Abstract
Transvaginal ultrasound-guided follicle aspiration is one method of obtaining recipient oocytes for equine somatic cell nuclear transfer (SCNT). This study was conducted: (1) to evaluate the possibility of oocyte aspiration from pre-ovulatory follicles using a short disposable needle system (14-G) by comparing the oocyte recovery rate with that of a long double lumen needle (12-G); (2) to investigate the developmental competence of recovered oocytes after SCNT and embryo transfer. The recovery rates with the short disposable needle vs. the long needle were not significantly different (47.5% and 35.0%, respectively). Twenty-six SCNT embryos were transferred to 13 mares, and one mare delivered a live offspring at Day 342. There was a perfect identity match between the cloned foal and the cell donor after analysis of microsatellite DNA, and the mitochondrial DNA of the cloned foal was identical with that of the oocyte donor. These results demonstrated that the short disposable needle system can be used to recover oocytes to use as cytoplasts for SCNT, in the production of cloned foals and for other applications in equine embryology
Although the successful production of equine offspring by somatic cell nuclear transfer (SCNT) [1129] occurred later than in other animal species like sheep [627] and pigs [19], cloned foals have since been reported consistently, albeit rarely [1217]. Similar to bovine and porcine SCNT, in vitro matured (IVM) oocytes derived from ovaries of slaughtered mares have been used as recipient cytoplasts in equine SCNT [4111317]. However, this method is limited in that the efficiency of IVM of equine oocytes is not very high (63-68%) [524] compared with that of cattle and pigs (over than 80%) [162326]. In addition, because there is an increasing aversion to horse slaughtering, the use of IVM equine oocytes from excised ovaries has become increasingly difficult [28].
For these reasons, the value of in vivo matured oocytes recovered by transvaginal ultrasound-guided follicle aspiration (TVUFA) is increasing, and several studies on their use have recently conducted [1715]. Oocyte recovery by TVUFA has been used in various species including humans [238] and horse [1029]. Because the recovery of equine oocytes by simple aspiration is not very efficient, a double lumen needle system for flushing follicles is needed for TVUFA in horse [18]. However, commercial double long needles are relatively expensive compared to the single, disposable short needle used in bovine TVUFA; therefore, some researchers have used the same needle in several mares to reduce costs even though this may cause reproductive problems (i.e., infections) in mares.
In this study, a short double-lumen needle system modified from the short needle single lumen system used for cattle TVUFA was tested for the recovery of equine follicular oocytes, and their developmental competence after SCNT and embryo transfer (ET) was investigated.
Fifty thoroughbred mares from 6 to 9 years of age, weighing 430 to 470 kg, were used between March and August 2009. Before the experiment, mares underwent clinical evaluation by transrectal palpation and ultrasonographic examination of the genital tract.
Unless otherwise stated, all chemicals were purchased from Sigma-Aldrich (USA). The base medium for IVM was tissue culture medium (TCM)-199 (Invitrogen, USA) supplemented with 0.6 mM cysteine, 1.0 mM pyruvate, 10 mU/mL FSH (Folltropin-V; Bioniche Animal Health, Canada), 50 ng/mL EGF, 1 µg/mL insulin-transferrin-sodium, 25 µg/mL gentamycin and 10% (v/v) fetal bovine serum (FBS) from the same batch. In vitro culture (IVC) medium was a 1:1 mixture of Dulbecco's modified Eagle's medium (D-MEM) (Invitrogen) and nutrient mixture F-12 (D-MEM/F-12) (Invitrogen) supplemented with 10% FBS.
A real-time ultrasound scanner (Mylab30Vet; Esaote, Italy) equipped with a 7.5 MHz convex array transducer (model EC123) housed in a hard plastic vaginal device with stainless steel needle guidance was used. Two types of needles were compared, a 12-G double lumen needle (V-EOAD-1260L; Cook Medical, Australia) and a 14-G double lumen needle system using a short disposable needle (Bovi-vet; Kruuse, Denmark). A vacuum pump was attached to the needle, and the aspiration pressure was adjusted to -150 mmHg for the 12-G needle and -200 mmHg for the 14-G needle. Before follicle aspiration, mares were restrained in stocks and sedated with 0.6 mg/kg xylazine intravenously (iv), 0.03 mg/kg acepromazine iv and 0.01 mg/kg butorphanol tartrate iv, as well as 0.1 mg/kg propantheline bromide iv for rectal relaxation. The ultrasound transducer was inserted into the fornix of the vagina, and the ovary was attracted transrectally to lie against the vaginal wall near the transducer. The follicles were aspirated by inserting the needle into the follicular cavity while viewing through the monitor of the ultrasound scanner, then massaged per rectum and flushed continuously with 150 to 200 mL of commercial flushing solution (Vigro; Bioniche Animal Health) containing 10 units/mL heparin. After the aspiration procedure, the fluid was immediately taken to a laboratory and examined under a stereomicroscope for recovery of cumulus-oocyte complexes (COCs).
