Abstract
Background
The purpose of this study was to determine the tibial fixation strength provided by different intraosseous soft tissue graft lengths within the tibial tunnel.
Methods
Porcine tibial bones and digital flexor tendons were used for testing. Bone mineral densities of proximal tibial medial condyles were measured, and two-strand tendon bundles of 8 mm diameter were used. An intraosseous graft length of 2 cm was used in group 1 (n = 10), and a graft length of 4 cm was used in group 2 (n = 10). Tunnels were 4 cm in length and 8 mm in diameter. Tibial fixation was performed using a suture tied around a screw post with a washer and an additionally inserted 7 × 20 mm bioabsorbable screw. After applying preconditioning loading of 10 cycles, 1,000 cycles between 70-220 N were applied at a frequency of 1 Hz. Graft slippage and total graft movement were recorded. Ultimate tensile strength was measured by pull-out testing at an Instron crosshead speed of 1,000 mm/min.
Results
No significant intergroup difference was found for total graft movement after cyclic loading (slippage in group 1, 1.2 mm and group 2, 1.2 mm, respectively, p = 0.917; and total graft movement in group 1, 3.3 mm and group 2, 2.7 mm, respectively, p = 0.199). However, mean ultimate tensile strength in group 2 was significantly higher than that in group 1 (group 1, 649.9 N; group 2, 938 N; p = 0.008).
Conclusions
In a porcine model, ultimate tensile strength was greater for a 4 cm long intraosseous flexor tendon in the tibial tunnel. However, no intergroup difference in graft slippage or total graft movement was observed. The results show that a 2 cm intraosseous graft length in the tibial tunnel is safe and has sufficient strength (> 450 N) for adequate rehabilitation after anterior cruciate ligament reconstruction.
Many options are available for anterior cruciate ligament (ACL) reconstruction such as an autologous bone-patellar tendon-bone graft, a hamstring tendon graft, or an allograft. The hamstring tendon is being increasingly used for ACL reconstruction,1) despite delayed healing of the bone-tendon interface.2) Soft tissue grafts, such as hamstring tendon grafts, for ACL reconstruction need a long intraosseous length within the bone tunnel for adequate fixation strength due to delayed healing compared with a bone-to-bone graft. We considered if the strengths of shorter tendon lengths within the bone tunnel are similar to those of the lengths currently used, ACL reconstruction could be performed using a single rather than a double tendon (semitendinosus and gracilis tendon), and thus, reduce donor site morbidity and allow earlier rehabilitation.
The ACL carries only small loads during normal daily functions, that is, to 20% (450-500 N) of its load-bearing capacity at failure.3,4) In fact, the most strenuous activities rarely expose the ACL to more than 50% of its maximal load.5) Furthermore, it has been previously established that fixation strength of 450 N is sufficient for rehabilitation after ACL reconstruction.6,7)
If a minimum of 28 cm of semitendinosus tendon is harvested, a 7 cm four-strand semitendinosus graft can be made for ACL reconstruction, a 2 cm graft for the femoral tunnel, a 3 cm graft for the intra-articular portion, and a 2 cm graft for the tibial tunnel. Some studies have considered optimal graft length in the femoral bone tunnel. Zantop et al.,8) Yamazaki et al.,9) and Qi et al.10) found that reducing tendon graft length in the femoral tunnel from 25 to 15 mm did not have any adverse histological or biomechanical effects in a small animal model, and a previous article reported that a semitendinosus tendon graft of > 28 cm is harvested for a four-stranded single graft in 94.5% of cases during ACL reconstruction surgery.11)
We hypothesized that fixation with a 2 cm tendon length in a bone tunnel would achieve the fixation strength of 450 N required for adequate rehabilitation after ACL reconstruction. Accordingly, we measured the tibial fixation strengths provided by soft tissue grafts of different lengths within tibial tunnels using porcine tibia, and measured the fixation strengths of 2 cm grafts within the bone tunnels.
