Abstract
Akt (protein kinase B (PKB)) is a serine/threonine kinase that acts in the phosphatidylinositol 3-kinase (PI3K)/Akt signaling pathway. The PI3K/Akt signaling pathway, triggered by growth factors and hormones including vasopressin, is an important pathway that is widely involved in cellular mechanisms regulating transcription, translation, cell growth and death, cell proliferation, migration, and cell cycles. In particular, Akt and Akt substrate protein of 160 kDa (AS160) are likely to participate in the trafficking of aquaporin-2 (AQP2) in the kidney collecting duct. In this study, we demonstrated that 1) small interfering RNA (siRNA)-mediated gene silencing of Akt1 significantly decreased Akt1 and phospho-AS160 protein expression; and 2) confocal laser scanning microscopy of AQP2 in mouse cortical collecting duct cells (M-1 cells) revealed AS160 knockdown by siRNA increased AQP2 expression in the plasma membrane compared with controls, despite the absence of dDAVP stimulation. Thus, the results suggest that PI3K/Akt pathways could play a role in AQP2 trafficking via the AS160 protein.
PI3K activation by certain growth factors and hormones induces the conversion of lipid phosphatidylinositol-4,5-bisphosphate [PI(4,5)P2] to phosphatidylinositol-3,4,5-triphosphate [PI(3,4,5)P3]1). The PI(3,4,5)P3 product provides the direct binding sites to effector proteins having pleckstrin-homology (PH) domains that are accumulated at the region of PI3K activation. Akt (protein kinase B (PKB)) and phosphoinositide-dependent kinase 1 (PDK1) are well-known PI(3,4,5)P3-binding proteins and are the key mediators in the PI3K signaling pathway. Interaction between PI(3,4,5)P3 and the effector proteins stimulates phosphorylation of Akt which is catalyzed by autophosphorylation of PDK12). In turn, the phosphorylation of Akt catalyzes the kinase activity of Akt, resulting in the phosphorylation of downstream effector proteins.
The kinase activity of Akt is dependent on phosphorylation at T308 and S473. While T308 phosphorylation of Akt is catalyzed by PDK1, the phosphorylation of S473 in the hydrophobic motif of the C-terminal tail is mainly regulated by the mammalian target of rapamycin (mTOR)3).
Three isoforms of the mammalian Akt have been found, and the Akt (PKB) gene family consists of Akt1, Akt2, and Akt3. Although they are encoded from different genes, isoforms of Akt have a similar structural homology of over 80% that is composed of an N-terminal PH domain, a central kinase catalytic domain and a C-terminal extension containing a regulatory hydrophobic motif4). Both phosphorylation sites, threonine in a kinase catalytic domain and serine in regulatory motif, are conserved in T308 and S473 of Akt1, T309 and S474 of Akt2, and T305 and S472 of Akt3.
Despite their structural similarity, the three isoforms show different expressions and functions. Akt isoforms are widely expressed in normal tissues as well as in various tumor cells5). Akt1 is localized in the nucleus and the cytoplasm and translocated to the plasma membrane (PM) by stimulation. Akt2 is colocalized with the mitochondria, while Akt3 is localized in both the nucleus and nuclear membrane6).
Akt signaling is involved in the maintenance of metabolic homeostasis through regulation of the membrane protein trafficking as well as other intracellular protein trafficking. PI3K/Akt signaling is known to maintain the lipid homeostasis by regulating the translocation of surfactant protein A (SP-A) receptor p63 to the PM7). Moreover, Sharpe et al.8) reported that Akt regulates the early secretory pathway from ER to Golgi by phosphorylation of Sec24 protein involved in cargo selection. Furthermore, Robertson et al.9) demonstrated that acute insulin-stimulated Akt signaling decreases the surface expression of the norepinephrine transporter in the mouse hippocampus.