Recovered COCs were classified as follows according to the morphology of the cumulus (Fig. 1) [14]: (1) compact (Co, COCs with cumulus or corona cells tightly surrounding the oocyte); (2) expanded (Ex, COCs with well-expanded cumulus or surrounded by a mucous matrix); (3) denuded (De, COCs with only corona radiata or a partial layer of cumulus). After washing in IVM medium, each COC was placed into one well of a four-well multi-dish (Nunc, Denmark) containing 500 µL IVM medium and cultured at 38.5℃ in a humidified atmosphere of 5% CO2 in air for 13 to 16 h (Ex) or 24 to 27 h (Co).
After transport from the slaughterhouse to the laboratory at 30℃ (within 0.5-1 h), ovaries were cleaned by trimming the adnexa with scissors and washing with sterile saline. All visible follicles were opened with a scalpel and the follicular wall was scraped using a bone curette. Scraped contents were washed into petri dishes with D-MEM/F-12 supplemented with 0.05% (w/v) polyvinyl alcohol and examined under a stereomicroscope. Cumulus-oocyte complexes were classified and held in a mixture of 40% (v/v) TCM-199 with Earle's salts (Invitrogen) and 40% (v/v) TCM-199 with Hanks' salts (Invitrogen) with 20% (v/v) FBS [20] at room temperature for 23 h (holding treatment). After the holding treatment, COCs were washed with fresh IVM medium and cultured at 38.5℃ in a humidified atmosphere of 5% CO2 in air for 19 to 20 h.
Skin fibroblasts from Warmblood stallions were seeded into a four-well plate and grown in D-MEM/F-12 (Invitrogen) supplemented with 15% (v/v) FBS until a complete monolayer formed. Donor cells were induced to synchronize at the G0/G1 stage of the cell cycle by contact inhibition for 24 to 72 h. Cells of the same passage (3-6 passages) were used in each replicate for the various treatments. A single cell suspension was prepared by trypsinization of cultured cells and resuspension in D-MEM containing 0.4% (w/v) BSA (MEM-BSA) prior to nuclear transfer.
After IVM, cumulus-cell-free oocytes from which cumulus cells had been removed by exposure to 15% (w/v) hyaluronidase and repeated pipetting through a small glass pipette were incubated for 10 min in manipulation medium (MEM-BSA) containing 5 µg/mL cytochalasin B. Following incubation, the oocytes were transferred into a drop of manipulation medium on a glass dish (WillCo-dish; Willco Wells, The Netherlands) and the zonae pellucidae were penetrated with a 12-µm blunt glass pipette (Humagen, USA) using a Piezo drill (PMM-150FU; PRIME TECH, Japan). Oocytes were enucleated by aspirating the first polar body and the adjacent cytoplasm containing the MII chromosomes, which were confirmed by the absence of metaphase spindles under a microscope (Ti-U; Nikon, Japan) equipped with a polarization-based optical instrument (Oosight; Cambridge Research and Instrumentation, USA). Following enucleation, a single donor cell was inserted into the perivitelline space of each oocyte. The oocyte-cell couplets were then placed on a 0.5 mm fusion chamber overlaid with 280 mM mannitol containing 0.05 mM CaCl2 and 0.1 mM MgCl2. Membrane fusion was induced by applying two pulses of 2.2 to 2.4 kV/cm direct current for 15 µsec using a cell fusion generator (LF101; Nepa Gene, Japan). After incubation in MEM-BSA for 15 min, non-fused couplets were subjected to subsequent electric pulses. Reconstructed oocytes were activated at 1 to 2 h after fusion by exposure to medium containing 5 µM ionomycin for 4 min, followed by washing and incubation in IVC medium containing 2 mM 6-dimethylaminopurine at 38.5℃ in a humidified atmosphere of 5% CO2 in air for 5 h.
Following the activation procedure, SCNT embryos were transferred to recipient mares (1-3 embryos/mare) by standing flank laparotomy within 24 h of ovulation [29]. Briefly, after treatment with a combination of 0.6 mg/kg xylazine iv, 0.03 mg/kg acepromazine iv and 0.02 mg/kg butorphanol tartrate iv, approximately 150 mL of lidocaine was instilled into the paralumbar fossa of the mare for local anesthesia (inverted L block). The ovary and oviduct ipsilateral to ovulation were exteriorized, and SCNT embryos were transferred into the mid-ampullary region of the oviduct using a 14 cm catheter (Tom Cat Catheter; Kendall, USA). After initial detection of pregnancy by transrectal ultrasonography (5 MHz transducer) between Days 14 and 16 (Day 0 = surgical transfer), mares were subsequently examined at Days 21, 30, 50 and 60 of gestation. After Day 100, pregnancy examinations were performed by transabdominal ultrasonography (2.5 MHz transducer) at monthly intervals.