This study was approved by Kyungpook National University Hospital's Institutional Review Board, which has been certified by the Forum for Ethical Review Committees in the Asian and Western Pacific Region. This study was performed using 20 freshly frozen porcine hind limbs (10 pairs) from 90 kg pigs aged 8-9 months. After removing all muscles, ligaments, and knee joint capsules, tibiae were prepared for testing. Hind limb flexor digitorum tendons were then harvested, and all specimens were stored at -20℃ until required for testing. Before testing, each specimen was thawed overnight at 4℃. Hind-limbs were then allocated to one of the two study groups (group 1 [n = 10], the 2 cm intraosseous graft length group; and group 2 [n = 10], the 4 cm graft length group). Left and right tibiae were allocated to groups 1 and 2, respectively.
Bone mineral densities (BMD) were measured by dual-emission X-ray absorptiometry (DEXA; Lunar Prodigy, GE HealthCare, Madison, WI, USA) at the anteromedial tibial metaphysis, which was the planned tunnel location. In addition, the BMDs of the anteromedial tibial metaphyses of proximal tibiae were measured in 10 healthy young men (mean age, 27.9 ± 2.6 years; mean body mass index, 24.5 ± 2.1 kg/m2). For all porcine tibiae, a tunnel of diameter 8 mm and length of 4 cm was prepared on the anteromedial tibia, and a guide pin was located at the center of the ACL. Graft tendons of length 8 and 6 cm were prepared. After making a double loop by folding grafts once, graft diameters were 8 mm. Graft ends were then sewn using Ticron #2 (Tyco, Waltham, MA, USA) non-absorbable sutures with an interlocking stitch (Fig. 1). In group 1 (n = 10), the grafts had an intraosseous length of 2 cm and an extraosseous length of 4 cm, whereas in group 2 (n = 10) grafts had an intraosseous length of 4 cm and an extraosseous length of graft of 4 cm. Grafts were fixed using four-strand sutures tied around a screw post with a washer. In addition, a single 7 × 20 mm bioabsorbable screw (Bioscrew, ConMed Linvtec, Largo, FL, USA) was inserted between the tibial bone tunnels and grafts (Fig. 2).12,13)
An Electroplus E3000 unit (Instron, High Wycombe, UK) was used for tensile strength testing. Briefly, a tibia was positioned beneath the load cell with the bone tunnel parallel to the axis of the test machine, and the graft loop was then secured over a shackle bolt (rafts were kept moist with a saline spray during preparation and testing) (Fig. 3). Samples were subjected to preconditioning, which involved 10 loading cycles alternating between 0 and 50 N. The applied load was then increased to 145 N and samples were subjected to 1,000 cycles of 70-220 N at 1 Hz (this protocol is consistent with the ACL loads during normal walking, as determined by Coleridge and Amis14)). Grafts were marked with ink at tunnel exit points after applying the preconditioning load, and again after cycle tensile loading. Graft slippage was defined as the distance between these two lines, and was measured using an electronic caliper.15) Total graft movement was defined as the change in maximum crosshead movement relative to the extension at the first load peak, and represented a combination of graft slippage relative to the tibia plus creep elongation. Ultimate tensile strength was measured by pull-out testing at a crosshead speed of 1,000 mm/min after completing the cyclic tensile loading test; pull-out testing ended when a graft pulled out of the bone.15) Failure modes were evaluated, and the means, ranges, and standard deviations of graft slippage, total graft movement, and ultimate tensile strength; 95% confidence limits were calculated.
The Mann-Whitney U-test was used to compare graft slippage, total graft movement, and ultimate tensile strength, and the Kruskal-Wallis test was used to compare BMD differences. The required study sample size was determined using biomechanical data from earlier studies.16,17,18) Results were analyzed using IBM SPSS ver. 19 (IBM Co., Armonk, NY, USA), and p-value < 0.05 was considered significant.
Mean BMD at the anteromedial tibial condyle was 1.119 g/cm2 (range, 0.968 to 1.344 g/cm2) in group 1, 1.025 g/cm2 (range, 0.854 to 1.298 g/cm2) in group 2, and 0.974 g/cm2 (range, 0.774 to 1.214 g/cm2) in the human control group (p = 0.059 and 0.356). The BMD differences were not significant. All grafts pulled-out from the bone tunnel, except one in group 2 that failed due to graft rupture.
