJ, Munson PJ, Danner RL. Carbon monoxide blocks lipopolysaccharide-induced gene expression by interfering with proximal TLR4 to NF-kappaB signal transduction in human monocytes. PLoS One. 2009; 4:e8139.
21. Wall EA, Zavzavadjian JR, Chang MS, Randhawa B, Zhu X, Hsueh RC, Liu J, Driver A, Bao XR, Sternweis PC, Simon MI, Fraser ID. Suppression of LPS-induced TNF-alpha production in macrophages by cAMP is mediated by PKA-AKAP95-p105. Sci Signal. 2009; 2:ra28.
22. Kim SH, Serezani CH, Okunishi K, Zaslona Z, Aronoff DM, Peters-Golden M. Distinct protein kinase A anchoring proteins direct prostaglandin E2 modulation of Toll-like receptor signaling in alveolar macrophages. J Biol Chem. 2011; 286:8875–8883.
23. Olszanecki R, Kurnyta M, Biedroń R, Chorobik P, Bereta M, Marcinkiewicz J. The role of heme oxygenase-1 in down regulation of PGE2 production by taurine chloramine and taurine bromamine in J774.2 macrophages. Amino Acids. 2008; 35:359–364.
24. Liu XH, Pan LL, Yang HB, Gong QH, Zhu YZ. Leonurine attenuates lipopolysaccharide-induced inflammatory responses in human endothelial cells: involvement of reactive oxygen species and NF-kappaB pathways. Eur J Pharmacol. 2012; 680:108–114.
25. Immenschuh S, Kietzmann T, Hinke V, Wiederhold M, Katz N, Muller-Eberhard U. The rat heme oxygenase-1 gene is transcriptionally induced via the protein kinase A signaling pathway in rat hepatocyte cultures. Mol Pharmacol. 1998; 53:483–491.
26. Kim HJ, Tsoy I, Park MK, Lee YS, Lee JH, Seo HG, Chang KC. Iron released by sodium nitroprusside contributes to heme oxygenase-1 induction via the cAMP-protein kinase A-mitogen-activated protein kinase pathway in RAW 264.7 cells. Mol Pharmacol. 2006; 69:1633–1640.
27. Park MK, Kang YJ, Ha YM, Jeong JJ, Kim HJ, Seo HG, Lee JH, Chang KC. EP2 receptor activation by prostaglandin E2 leads to induction of HO-1 via PKA and PI3K pathways in C6 cells. Biochem Biophys Res Commun. 2009; 379:1043–1047.
28. Nakao S, Ogata Y, Shimizu-Sasaki E, Yamazaki M, Furuyama S, Sugiya H. Activation of NFkappaB is necessary for IL-1beta-induced cyclooxygenase-2 (COX-2) expression in human gingival fibroblasts. Mol Cell Biochem. 2000; 209:113–118.