One of the well-known functions of Akt is to regulate glucose homeostasis. In Akt1-knockout mice, deletion of Akt1 inhibits the translocation of GLUT1 to the PM, and leads to reduced glucose uptake10). In muscle and fat cells, Akt and protein kinase C (PKC) are key signaling mediators of the PI3K pathway in the insulin-regulated transport of glucose into cells via glucose transporter GLUT411, 12). It was reported that AS160 (Akt substrate of 160 kDa, originally named TBC1D4 of the TBC family, Tre-2/Bub2/cdc16) is a downstream mediator of Akt signaling during the process of GLUT4 trafficking13). AS160 contains a Rab GTPase-activating protein (GAP) domain. It is proposed that Akt-induced phosphorylation of AS160 inhibits its GAP activity, leading to an increase in the active GTP-bound form of the AS160 target Rab proteins for vesicle trafficking14). Therefore, AS160, a downstream effecter protein of Akt signaling, could contribute to the regulation of Rab proteins associated with intracellular vesicle trafficking, possibly for aquaporin-2 (AQP2) in the kidney collecting ducts.
Rab proteins have been known to be a key mediator of regulated vesicle trafficking. Previous studies demonstrated that Rab proteins are associated with Akt signaling: (1) Both Rab 5 and Akt signaling are required to regulate insulin receptor-mediated endocytosis15, 16); (2) Rab 10 is involved Na+,K+-ATPase translocation to the PM by insulin-stimulated Akt activation17); (3) Rab 11-mediated Akt signaling promotes glucose uptake via GLUT1 trafficking18); (4) Rab 4 was identified as a specific Akt2 substrate in the modulating of GLUT4 vesicle trafficking19); (5) Rab 10 also mediated the regulatory signaling of GLUT4 trafficking dependent on the Akt pathway20-22); and (6) Rab 11 activation by Akt phosphorylation induced internalization and recycling of the insulin-like growth factor type-1 receptor and GLUT4 trafficking23-25).
AQP2 is the water channel protein expressed in the renal connecting tubule and collecting duct. Cell surface expression of AQP2 is regulated by arginine vasopressin (AVP)26, 27). Binding of vasopressin to vasopressin V2 receptor (V2R) in the basolateral PM stimulates adenylyl cyclase which converts ATP to cyclic AMP (cAMP). In turn, cAMP activates the protein kinase A (PKA) signaling pathway that is the major signaling pathway for AQP2 trafficking and up-regulation of AQP2 expression.
Recently, the Akt signaling pathway has been proposed to be another significant signaling pathway for regulation of AQP228). Insulin, one of the most potent hormones for Akt activation, stimulates the PI3K/Akt pathway via insulin receptor and increased AQP2 expression in primary cultures of the inner medullary collecting duct (IMCD) cells of the rat kidney. Moreover, a recent study suggested that AVP stimulates PI3K/Akt signaling pathways29), by demonstrating that dDAVP stimulates Akt phosphorylation in rat kidney IMCD tubule suspension.
Rab GTPases are important mediators for Akt-dependent intracellular vesicle trafficking. Transcripts of several Rab proteins were found in the IMCD of the rat kidney by transcriptional profiling analysis30). Morevoer, Rab proteins were identified in the AQP2-expressing vesicles from IMCD cells of the rat kidney by LC-MS/MS analysis31). Among Rab proteins identified by proteomic analysis, seven Rab proteins (Rab 4, 5, 7, 11, 18, 21 and 25), have been known to be involved in the trafficking of endosomes. In addition, Rab 5, Rab 7 and Rab 11 were detected in the AQP2-immunoisolated vesicles by immunogold staining for electron microscopy. Rab 4, Rab 5, Rab 7 and Rab 11 were also found in the AQP2-expressing vesicles by immunoblotting31). Importantly, Rab 2, Rab 10 and Rab 14 are the AQP2 vesicle-associated Rab proteins and are also reported as target substrates of AS160 in insulin-stimulated Akt singaling14).
Indeed, we examined whether AS160, a novel Akt substrate, plays a role in the regulation of AQP2 trafficking in the kidney collecting duct cells. The main strategy was to examine whether AS160 knockdown in mouse cortical collecting duct cells (M-1 cells) by small interfering RNA (siRNA)-mediated gene silencing could affect the AQP2 trafficking to the PM. The results were summarized below.