Table 1 summarizes graft slippage, total graft movement, and ultimate tensile strength in the two experimental groups. After cyclic loading, mean graft slippage was 1.2 mm in group 1 and 1.2 mm in group 2 (p = 0.917), and the upper 95% confidence limit of graft slippage was < 2.0 mm in both groups. Mean total graft movement after cyclic loading was 3.3 mm in group 1 and 2.7 mm in group 2 (p = 0.199), and the upper 95% confidence limit of total graft movement was 4.1 mm in group 1 and 3.1 mm in group 2 (Table 1 and Fig. 4). No significant intergroup difference was observed for graft slippage or total graft movement.
Mean ultimate tensile strength was significantly lower in group 1 than that in group 2 (649.9 N and 938 N, respectively; p = 0.008) (Table 1 and Fig. 5), and the mean lower 95% confidence limits in the two groups were 494.4 N and 783.3 N, respectively. Nevertheless, fixation strength of the 2 cm graft (> 450 N) was suitable for early rehabilitation after ACL reconstruction.
This study was undertaken to determine whether a 2 cm long graft within a bone tunnel has sufficient strength for early rehabilitation after ACL reconstruction, and whether a single hamstring tendon, the use of which would reduce donor site morbidity after harvesting semitendinosus and gracilis tendon, is suitable for ACL reconstruction. Mean ultimate tensile loads in groups 1 and 2 for a four-strand suture tied around a screw post with a washer and secured with additional a bioabsorbable interference screw were 649.9 N and 938 N, respectively, which were greater than ultimate tensile loads reported in previous studies.12,14,15,19) Our findings indicate the ultimate tensile loads of 2 cm grafts meet early rehabilitation requirements, despite being lower than those provided by a 4 cm graft.
Early rehabilitation after ACL reconstruction is associated with better outcomes. However, previous fixation strength studies after ACL reconstruction have usually focused on fixation methods and graft materials or on the biomechanical properties of interference screw fixed soft-tissue grafts,12,14,15,19) and relatively few have addressed tendon lengths within bone tunnels.8,9,10,20) In these biomechanical studies, mean reported ultimate failure loads for metal screws ranged from 338 N to 521 N. Moreover, it has been separately reported that the ACL graft fixation complex is subjected to loads of approximately 450 N during early functional rehabilitation programs.6,7)
Insufficient scientific evidence is available to make recommendations regarding graft length in the femoral tunnel during ACL reconstruction using a soft tissue graft, but it is commonly believed that graft healing is improved by having more graft tissue within the bone tunnel, as this provides a tighter fit.8) Greis et al.,20) in an extra-articular dog model, reported that pull-out graft strength at 6 weeks after surgery was enhanced by increasing tendon length within the tunnel. However, Yamazaki et al.,9) who compared 5 mm and 15 mm tibial intraosseous grafts in an intra-articular dog model, found no differences between the histological and biomechanical properties of healing grafts at 6 weeks postoperatively. In contrast, the placement of an excessively long flexor graft within the bone tunnel does not result in an additional increase in anchoring strength or graft stiffness after ACL reconstruction. Zantop et al.8) reported on intra-articular bone tunnel tendon healing in a goat model, and found no negative correlation between graft length in the femoral tunnel and resulting knee kinematics or structural properties. Furthermore, reducing tendon graft length in femoral bone tunnels from 25 mm to 15 mm did not have an adverse effect. Qi et al.10) performed an intra-articular study on bone tunnel tendon healing in a dog model and concluded that the histological maturity and biomechanical strength of the tendon bone junction after ACL reconstruction is delayed during the early stage if graft length in the bone tunnel is < 15 mm. However, it should be added that all of the above-mentioned animal studies were performed using small animals.8,9,10,20)
Some biomechanical and clinical studies have been conducted on ACL reconstruction using a single semitendinosus tendon, that is, without gracilis harvesting.11,21,22,23,24) The majority of these studies concluded that ACL reconstruction using a single four-strand semitendinosus tendon short graft produces good clinical results, does not compromise joint stability, and reduces donor site morbidity. They concluded that this technique provide a good option for ACL reconstruction.7,21,23)
Some limitations of the current study should be considered. First, we used a porcine model, which raises the question as to whether our results are clinically applicable. Furthermore, the specimen type used is important when performing graft fixation experiments using animal models. In a previous study on the use of interference screw graft fixation in porcine and human bone, the maximum tensile strength differences were insignificant.25) Direct comparisons between human specimens are difficult because it is difficult to control for factors such as, donor age and bone density differences. For these reasons, we chose to use porcine bone, which allowed us to control these factors. Furthermore, porcine tibiae have been used many times in similar experimental studies,13,18,19) and we found no significant difference between porcine and human BMDs. We chose not to use bovine tendons because they were more difficult to obtain, despite a report that they more closely match young human tendons.26) Second, this study involved time-zero biomechanical testing under immediate postoperative conditions; thus, no histological comparison was possible. However, to address the effects in humans, we performed tensile testing after 1,000 cyclic loadings, which ostensibly represents the activities of daily living.14,27) Third, we did not repeat measurement of the graft slippage to reduce error and did not perform intra- or interobserver reliability testing.