Parentage analysis was performed on the foal produced by SCNT to confirm genetic identity with the somatic donor cells. The DNA from blood and cells was extracted by the standard proteinase K/phenol-chloroform procedure [21]. The extracted genomic DNA samples were subjected to microsatellite assay using a 17-plex Applied Biosystems (USA) horse genotyping kit (VHL20, HTG4, AHT4, HMS7, HTG6, HMS6, HTG7, HMS3, AHT5, ASB2, HTG10, HMS2, ASB17, LEX3, HMS1, CA425, and ASB23) labeled with one of the fluorescent dyes, FAM, NED, PET or VIC. Polymerase chain reaction (PCR) products were generated in 15 µL reactions containing 10 ng DNA, 2.5 µL StockMarks PCR buffer, 4 µL dNTP mix, 4 µL of 17 primer sets (one for each of the microsatellite markers) and 2.5 U Taq DNA polymerase (Applied Biosystems). Amplification was carried out in a GeneAmp PCR System 9700 thermal cycler (Applied Biosystems). The reaction profiles included the following: one cycle of denaturation at 95℃ for 10 min, followed by 30 cycles of denaturation at 95℃ for 30 sec, annealing at 60℃ for 30 sec and extension at 72℃ for 60 sec and then final extension at 72℃ for 60 min. PCR products were resolved with an internal size standard (GeneScan 500 LIZ; Applied Biosystems). Genotyping was performed by electrophoresis on an automated DNA sequencer (ABI 3100; Applied Biosystems), and genotype data were analyzed using the GeneScan and Genotyper software (Applied Biosystems).
Equine mitochondrial D-loop analysis was performed to confirm mitochondrial genome identity on the foal produced by SCNT and on the oocyte donor cell used for SCNT. We sequenced the equine mitochondrial D-loop, which is ~1 kb including the D-loop adjacent region, in 11 mtDNA samples. Two primer sets for amplification and sequencing analysis were designed based on GenBank sequences (NCBI accession no. X79547). The primer sequences of the two PCR primer sets were: D-loop1-Forward: TCCCCCTCATATTAAACCAGAA, Reverse: CATGTCAGGTGGGTATGGTG; D-loop2-Forward: AACGTTTCCTCCCAAGGACT, Reverse: GTGTGAGCATGGGCTGATTA. PCR was performed in a mixture of 1.25 pM of each primer, 30 ng genomic DNA, 250 µM dNTPs and 1.5 U Taq DNA polymerase (Applied Biosystems) in the buffer provided by the manufacturer. Amplification was carried out in a GeneAmp PCR System 9700 thermal cycler (Applied Biosystems) under touch-down conditions [9]. Nucleotide sequencing was performed using the Big Dye Terminator version 3.1 Cycle Sequencing kit (Applied Biosystems) and an ABI 3730 Genetic Analyzer (Applied Biosystems). Sequence variants were verified by means of chromatograms using the DNA star software.
In experiment 1, the type of needle used for TVUFA in mares was examined. Two types of needle were compared, a 12-G double lumen needle and a 14-G double lumen needle system using a short disposable needle. To determine the effects of follicular size on recovery rates, the diameters of ovarian follicles were first measured. Follicles were classified into three types: (1) Group A (from 25 to 30 mm); (2) Group B (from 30 to 35 mm); (3) Group C (> 35 mm). In experiment 2 and 3, the developmental competence of oocytes recovered in vivo using TVUFA with a short needle was investigated by IVM and SCNT. To determine the maturation rate according to the status of COCs, oocytes were denuded and examined for condition of the cytoplasm and extrusion of the first polar body (PB). In vitro matured oocytes recovered by scraping follicles from ovaries of slaughtered mares were used at the same time to compare their in vivo development after SCNT and ET. In experiment 4, nuclear DNA extracted from donor cells and blood samples of the cloned foal, the oocyte donor and the recipient mare and analyzed to determine the identity of the cloned foal. Mitochondrial DNA was also analyzed to confirm that the foal was not genetically related to the recipient mare.
All analyses were performed using the Statistical Analysis System (ver. 9.1; SAS Institute, USA). Data were analyzed using a general linear model followed by the least significant difference mean separation procedure when treatments differed at p < 0.05. Percentage data were arcsine transformed prior to analysis to maintain homogeneity of variances. Percentage data are expressed as the means ± SEM.
The recovery rate (47.5%) with the 14-G short needle system did not differ significantly from that (35.0%) using the 12-G long needle (Table 1). Comparison of the recovery rate according to the size of follicles revealed recovery rates of Group A, B and C of 55.7% (n = 56), 41.4% (n = 68) and 36.5% (n = 24), respectively.
Table 2 shows the IVM status of equine oocytes according to the type of COCs. The maturation rate of Ex oocytes (97.1%) was not different from that of Co oocytes matured for 24 to 26 h or for 28 to 30 h (83.3% and 91.0%, respectively). The percentage of oocytes without PB (13.9%) in Co oocytes matured for 24 to 26 h was not significantly different from that (0.0%) in Ex oocytes.
In vivo efficiency of oocytes recovered by TVUFA (in vivo oocytes) was compared with that of oocytes derived from slaughtered ovaries (in vitro oocytes) after SCNT and ET (Table 3). The fusion rate of in vivo oocytes was not different from that of in vitro oocytes. After the transfer of SCNT embryos derived from in vitro oocytes, one embryo formed embryonic vesicles (1/42 = 2.4%) at early pregnancy examination, but this could not be maintained over 30 days. Among 26 SCNT embryos derived from in vivo oocytes, three formed embryonic vesicles (3/26 = 11.5%) after transfer to 13 recipient mares and one went to term (1/26 = 3.8%, gestation periods: 342 days) (Fig. 2). The cloned foal has grown to date without any observed abnormalities.