Immunofluorescence microscopy demonstrated that short-term dDAVP treatment (10-8 M, 15 minutes) increased AQP2 labeling intensity in the PM of M-1 cells (arrows in Fig. 1B), compared with vehicle-treated M-1 cells (Fig. 1A). Moreover, AQP2 expression (both deglycosylated ~28 kDa and glycosylated ~35-50 kDa bands) was significantly increased in response to 2 day-dDAVP (10-8 M) treatment (130 ± 4% of vehicle-treated control levels, P < 0.05), compared with either vehicle (100 ± 7%) or 1 day-dDAVP (10-8 M) treatment (107 ± 4%, Fig. 1C and 1D). This indicates that AQP2 is endogenously expressed in M-1 cells, a mouse cortical collecting duct cell line, and its expression is regulated by both short-term and long-term treatment of dDAVP.
Akt1-directed siRNA significantly decreased Akt1 protein expression (27 ± 1% of control levels, non-targeting siRNA-transfected M-1 cells, P < 0.05, Fig. 2A and 2B). Importantly, AQP2 protein expression was unchanged (116 ± 2%, not significant) in response to Akt1 knockdown (Fig. 2C and 2D). In addition, M-1 cells with Akt1 knockdown for 48 hours demonstrated no changes in morphology and cell number compared with control M-1 cells transfected with non-targeting siRNA (not shown).
One more set of Akt1 knockdown [Akt1 expression: 12 ± 2% of control levels (non-targeting siRNA-transfected M-1 cells), P < 0.05, data not shown)] was made. Akt1 knockdown was associated with unchanged or marginally increased total AS160 expression (vehicle-treated group: 128 ± 7%, P = 0.054 and short-term dDAVP-treated group, 10-8 M, 10 minutes, 138 ± 9%, P < 0.05, Fig. 3A and 3B). Importantly, the expression of phospho-AS160 was unchanged indicated by an arrow in Fig. 3A, and hence, the phospho-AS160 values normalized to the total AS160 protein values were markedly decreased (Fig. 3C). For phosphoproteins, the phosphoprotein values were normalized to the total protein values (i.e., log2 [(Fep/Fcp)/(Fet/Fct)], where F = band intensity, e = experiment, c = control, p = phosphoprotein, and t = total protein, as previously demonstrated)29). The number of replicates (n) in the figures indicates the number of separate cell lysates examined. This indicates that AS160 expression and phosphorylation of AS160 are dependent on Akt.
As shown in Fig. 4, AQP2-labeled M-1 cells were randomly selected in each group (asterisk in Fig. 4A). The total pixel intensity of the PM (Fig. 4C) and the whole cell (both PM and cytosol except nucleus, Fig. 4D) were acquired, respectively. The ratio of total pixel intensity in the PM to that of the whole cell (ratio of PM/Total, Fig. 4G) from the randomly selected cells in each group was acquired. The ratio is a measure of surface AQP2 normalized for the whole cell AQP2 expression, and the changes of this ratio would demonstrate the changes of the surface-to-total AQP2 distribution. Laser scanning confocal microscopic examination of AQP2 and quantitative analysis of AQP2 immunolabeling density (i.e., changes of the surface-to-total AQP2 distribution in the M-1 cells) demonstrated that AQP2 expression in the PM in the vehicle-treated basal condition was significantly higher in the M-1 cells with AS160 knockdown (123 ± 2%, P < 0.05, number of examined cells = 52, Fig. 4F and 4G) compared with the control M-1 cells transfected with non-targeting siRNA (number of examined cells = 53, Fig. 4E and 4G). This indicates that AS160 plays a role as a negative regulator for exocytosis of AQP2.
Taken together, (1) siRNA-mediated gene silencing of Akt1 significantly decreased Akt1 and phospho-AS160 protein expression; and (2) confocal laser scanning microscopy of AQP2 in M-1 cells revealed that AS160 knockdown by siRNA increased AQP2 expression in the PM compared with control, despite the absence of dDAVP stimulation. This finding is compatible with a previous study showing increased GLUT4 trafficking in adipocytes with AS160 knockdown despite the absence of insulin stimulation. Thus, the results suggest that PI3K/Akt pathways could play a role in AQP2 trafficking via the AS160.
Acknowledgement
This study was supported by the Korean Society of Electrolyte Metabolism (2009) and a grant of the Korea Healthcare Technology R & D Project, Ministry of Health & Welfare, Korea (A100617).
References
2. Casamayor A, Morrice NA, Alessi DR. Phosphorylation of Ser-241 is essential for the activity of 3-phosphoinositide-dependent protein kinase-1: identification of five sites of phosphorylation in vivo. Biochem J. 1999; 342:287–292. PMID: 10455013.