Our results show that ultimate tensile strength was greater for a 4 cm (938 N) long flexor tendon than a 2 cm (650 N) flexor tendon in the tibial tunnel in a porcine model,. However, these two flexor tendon lengths were not significantly different in graft slippage, total graft movement, or mode of failure. We conclude that a 2 cm graft is suitable for an early rehabilitation program after ACL reconstruction as it can cope with loads in excess of 450 N.
Figures and Tables
Fig. 2
Grafts in tibial tunnels. Drawing of a 2-cm long graft (A) and a 4-cm long graft (B) in intraosseous tunnels. *Indicates a bioabsorbable interference screw. (C) Tendon graft was fixed at the tibial tunnel by a post-tie suture and a bioabsorbable screw.
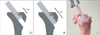
References
1. Pinczewski L, Roe J, Salmon L. Why autologous hamstring tendon reconstruction should now be considered the gold standard for anterior cruciate ligament reconstruction in athletes. Br J Sports Med. 2009; 43(5):325–327.
2. Rodeo SA, Arnoczky SP, Torzilli PA, Hidaka C, Warren RF. Tendon-healing in a bone tunnel: a biomechanical and histological study in the dog. J Bone Joint Surg Am. 1993; 75(12):1795–1803.
3. Noyes FR, Butler DL, Paulos LE, Grood ES. Intra-articular cruciate reconstruction. I: Perspectives on graft strength, vascularization, and immediate motion after replacement. Clin Orthop Relat Res. 1983; (172):71–77.
4. Noyes FR, Grood ES. The strength of the anterior cruciate ligament in humans and Rhesus monkeys. J Bone Joint Surg Am. 1976; 58(8):1074–1082.
5. Noyes FR, Keller CS, Grood ES, Butler DL. Advances in the understanding of knee ligament injury, repair, and rehabilitation. Med Sci Sports Exerc. 1984; 16(5):427–443.
6. Salmon L, Pinczewski L, Roe J. The science of rehabilitation of the ACL injured and reconstructed knee. J Sci Med Sport. 2009; 12:Suppl. S59–S60.
7. Shelbourne KD, Klotz C. What I have learned about the ACL: utilizing a progressive rehabilitation scheme to achieve total knee symmetry after anterior cruciate ligament reconstruction. J Orthop Sci. 2006; 11(3):318–325.
8. Zantop T, Ferretti M, Bell KM, Brucker PU, Gilbertson L, Fu FH. Effect of tunnel-graft length on the biomechanics of anterior cruciate ligament-reconstructed knees: intra-articular study in a goat model. Am J Sports Med. 2008; 36(11):2158–2166.
9. Yamazaki S, Yasuda K, Tomita F, Minami A, Tohyama H. The effect of intraosseous graft length on tendon-bone healing in anterior cruciate ligament reconstruction using flexor tendon. Knee Surg Sports Traumatol Arthrosc. 2006; 14(11):1086–1093.
10. Qi L, Chang C, Jian L, Xin T, Gang Z. Effect of varying the length of soft-tissue grafts in the tibial tunnel in a canine anterior cruciate ligament reconstruction model. Arthroscopy. 2011; 27(6):825–833.
11. Kyung HS, Kim TG, Oh CW, Yoon SH. Anterior cruciate ligament reconstruction with a four-strand single semitendinosus tendon autograft. J Korean Arthrosc Soc. 2009; 13(2):138–142.
