Abstract
Purpose
The purpose of this study was to investigate the effects of transplantation of an in vitro-generated, scaffold-free, tissue-engineered cartilage tissue analogue (CTA) using a suspension chondrocyte culture in a rabbit growth-arrest model.
Materials and Methods
We harvested cartilage cells from the articular cartilage of the joints of white rabbits and made a CTA using a suspension culture of 2×107 cells/mL. An animal growth plate defect model was made on the medial side of the proximal tibial growth plate of both tibias of 6-week-old New Zealand white rabbits (n=10). The allogenic CTA was then transplanted onto the right proximal tibial defect. As a control, no implantation was performed on the left-side defect. Plain radiographs and the medial proximal tibial angle were obtained at 1-week intervals for evaluation of bone bridge formation and the degree of angular deformity until postoperative week 6. We performed a histological evaluation using hematoxylin-eosin and Alcian blue staining at postoperative weeks 4 and 6.
Results
Radiologic study revealed a median medial proximal tibial angle of 59.0° in the control group and 80.0° in the CTA group at 6 weeks. In the control group, statistically significant angular deformities were seen 3 weeks after transplantation (p<0.05). On histological examination, the transplanted CTA was maintained in the CTA group at 4 and 6 weeks postoperative. Bone bridge formation was observed in the control group.
In childhood and adolescence, growth plate injuries can cause premature arrest of the growth plate with bone bridge, which can result in leg-length discrepancy and angular deformity. Limb shortening and angulation caused by growth plate injuries is a major problem encountered by many physicians, and treatment is more challenging in younger patients.12 Nevertheless, standard treatment has not yet been established. Bone bridge resection and variable implantation materials, such as fat,3456 bone wax,4 silastic,78 free growth plate,9 and vascularized growth plate,10 have been applied clinically and experimentally to treat growth plate injuries. However, these procedures have limitations, including bone growth control and long-term safety of implant materials in vivo. As alternatives, homogeneous or heterogeneous cartilage cells and stem cell transplants have been used,11121314 although doing so requires a scaffold for cell transplantation. To date, no ideal scaffold has been developed.
Researchers recently generated a cartilage tissue analogue (CTA) using a suspension culture with biophysical properties similar to those of native hyaline cartilage.151617 This allows the CTA to be transplanted without a scaffold to treat the physeal defect. However, to the best of our knowledge, there are no reports on a scaffold-free CTA for the treatment of physeal cartilage injury. The purpose of this study was to evaluate the effects of transplantation of an in vitro-generated, allogenic scaffold-free CTA in a rabbit growth-arrest model via measurement of the medial proximal tibial angle (MPTA) and histological evaluation of the newly formed cartilaginous tissue.
The Institutional Animal Care and Use Committee of our institution, approved all the animal experiments. Articular cartilage was harvested from the knee and hip joints of male New Zealand white rabbits weighing 2.0 to 2.5 kg each.
Articular cartilage from the femoral head, distal femoral condyles, and tibial plateau was harvested under sterile conditions from 5-week-old New Zealand white rabbits within 8 h of death. The total number of the New Zealand white rabbits was five. The procedure for isolation of chondrocytes and fabrication of CTA has been previously described.16 The cartilage was held in Dulbecco's minimum essential medium (DMEM; Mediatech, Herndon, VA, USA) at 4℃ until chondrocytes were isolated, which occurred within several hours (<8 h). The soft tissue and perichondrium were removed, and the cartilage was isolated free of any growth plate before cutting it into small pieces. The harvested cartilage was sectioned into small pieces of approximately 3 mm3. The articular cartilage was then washed several times with Hanks' balanced salt solution containing 2× antibiotics and 2× antimycotic (penicillin, 200 units/mL; streptomycin, 200 units/mL; and amphotericin B, 5.0 mg/mL). The articular cartilage was processed through enzymatic digestion with bacterial collagenase initially at 2 mg/mL for 1 h, followed by 0.5 mg/mL overnight in complete medium at 37℃. The next day, cells that were released by enzymatic digestion were filtered through a nylon mesh filter (70-mm pore size nylon cell strainer; Falcon, Becton Dickinson, Lincoln Park, NJ, USA) into a 50-mL tube. The cells were washed twice using centrifugation at 1300 rpm at 16℃ for 10 min. The cells were not pooled but were allowed to remain separate after isolation. As a result, chondrocytes were isolated, and this pool of cells was used to prepare the constructs. After the final wash, the cells were resuspended in a fresh DMEM containing 10% FBS medium. The average yield was 4.0×107 cells/g of tissue. The isolated chondrocytes were plated at a density of 2×107/well/mL in a 24-well plate (2.4 cm2/well) treated with poly-hydroxyethyl methacrylate (or low cluster plate; Becton, Dickinson and Company, Franklin Lakes, NJ, USA). Some investigators have reported that growth of cartilage-like material is most efficiently achieved when cultures of high density (>2.0×107/mL) are used.18