Parentage analysis data of the donor cell, the cloned foal, the oocyte donor and the recipient mare are shown in Tables 4 and 5. Analysis of seventeen equine specific microsatellite loci confirmed that the cloned foal was genetically identical to the donor cell, and there was no genetic relationship between the cloned foal and its surrogate mother (Table 4). Analysis of mitochondrial DNA revealed perfect homology between the cloned foal and the oocyte donor, while the mtDNA of the cloned foal was different from that of the donor cell and the surrogate mother (Table 5).
While equine SCNT is an appealing topic, it has not been a major field of study to date because it is difficult to obtain sufficient numbers of ovaries and a great deal of time and effort is required to recover oocytes matured in vivo using TVUFA. The maturation efficiency of in vivo equine oocytes recovered by TVUFA is higher than that of in vitro oocytes derived from slaughtered ovaries [2425], but this approach may be restricted by variations in recovery rates depending on personal skills and/or the high cost for maintenance of donor mares.
The portion of Ex oocytes was lower using the 14-G needle system than the 12-G needle, perhaps because their expanded cumulus cells embedded in mucus are difficult to pass through the narrower inner lumen of the 14-G needle. Actually, the inner needle was sometimes clogged during aspiration of large follicles, and ruptured oocytes were recovered when the negative pressure was increased to prevent this phenomenon. Therefore, it seems that use of a larger inner needle is needed to increase the efficiency of the short disposable needle system.
The oocyte recovery rate from larger follicles was lower than that of smaller follicles in our study. The reason for this is not clear, but it is highly likely that it was due to difficulty in controlling the ovary with one hand when larger follicles were present. For recovery of equine follicular oocytes, it is important not only to flush the follicle with recovery medium, but also to simultaneously massage the follicle with the hand holding the ovary (Dr. D. Vanderwall, personal communication). It appears that massage of the follicle during flushing was not sufficient in cases in which two large follicles were developed in one ovary.
The rate of in vitro maturation of oocytes recovered by TVUFA was 83.3 to 97.1%, which is slightly higher than the results observed in other studies [1525]. The variations in maturation rates are likely a result of the different maturation systems employed among laboratories and/or the different sizes of aspirated follicles. Surgical transfer of 1-cell embryos immediately after SCNT [29] is more difficult and expensive than non-surgical transfer after in vitro culture of SCNT embryos [1117]. However, it has the advantage of providing a better environment for equine SCNT embryos, which have a lower developmental rate after IVC than those of cattle and pigs. Although equine SCNT studies have frequently been reported, there have been only a few reports of foal production after SCNT embryo transfer [11131729]. Our study produced an efficiency of live foal production of 3.8% (1/26), which is typical of cloned foal production.
In this study, a recipient mare delivered a live foal on 12 May 2010. The foal weighed 47.7 kg at birth and grew without any problems (Fig. 2; currently 20 months of age). The foal is the first cloned equid reported in Asia. For DNA analysis, a blood sample from the cloned foal and frozen donor cells was used because the donor stallion died before the foal was born. Unlike other studies that reported the production of cloned foals, mitochondrial D-loop analysis was performed in addition to microsatellite DNA analysis in the present study. While it is difficult to secure individual information regarding in vitro oocytes derived from slaughterhouse ovaries, the in vivo oocytes recovered by TVUFA have the major advantage of being easy to confirm the origin of mares. Although mtDNA has been reported to be related to milk production in cattle [22], few studies of the roles of equine mtDNA have been conducted to date. Accordingly, further investigation of equine SCNT using oocytes recovered by TVUFA is needed to determine the relationships between mtDNA and characteristics of horses.
The results of this study confirmed that the short needle system could be adapted to recovery of equine follicular oocytes by TVUFA, and that the developmental competence of oocytes recovered with this system could be maintained after SCNT and transfer to recipient mares, culminating in the birth of a healthy foal.
Figures and Tables
Fig. 1
Representative pictures of various equine oocytes. (A) Ex-cumulus oocyte complexes (COCs) with well-expanded cumulus or mucous. (B) COCs with cumulus or corona cells tightly surrounding oocytes. (C) COCs with only corona radiata or partial layer of cumulus. (D) COCs with ruptured cytoplasm. (E) Oocytes without the first polar body. (F) Oocytes with degenerated cytoplasm.
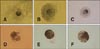
Table 2
In vitro maturation of equine oocytes recovered from pre-ovulatory follicles by the short needle system