12. Herrera A, Martinez F, Iglesias D, Cegonino J, Ibarz E, Gracia L. Fixation strength of biocomposite wedge interference screw in ACL reconstruction: effect of screw length and tunnel/screw ratio: a controlled laboratory study. BMC Musculoskelet Disord. 2010; 11:139.
13. Nagarkatti DG, McKeon BP, Donahue BS, Fulkerson JP. Mechanical evaluation of a soft tissue interference screw in free tendon anterior cruciate ligament graft fixation. Am J Sports Med. 2001; 29(1):67–71.
14. Coleridge SD, Amis AA. A comparison of five tibial-fixation systems in hamstring-graft anterior cruciate ligament reconstruction. Knee Surg Sports Traumatol Arthrosc. 2004; 12(5):391–397.
15. Halewood C, Hirschmann MT, Newman S, Hleihil J, Chaimski G, Amis AA. The fixation strength of a novel ACL soft-tissue graft fixation device compared with conventional interference screws: a biomechanical study in vitro. Knee Surg Sports Traumatol Arthrosc. 2011; 19(4):559–567.
16. Fleming BC, Magarian EM, Harrison SL, Paller DJ, Murray MM. Collagen scaffold supplementation does not improve the functional properties of the repaired anterior cruciate ligament. J Orthop Res. 2010; 28(6):703–709.
17. Murray MM, Magarian EM, Harrison SL, Mastrangelo AN, Zurakowski D, Fleming BC. The effect of skeletal maturity on functional healing of the anterior cruciate ligament. J Bone Joint Surg Am. 2010; 92(11):2039–2049.
18. Seil R, Rupp S, Krauss PW, Benz A, Kohn DM. Comparison of initial fixation strength between biodegradable and metallic interference screws and a press-fit fixation technique in a porcine model. Am J Sports Med. 1998; 26(6):815–819.
19. Tetsumura S, Fujita A, Nakajima M, Abe M. Biomechanical comparison of different fixation methods on the tibial side in anterior cruciate ligament reconstruction: a biomechanical study in porcine tibial bone. J Orthop Sci. 2006; 11(3):278–282.
20. Greis PE, Burks RT, Bachus K, Luker MG. The influence of tendon length and fit on the strength of a tendon-bone tunnel complex: a biomechanical and histologic study in the dog. Am J Sports Med. 2001; 29(4):493–497.
21. Gobbi A, Domzalski M, Pascual J, Zanazzo M. Hamstring anterior cruciate ligament reconstruction: is it necessary to sacrifice the gracilis? Arthroscopy. 2005; 21(3):275–280.
22. Kyung HS, Oh CW, Lee HJ. Clinical evaluation of anterior cruciate ligament reconstruction with remnant-preserving technique: method using single four-strand semitendinosus tendon. J Korean Orthop Assoc. 2011; 46(1):60–67.
23. Streich NA, Friedrich K, Gotterbarm T, Schmitt H. Reconstruction of the ACL with a semitendinosus tendon graft: a prospective randomized single blinded comparison of double-bundle versus single-bundle technique in male athletes. Knee Surg Sports Traumatol Arthrosc. 2008; 16(3):232–238.
24. Zamarra G, Fisher MB, Woo SL, Cerulli G. Biomechanical evaluation of using one hamstrings tendon for ACL reconstruction: a human cadaveric study. Knee Surg Sports Traumatol Arthrosc. 2010; 18(1):11–19.
25. Paschal SO, Seemann MD, Ashman RB, Allard RN, Montgomery JB. Interference fixation versus postfixation of bone-patellar tendon-bone grafts for anterior cruciate ligament reconstruction: a biomechanical comparative study in porcine knees. Clin Orthop Relat Res. 1994; (300):281–287.
26. Donahue TL, Gregersen C, Hull ML, Howell SM. Comparison of viscoelastic, structural, and material properties of double-looped anterior cruciate ligament grafts made from bovine digital extensor and human hamstring tendons. J Biomech Eng. 2001; 123(2):162–169.
27. Morrison JB. The mechanics of the knee joint in relation to normal walking. J Biomech. 1970; 3(1):51–61.