3. Sarbassov DD, Guertin DA, Ali SM, Sabatini DM. Phosphorylation and regulation of Akt/PKB by the rictor-mTOR complex. Science. 2005; 307:1098–1101. PMID: 15718470.


4. Kumar CC, Madison V. AKT crystal structure and AKT-specific inhibitors. Oncogene. 2005; 24:7493–7501. PMID: 16288296.


5. Zinda MJ, Johnson MA, Paul JD, et al. AKT-1, -2, and -3 are expressed in both normal and tumor tissues of the lung, breast, prostate, and colon. Clin Cancer Res. 2001; 7:2475–2479. PMID: 11489829.
6. Santi SA, Lee H. The Akt isoforms are present at distinct subcellular locations. Am J Physiol Cell Physiol. 2010; 298:C580–C591. PMID: 20018949.


7. Kazi AS, Tao JQ, Feinstein SI, Zhang L, Fisher AB, Bates SR. Role of the PI3-kinase signaling pathway in trafficking of the surfactant protein A receptor P63 (CKAP4) on type II pneumocytes. Am J Physiol Lung Cell Mol Physiol. 2010; 299:L794–L807. PMID: 20870746.


8. Sharpe LJ, Luu W, Brown AJ. Akt phosphorylates Sec24: new clues into the regulation of ER-to-Golgi trafficking. Traffic. 2011; 12:19–27. PMID: 20950345.


9. Robertson SD, Matthies HJ, Owens WA, et al. Insulin reveals Akt signaling as a novel regulator of norepinephrine transporter trafficking and norepinephrine homeostasis. J Neurosci. 2010; 30:11305–11316. PMID: 20739551.


10. Boxer RB, Stairs DB, Dugan KD, et al. Isoform-specific requirement for Akt1 in the developmental regulation of cellular metabolism during lactation. Cell Metab. 2006; 4:475–490. PMID: 17141631.


11. Czech MP, Corvera S. Signaling mechanisms that regulate glucose transport. J Biol Chem. 1999; 274:1865–1868. PMID: 9890935.


12. Khan AH, Pessin JE. Insulin regulation of glucose uptake: a complex interplay of intracellular signalling pathways. Diabetologia. 2002; 45:1475–1483. PMID: 12436329.


13. Eguez L, Lee A, Chavez JA, et al. Full intracellular retention of GLUT4 requires AS160 Rab GTPase activating protein. Cell Metab. 2005; 2:263–272. PMID: 16213228.


14. Miinea CP, Sano H, Kane S, et al. AS160, the Akt substrate regulating GLUT4 translocation, has a functional Rab GTPase-activating protein domain. Biochem J. 2005; 391:87–93. PMID: 15971998.
15. Su X, Lodhi IJ, Saltiel AR, Stahl PD. Insulin-stimulated Interaction between insulin receptor substrate 1 and p85alpha and activation of protein kinase B/Akt require Rab5. J Biol Chem. 2006; 281:27982–27990. PMID: 16880210.
16. Barbieri MA, Kohn AD, Roth RA, Stahl PD. Protein kinase B/akt and rab5 mediate Ras activation of endocytosis. J Biol Chem. 1998; 273:19367–19370. PMID: 9677351.


17. Comellas AP, Kelly AM, Trejo HE, et al. Insulin regulates alveolar epithelial function by inducing Na+/K+-ATPase translocation to the plasma membrane in a process mediated by the action of Akt. J Cell Sci. 2010; 123:1343–1351. PMID: 20332111.


18. Wieman HL, Wofford JA, Rathmell JC. Cytokine stimulation promotes glucose uptake via phosphatidylinositol-3 kinase/Akt regulation of Glut1 activity and trafficking. Mol Biol Cell. 2007; 18:1437–1446. PMID: 17301289.


19. Yoshizaki T, Imamura T, Babendure JL, Lu JC, Sonoda N, Olefsky JM. Myosin 5a is an insulin-stimulated Akt2 (protein kinase Bbeta) substrate modulating GLUT4 vesicle translocation. Mol Cell Biol. 2007; 27:5172–5183. PMID: 17515613.
20. Sano H, Roach WG, Peck GR, Fukuda M, Lienhard GE. Rab10 in insulin-stimulated GLUT4 translocation. Biochem J. 2008; 411:89–95. PMID: 18076383.