Acknowledgments
The authors thank Ms. Jihyun Park, Mr. Joohyung Lee, and Dr. Yongwoo Chun for excellent technical assistance. This study was financially supported by National Research Foundation (grant no. NRF-2011-0014941), Institute of Planning and Evaluation for Technology in Food, Agriculture, Forestry and Fisheries (no. 109023-05-1-CG000), and the Brain Korea 21 plus program for Veterinary Science and the Research Institute for Veterinary Science.
References
1. Altermatt JL, Suh TK, Stokes JE, Carnevale EM. Effects of age and equine follicle-stimulating hormone (eFSH) on collection and viability of equine oocytes assessed by morphology and developmental competency after intracytoplasmic sperm injection (ICSI). Reprod Fertil Dev. 2009; 21:615–623.


2. Bols PEJ, Vandenheede JMM, Van Soom A, de Kruif A. Transvaginal ovum pick-up (OPU) in the cow: a new disposable needle guidance system. Theriogenology. 1995; 43:677–687.


3. Cheon KW, Song SJ, Choi BC, Lee SC, Lee HB, Yu SY, Yoo KJ. Comparison of clinical efficacy between a single administration of long-acting gonadotrophin-releasing hormone agonist (GnRHa) and daily administrations of short-acting GnRHa in in vitro fertilization-embryo transfer cycles. J Korean Med Sci. 2008; 23:662–666.