The culture medium used throughout the experiment was a complete medium with 50-µg/mL ascorbic acid, and was changed every 2 to 3 days. The cells were incubated at 37℃ in 5% CO2. The chondrocytes were maintained in culture with a fresh medium replaced every 3 or 4 days. The CTA, which has similar mechanical properties to native cartilage, was transplanted to the physeal defect after 8 weeks of culture.16 We also histologically analyzed the CTA using Alcian blue staining and immunofluorescence.
The number of rabbits was 14. However, the final analysis included only 10 cases, excluding four cases where the animals had a fracture, a wound complication, died from an anesthetic problem, or were killed at postoperative week 4 for histological evaluation, respectively.
Six-week-old New Zealand white rabbits were anesthetized using an intramuscular injection of 0.3 mL/kg of Zoletile (Zoletile 50; Virbac S.A., Carros, France). Rabbits underwent operation in a similar method to that previously reported by Yoshida, et al.19 The medial side of the knee joint of each of the New Zealand white rabbits was shaved, prepped, and draped for aseptic surgery in the supine position. A 3-cm anteromedial incision was made, and the tissue overlying the proximal tibia was dissected. A straight incision was made below the knee joint to prevent a contracted scar. An experimental model of growth plate injury was created by excising the growth plate at the proximal medial side of the tibia (4-mm diameter and 3-mm depth) using a 4-mm harvester of the Consistent Osteochondral Repair (COR) system (Mitek, Westwood, MA, USA) (Fig. 1A). Mean growth plate length of the proximal tibia was 15 mm in the AP radiologic image, and 27% defect of the growth plate was made using a harvester with a diameter of 4 mm. After sterile saline water irrigation, the CTA was implanted onto the proximal tibial defect (CTA group) (Fig. 1B). Transplant stability was achieved through press fit fixation and tight suturing using the edges of the periosteum with absorbable suture (Vicryl 4-0, Ethicon, Livingston, Scotland). We did not perform any special procedure to fix the transplanted CTA, except press fit fixation and tight repair of the periosteum and soft tissue. We think that the transplanted material might have been fixed on the site because CTA was inserted in the bone hole and periosteum, and the subcutaneous tissue was repaired tightly. For controls, a defect of the same size was made on the medial side of the left proximal tibial growth plate without any graft in the same rabbit. Wound incision was closed using a continuous running suture of 4-0 nylon. The rabbits were allowed to walk freely in the cage.
Plain radiographs obtained at 1-week intervals were examined to evaluate bone bridge formation. Under anesthesia induced with an intramuscular injection of 0.3-mL/kg Zoletile 50 (Virbac S.A.), radiographs were obtained with the animals in the supine position and with 2-cm-thick Styrofoam placed under the calf. The MPTA was measured to assess the degree of angular deformity until postoperative week 6. The MPTA was performed according to the system described by Paley, et al.20 and defined as the angle between the long axis of the tibia and tibial plateau in the anteroposterior lower limb (Fig. 2).19
Histological examination using hematoxylin-eosin (H&E) and Alcian blue staining was performed at postoperative weeks 4 and 6. To obtain samples for histological evaluation, only one animal was killed at postoperative week 4; the five remaining animals were killed at postoperative week 6.
Coronal sections through the operated site of the proximal tibia were made. The recovered, formalin-fixed, alcohol-preserved specimens were decalcified in 10% formic acid formalin solution for 14 days, followed by dehydration, paraffin embedding, and microsection in the direction parallel to the axis of the bone. The sections were then stained with H&E and Alcian blue. Histological observation for growth plate regeneration, bone bridge formation, and any inflammatory response were performed using an Olympus BX40 microscope (Olympus, Tokyo, Japan).
The limbs of the rabbits were divided into two groups according to the presence of CTA (control group: no transplantation, CTA group: with CTA transplantation). Statistical analysis was performed using the nonparametric Wilcoxon signed rank test with SPSS version 18.0 (SPSS Inc., Chicago, IL, USA). Relationships were considered statistically significant at a p value of <0.05.
Fig. 3A shows a characteristic micrograph of a representative Alcian blue CTA grown for 8 weeks and hypertrophic (cells with enlarged lacunae) chondrocytes. Immunofluorescence CTA staining using antibodies to detect type II collagen revealed the expression of constitutive cartilage collagen (Fig. 3B; green signal).
Gross photographs of the rabbit tibia revealed the attenuation and progression of the angular deformity for 6 weeks. In the CTA group, the right side showed greater attenuation of the angular deformity than the left side (Fig. 4).
In the plain radiographic evaluation, serial radiographs of the rabbit tibia obtained at 6 weeks after surgery showed angular deformity of the proximal tibia on the left side in the control group. However, no deformity was found on the right side in the CTA group (Fig. 5).