21. Sano H, Eguez L, Teruel MN, et al. Rab10, a target of the AS160 Rab GAP, is required for insulin-stimulated translocation of GLUT4 to the adipocyte plasma membrane. Cell Metab. 2007; 5:293–303. PMID: 17403373.


22. Larance M, Ramm G, Stockli J, et al. Characterization of the role of the Rab GTPase-activating protein AS160 in insulin-regulated GLUT4 trafficking. J Biol Chem. 2005; 280:37803–37813. PMID: 16154996.


23. Romanelli RJ, LeBeau AP, Fulmer CG, Lazzarino DA, Hochberg A, Wood TL. Insulin-like growth factor type-I receptor internalization and recycling mediate the sustained phosphorylation of Akt. J Biol Chem. 2007; 282:22513–22524. PMID: 17545147.


24. Uhlig M, Passlack W, Eckel J. Functional role of Rab11 in GLUT4 trafficking in cardiomyocytes. Mol Cell Endocrinol. 2005; 235:1–9. PMID: 15866422.


25. Kessler A, Tomas E, Immler D, Meyer HE, Zorzano A, Eckel J. Rab11 is associated with GLUT4-containing vesicles and redistributes in response to insulin. Diabetologia. 2000; 43:1518–1527. PMID: 11151761.


26. Kwon TH, Nielsen J, Moller HB, Fenton RA, Nielsen S, Frokiaer J. Aquaporins in the kidney. Handb Exp Pharmacol. 2009; 190:95–132. PMID: 19096774.


27. Lee YJ, Song IK, Jang KJ, et al. Increased AQP2 targeting in primary cultured IMCD cells in response to angiotensin II through AT1 receptor. Am J Physiol Renal Physiol. 2007; 292:F340–F350. PMID: 16896188.
28. Bustamante M, Hasler U, Kotova O, et al. Insulin potentiates AVP-induced AQP2 expression in cultured renal collecting duct principal cells. Am J Physiol Renal Physiol. 2005; 288:F334–F344. PMID: 15494547.


29. Pisitkun T, Jacob V, Schleicher SM, Chou CL, Yu MJ, Knepper MA. Akt and ERK1/2 pathways are components of the vasopressin signaling network in rat native IMCD. Am J Physiol Renal Physiol. 2008; 295:F1030–F1043. PMID: 18667481.


30. Uawithya P, Pisitkun T, Ruttenberg BE, Knepper MA. Transcriptional profiling of native inner medullary collecting duct cells from rat kidney. Physiol Genomics. 2008; 32:229–253. PMID: 17956998.


31. Barile M, Pisitkun T, Yu MJ, et al. Large scale protein identification in intracellular aquaporin-2 vesicles from renal inner medullary collecting duct. Mol Cell Proteomics. 2005; 4:1095–1106. PMID: 15905145.