4. Choi YH, Love CC, Varner DD, Love LB, Hinrichs K. Effects of gas conditions, time of medium change, and ratio of medium to embryo on in vitro development of horse oocytes fertilized by intracytoplasmic sperm injection. Theriogenology. 2003; 59:1219–1229.


5. Choi YH, Love LB, Varner DD, Hinrichs K. Holding immature equine oocytes in the absence of meiotic inhibitors: effect on germinal vesicle chromatin and blastocyst development after intracytoplasmic sperm injection. Theriogenology. 2006; 66:955–963.


6. Cibelli JB, Stice SL, Golueke PJ, Kane JJ, Jerry J, Blackwell C, Ponce de León FA, Robl JM. Cloned transgenic calves produced from nonquiescent fetal fibroblasts. Science. 1998; 280:1256–1258.


7. Colleoni S, Duchi R, Lazzari G, Galli C. Embryo production by ovum pickup-intracytoplasmic sperm injection-IVC in an equine ovum pickup program using semen from fertile and infertile stallions. Reprod Fertil Dev. 2010; 22:345.


8. De Roover R, Feugang JMN, Bols PEJ, Genicot G, Hanzen Ch. Effects of ovum pick-up frequency and FSH stimulation: a retrospective study on seven years of beef cattle in vitro embryo production. Reprod Domest Anim. 2008; 43:239–245.


9. Don RH, Cox PT, Wainwright BJ, Baker K, Mattick JS. 'Touchdown' PCR to circumvent spurious priming during gene amplification. Nucleic Acids Res. 1991; 19:4008.


10. Galli C, Crotti G, Turini P, Duchi R, Mari G, Zavaglia G, Duchamp G, Daels P, Lazzari G. Frozen-thawed embryos produced by ovum pick up of immature oocytes and ICSI are capable to establish pregnancies in the horse. Theriogenology. 2002; 58:705–708.