A statistically significant difference in MPTA was observed between the two groups at 3 weeks after surgery (p<0.05) (Fig. 6). At postoperative week 3, the control group showed a lower median MPTA than the CTA group, with the difference gradually increasing with time. The median MPTA was 80.0° in the CTA group and 59.0° in the control group at 6 weeks after surgery (Table 1). The CTA group showed subtle varus deformity of the proximal tibia in contrast to the severe varus deformity in the control group.
Histological evaluation of all the 4- and 6-week-old specimens revealed bone bridges in both groups. However, only bone bridge and fibrotic tissue formations were found at the defect site of the growth plate in the control group (Fig. 7A, C, and E). Compared to the control group, the CTA group showed not only less bone bridge formation but also proliferative chondrocyte-like cells and extracellular matrix at the defect site at 4 and 6 postoperative weeks (Fig. 7B, D, and F). The regenerated tissue showed a columnar arrangement as in normal physeal tissue and growth plate-like tissue. The cartilage matrix showed staining with Alcian blue, indicating the glycosaminoglycan in cartilages. A specimen from the CTA group obtained at 6 weeks after surgery showed morphological changes with hypertrophic maturation, chondrocyte columniation during the process of CTA differentiation. However, the areas of bone bridge formation seemed to have increased.
Chondrocytes or chondrogenic cells have been used as part of an implant for the treatment of physeal cartilage defects.1121222324 The development of a successful approach in cell therapy for regenerative medicine requires appropriate cells and an optimal scaffold.25 The extent and quality of growth plate regeneration from transplanted chondrocytes may depend on the carrier matrix with which cells are transplanted. For this purpose, many studies have placed priority on the development of scaffolds, and various scaffolds have been approved for clinical use.26 The purpose of the present study was to investigate the effects of transplantation of an in vitro-generated, scaffold-free, tissue-engineered CTA in a rabbit growth injury model by evaluating a suspension chondrocyte culture. Some investigators have suggested that a CTA, which is produced by a self-aggregating suspension culture model, bears many similarities to natural cartilage.15 This suspension culture approach has been demonstrated to produce a tissue-engineered structure with a cartilage-like phenotype.1627 A CTA does not use a foreign scaffold and may increase biocompatibility for cartilage replacement. We found that the MPTA of the control group decreased in a time-dependent manner for up to 6 weeks, indicating severe angular deformity. Analysis revealed that the injured growth plate was closed and that bone and fibrotic bridges had formed. Otherwise, the CTA group showed regeneration of the epiphyseal plate and attenuation of angular deformity, with statistical significance after 3 postoperative weeks. As a result, physeal closure was prevented by CTA transplantation until postoperative week 6. In addition, the CTA formed cartilage-like tissue similar to a growth plate at 4 and 6 postoperative weeks. We think that CTA transplantation may have efficiently prevented bone bridge formation and angular deformities in our acutely injured growth plate rabbit models.
CTA transplantation offers relative advantages in the treatment of partial growth plate injury. First, a scaffold-free CTA may reduce the potential risk of adverse effects induced by biological and artificial materials of the scaffold. Second, the transplanted CTA is similar to the columnar structure of the native growth plate, based on histological evaluation of the 4- and 6-week specimens. Therefore, the use of CTA may prevent the incidence of potential chondrogenesis by mesenchymal stem cells. To determine the quality of the repair tissue, biomechanical assessments may be a better approach. Several studies on the prevention of growth arrest using materials interposition, such as stem cell and scaffolds, in physeal injuries did not perform biomechanical assessments.2829 We previously showed that the mechanical properties of CTA from pigs are similar to those of the native cartilage.16
The CTA is a high-density suspension culture of chondrocytes. Several investigators have previously shown in this suspension culture that the chondrocyte phenotype is maintained and the proper biochemical components are produced.1827 When the cultures are started with articular chondrocytes, they continue to produce collagen type II and do not produce collagen type I, which would be indicative of their differentiation to a fibroblastic phenotype. Also, average sodium concentration of the 8-week CTA ranged from 260 to 278 mM and average T1q relaxation times from 105 to 107 ms, indicating proteoglycan content similar to that of native articular cartilage.16 Unfortunately, we did not perform biomechanical assessments of the CTA from the rabbits in this study. We will consider biomechanical assessments in our future study.