Fig. 1
Regulation of aquaporin-2 (AQP2) in response to dDAVP treatment in mouse cortical collecting duct cells (M-1 cells). A and B) Immunofluorescence microscopy of AQP2. Short-term dDAVP-treatment (10-8 M) for 15 minutes in M-1 cells increased AQP2 trafficking to the plasma membrane (indicated by arrows in B), compared with vehicle-treated M-1 cells (A). C and D) Semiquantitative immunoblotting of AQP2. Immunoblots reacted with anti-AQP2 revealed 29- and 35- to 50-kDa AQP2 bands, representing nonglycosylated and glycosylated forms of AQP2, respectively. AQP2 expression was significantly increased in 2 day-dDAVP-treated M-1 cells, compared with either vehicle-treated or 1 day-dDAVP-treated M-1 cells. n, the number of separate M-1 cell lysates.
*P < 0.05 when compared to the vehicle-treated control group.
†P < 0.05 when compared to the 1 day-dDAVP treated group.
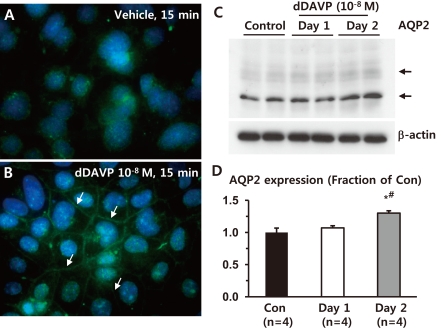
Fig. 2
Semiquantitative immunoblotting of Akt1 and AQP2, aquaporin-2 (AQP2) in mouse cortical collecting duct cells (M-1 cells) with Akt1 knockdown. A and B) Immunoblots reacted with anti-Akt1 revealed 60-kDa Akt1 bands. Akt1-directed small interfering RNA (siRNA) treatment decreased Akt1 expression. C and D) Immunoblots reacted with anti-AQP2 revealed 29- and 35- to 50-kDa AQP2 bands, representing nonglycosylated and glycosylated forms of AQP2, respectively. AQP2 expression was unchanged in M-1 cells with Akt1 knockdown. n, the number of separate M-1 cell lysates.
*P < 0.05 when compared to control non-targeting siRNA-transfected M-1 cells.
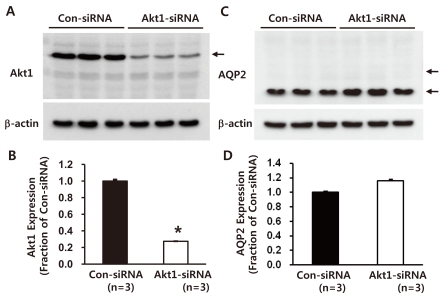
Fig. 3
Semiquantitative immunoblotting of Akt1, AS160, and p-AS160 in mouse cortical collecting duct cells (M-1 cells) with Akt1 knockdown. A-C) Akt1 knockdown was associated with unchanged or marginally increased total AS160 expression. In contrast, phospho-AS160 level normalized to the total AS160 protein values were markedly decreased (~160 kDa band on PAS immunoblots indicated by an arrow in panel A). siRNA, small interfering RNA; n, the number of separate M-1 cell lysates.
*P < 0.05 when compared with the vehicle-treated control group (non-targeting siRNA-transfected M-1 cells).
†P < 0.05 when compared with dDAVP-treated control group (non-targeting siRNA-transfected M-1 cells).
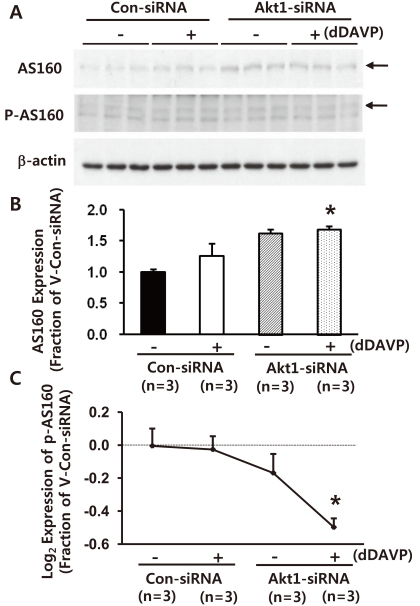
Fig. 4
Semiquantitative immunocytochemistry of aquaporin-2 (AQP2). AQP2-labeled mouse cortical collecting duct cells (M-1 cells) were randomly selected (asterisk in A) and total pixel intensity of the plasma membrane (PM) (C) and whole cell (both PM and cytosol except nucleus, D) were acquired respectively from the selected cells (B). E and F) AQP2 labeling in M-1 cells transfected with Con-small interfering RNA (Con-siRNA) or AS160-siRNA. G) The ratio of total pixel intensity in the PM to that of the whole cell (ratio of PM/Total) was calculated from randomly selected M-1 cells and averaged in each group. N, nucleus; n = number of cells randomly chosen and examined in each group.
*P < 0.05 when compared to Con-siRNA. Bar, 10 µm.
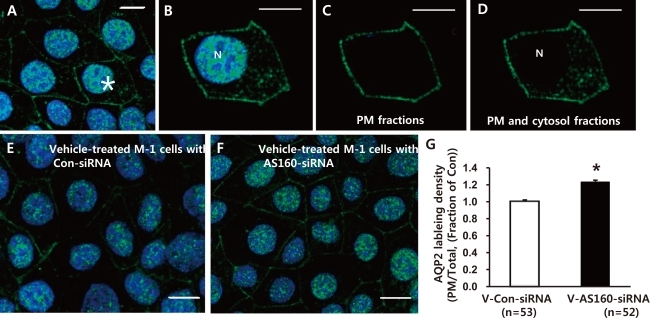