11. Galli C, Lagutina I, Crotti G, Colleoni S, Turini P, Ponderato N, Duchi R, Lazzari G. Pregnancy: a cloned horse born to its dam twin. Nature. 2003; 424:635.
12. Hinrichs K, Choi YH, Love CC, Chung YG, Varner DD. Production of horse foals via direct injection of roscovitine-treated donor cells and activation by injection of sperm extract. Reproduction. 2006; 131:1063–1072.


13. Hinrichs K, Choi YH, Varner DD, Hartman DL. Production of cloned horse foals using roscovitine-treated donor cells and activation with sperm extract and/or ionomycin. Reproduction. 2007; 134:319–325.


14. Hinrichs K, Schmidt AL, Friedman PP, Selgrath JP, Martin MG. In vitro maturation of horse oocytes: characterization of chromatin configuration using fluorescence microscopy. Biol Reprod. 1993; 48:363–370.


15. Jacobson CC, Choi YH, Hayden SS, Hinrichs K. Recovery of mare oocytes on a fixed biweekly schedule, and resulting blastocyst formation after intracytoplasmic sperm injection. Theriogenology. 2010; 73:1116–1126.


16. Kim J, You J, Hyun SH, Lee G, Lim J, Lee E. Developmental competence of morphologically poor oocytes in relation to follicular size and oocyte diameter in the pig. Mol Reprod Dev. 2010; 77:330–339.


17. Lagutina I, Lazzari G, Duchi R, Colleoni S, Ponderato N, Turini P, Crotti G, Galli C. Somatic cell nuclear transfer in horses: effect of oocyte morphology, embryo reconstruction method and donor cell type. Reproduction. 2005; 130:559–567.


18. Mari G, Barbara M, Eleonora I, Stefano B. Fertility in the mare after repeated transvaginal ultrasound-guided aspirations. Anim Reprod Sci. 2005; 88:299–308.


19. Polejaeva IA, Chen SH, Vaught TD, Page RL, Mullins J, Ball S, Dai Y, Boone J, Walker S, Ayares DL, Colman A, Campbell KHS. Cloned pigs produced by nuclear transfer from adult somatic cells. Nature. 2000; 407:86–90.


20. Ribeiro BI, Love LB, Choi YH, Hinrichs K. Transport of equine ovaries for assisted reproduction. Anim Reprod Sci. 2008; 108:171–179.


21. Sambrook J, Fritsch EF, Maniatis T. Preparation of radiolabeled DNA and RNA probes. Molecular Cloning: A Laboratory Manual. 2nd ed. Cold Spring Harbor Laboratory: New York;1989. p. 9.14–9.23.
22. Schutz MM, Freeman AE, Beitz DC, Mayfield JE. The importance of maternal lineage on milk yield traits of dairy cattle. J Dairy Sci. 1992; 75:1331–1341.


23. Song K, Lee E. Modification of maturation condition improves oocyte maturation and in vitro development of somatic cell nuclear transfer pig embryos. J Vet Sci. 2007; 8:81–87.


24. Tharasanit T, Colleoni S, Lazzari G, Colenbrander B, Galli C, Stout TA. Effect of cumulus morphology and maturation stage on the cryopreservability of equine oocytes. Reproduction. 2006; 132:759–769.


25. Torner H, Alm H, Kanitz W, Goellnitz K, Becker F, Poehland R, Bruessow KP, Tuchscherer A. Effect of initial cumulus morphology on meiotic dynamic and status of mitochondria in horse oocytes during IVM. Reprod Domest Anim. 2007; 42:176–183.


26. Voelkel SA, Hu YX. Use of ethylene glycol as a cryoprotectant for bovine embryos allowing direct transfer of frozen-thawed embryos to recipient females. Theriogenology. 1992; 37:687–697.


27. Wilmut I, Schnieke AE, McWhir J, Kind AJ, Campbell KHS. Viable offspring derived from fetal and adult mammalian cells. Nature. 1997; 385:810–813.


28. Whiting TL. The United States' prohibition of horsemeat for human consumption: is this a good law? Can Vet J. 2007; 48:1173–1180.