This study had several limitations. The most important limitation was the small number of cases. In addition, the present study involved an acute growth arrest model within 6 postoperative weeks. The outcome of the CTA treatment after 6 postoperative weeks is unknown. Further studies are needed to clarify the long-term outcomes of CTA treatment. Second, the study lacked imaging modalities for the evaluation of the physeal status and bone bridge formation, and another control group in which another approach, such as insertion of fat tissue and bone cement, should have been used for comparison. In addition, the plain radiographs were evaluated only to determine the bone bridge formation without micro-computed tomography, and histological scores could not be determined for quantitative comparison because of the small number of cases. Another limitation of this study was that chondrocytes from hyaline cartilage have distinct genetic properties different from those of the growth plate cartilage. Autologous chondrocytes from growth plate cartilage comprise more compatible genetic properties for the treatment of growth plate injury. However, harvesting autologous chondrocytes of the growth plate are associated with problems, such as donor site morbidity and it provides only a limited amount of bone. The transplantation of chondrocytes from hyaline cartilage has already been used in the treatment of osteochondral lesions. Several authors presented transplantation of chondrocyte from hyaline cartilage with different carriers in the defect in a growth plate injury.2930 Therefore, we may assume that hyaline cartilage for CTA is useful for the treatment of growth plate injury. In this study, rabbits were allowed free, weight-bearing movement with no immobilization of the legs after operation. We did not perform any special procedure to fix the transplanted CTA and performed experiments on both legs of the rabbit. There is a possibility that CTA was not maintained in the transplanted site and that the tibia could not withstand the weight load, although the bone defect was created in the area of 1/3 or less for tibia. These factors will affect the results of treatment. Also, an accurate radiologic image of true AP is important for measuring MPTA. Radiographs were obtained with the animals in the supine position and with 2-cm-thick Styrofoam placed under the calf under the anesthesia. However, it was not easy to get a patella frontal imaging for all data. Therefore, supplementary studies are needed to obtain accurate radiographs.
In conclusion, CTA transplantation as a treatment approach for growth plate injury minimized deformity via the attenuation of bone bridge formation. Therefore, CTA transplantation may be applied as another treatment option for growth plate injuries encountered in various clinical conditions, if further studies provide supplementary data on its effectiveness.
Figures and Tables
Fig. 1
(A) Intraoperative photograph showing an experimental model of growth arrest created by excising the growth plate at the proximal medial side of the tibia (4-mm diameter and 3-mm depth). (B) After sterile saline water irrigation, cartilage tissue analogue (black *) was implanted on the proximal tibial defect.
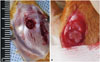
Fig. 2
The medial proximal tibial angle was defined as the angle between the long axis of the tibia and tibial plateau in the anteroposterior lower limb. MPTA, medial proximal tibial angle.
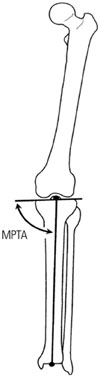
Fig. 3
Histological view of the representative cartilage tissue analogue grown for 8 weeks, stained with Alcian blue (A, ×200) and immunofluorescence (B, ×400).
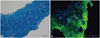
Fig. 4
(A) Gross and (B and C) cross-sectional views of the rabbit tibia at 6 weeks after surgery showing angular deformity of the proximal tibia on the left side (Lt, control group). However, the right side had no deformity (Rt, cartilage tissue analogue group).
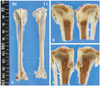
Fig. 5
Serial radiographs for both groups revealing the attenuation and progression of angular deformity for 6 weeks (A). The cartilage tissue analogue group (Rt) showed more attenuation of the angular deformity than the control group did (Lt). (B) Another serial radiograph showing the same result. Rt, right tibia; Lt, left tibia; W, week.
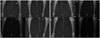
Fig. 6
Radiographic evaluation of growth plate regeneration. Compared with the control group, the CTA group showed a higher average medial proximal tibia angle, with the difference gradually increasing with statistical significance at 3 postoperative weeks (*p<0.05). CTA, cartilage tissue analogue; MPTA, medial proximal tibial angle.
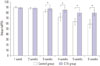
Fig. 7
Histological evaluation. (A and C) Histological evaluation of the 4-week-old specimens revealed that new bone was formed (black *) in the excised epiphyseal plate area and it partially closed the growth plate in the control group. (B and D) The CTA group showed regeneration of the growth plate and slight bone bridge formation, that is, longitudinally columnar chondrocytes (arrowheads; black arrows: normal epiphyseal plate). (E) Histological evaluation of the 6-week-old specimens revealed bone bridge formation that replaced the area of the excised epiphyseal plate in the control group. (F) The CTA group revealed regeneration of the epiphyseal plate and some bone bridge formation (A and B: hematoxylin-eosin staining, ×40; C and D: Alcian blue staining, ×40; E and F: Alcian blue staining, ×40). CTA, cartilage tissue analogue.
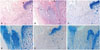
Table 1
Medial Proximal Tibial Angle after Surgery
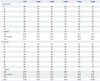
ACKNOWLEDGEMENTS
This work was supported by the Institute of Clinical Medicine Research of Bucheon St. Mary's Hospital, Research Fund, BCMC12IA01.
This study was conducted at Bucheon St. Mary's Hospital, College of Medicine, The Catholic University of Korea, Bucheon, Korea.
References
1. Langenskiöld A. Surgical treatment of partial closure of the growth plate. J Pediatr Orthop. 1981; 1:3–11.


2. Lee EH, Gao GX, Bose K. Management of partial growth arrest: physis, fat, or silastic? J Pediatr Orthop. 1993; 13:368–372.
3. Langenskiöld A. An operation for partial closure of an epiphysial plate in children, and its experimental basis. J Bone Joint Surg Br. 1975; 57:325–330.


4. Broughton NS, Dickens DR, Cole WG, Menelaus MB. Epiphyseolysis for partial growth plate arrest. Results after four years or at maturity. J Bone Joint Surg Br. 1989; 71:13–16.


5. Williamson RV, Staheli LT. Partial physeal growth arrest: treatment by bridge resection and fat interposition. J Pediatr Orthop. 1990; 10:769–776.
6. Foster BK, John B, Hasler C. Free fat interpositional graft in acute physeal injuries: the anticipatory Langenskiöld procedure. J Pediatr Orthop. 2000; 20:282–285.


7. Bright RW. Operative correction of partial epiphyseal plate closure by osseous-bridge resection and silicone-rubber implant. An experimental study in dogs. J Bone Joint Surg Am. 1974; 56:655–664.


8. Macksoud WS, Bright R. Bar resection and silastic interposition in distal radial physeal arrest. Orthop Trans. 1989; 13:1–2.
9. Olin A, Creasman C, Shapiro F. Free physeal transplantation in the rabbit. An experimental approach to focal lesions. J Bone Joint Surg Am. 1984; 66:7–20.


10. Bowen CV, Ethridge CP, O'Brien BM, Frykman GK, Gumley GJ. Experimental microvascular growth plate transfers. Part I--Investigation of vascularity. J Bone Joint Surg Br. 1988; 70:305–310.


11. Foster BK, Hansen AL, Gibson GJ, Hopwood JJ, Binns GF, Wiebkin OW. Reimplantation of growth plate chondrocytes into growth plate defects in sheep. J Orthop Res. 1990; 8:555–564.


12. Tobita M, Ochi M, Uchio Y, Mori R, Iwasa J, Katsube K, et al. Treatment of growth plate injury with autogenous chondrocytes: a study in rabbits. Acta Orthop Scand. 2002; 73:352–358.
13. Chung R, Foster BK, Xian CJ. Preclinical studies on mesenchymal stem cell-based therapy for growth plate cartilage injury repair. Stem Cells Int. 2011; 2011:570125.


14. Campbell CJ, Grisolia A, Zanconato G. The effects produced in the cartilaginous epiphyseal plate of immature dogs by experimental surgical traumata. J Bone Joint Surg Am. 1959; 41-A:1221–1242.


15. Kraft JJ, Jeong C, Novotny JE, Seacrist T, Chan G, Domzalski M, et al. Effects of hydrostatic loading on a self-aggregating, suspension culture-derived cartilage tissue analog. Cartilage. 2011; 2:254–264.


16. Novotny JE, Turka CM, Jeong C, Wheaton AJ, Li C, Presedo A, et al. Biomechanical and magnetic resonance characteristics of a cartilage-like equivalent generated in a suspension culture. Tissue Eng. 2006; 12:2755–2764.


17. Mohanraj B, Farran AJ, Mauck RL, Dodge GR. Time-dependent functional maturation of scaffold-free cartilage tissue analogs. J Biomech. 2014; 47:2137–2142.


18. Estrada LE, Dodge GR, Richardson DW, Farole A, Jimenez SA. Characterization of a biomaterial with cartilage-like properties expressing type X collagen generated in vitro using neonatal porcine articular and growth plate chondrocytes. Osteoarthritis Cartilage. 2001; 9:169–177.


19. Yoshida K, Higuchi C, Nakura A, Nakamura N, Yoshikawa H. Treatment of partial growth arrest using an in vitro-generated scaffold-free tissue-engineered construct derived from rabbit synovial mesenchymal stem cells. J Pediatr Orthop. 2012; 32:314–321.


20. Paley D, Herzenberg JE, Tetsworth K, McKie J, Bhave A. Deformity planning for frontal and sagittal plane corrective osteotomies. Orthop Clin North Am. 1994; 25:425–465.


21. Lennox DW, Goldner RD, Sussman MD. Cartilage as an interposition material to prevent transphyseal bone bridge formation: an experimental model. J Pediatr Orthop. 1983; 3:207–210.


22. Lee EH, Chen F, Chan J, Bose K. Treatment of growth arrest by transfer of cultured chondrocytes into physeal defects. J Pediatr Orthop. 1998; 18:155–160.


23. Kawabe N, Ehrlich MG, Mankin HJ. Growth plate reconstruction using chondrocyte allograft transplants. J Pediatr Orthop. 1987; 7:381–388.


24. Wakitani S, Kimura T, Hirooka A, Ochi T, Yoneda M, Yasui N, et al. Repair of rabbit articular surfaces with allograft chondrocytes embedded in collagen gel. J Bone Joint Surg Br. 1989; 71:74–80.


25. Steinwachs M. New technique for cell-seeded collagen-matrix-supported autologous chondrocyte transplantation. Arthroscopy. 2009; 25:208–211.


26. O'Grady JE, Bordon DM. Global regulatory registration requirements for collagen-based combination products: points to consider. Adv Drug Deliv Rev. 2003; 55:1699–1721.
27. Reginato AM, Iozzo RV, Jimenez SA. Formation of nodular structures resembling mature articular cartilage in long-term primary cultures of human fetal epiphyseal chondrocytes on a hydrogel substrate. Arthritis Rheum. 1994; 37:1338–1349.


28. Ebihara G, Sato M, Yamato M, Mitani G, Kutsuna T, Nagai T, et al. Cartilage repair in transplanted scaffold-free chondrocyte sheets using a minipig model. Biomaterials. 2012; 33:3846–3851.


29. Planka L, Srnec R, Rauser P, Stary D, Filova E, Jancar J, et al. Nanotechnology and mesenchymal stem cells with chondrocytes in prevention of partial growth plate arrest in pigs. Biomed Pap Med Fac Univ Palacky Olomouc Czech Repub. 2012; 156:128–134.


30. Tobita M, Ochi M, Uchio Y, Mori R, Iwasa J, Katsube K, et al. Treatment of growth plate injury with autogenous chondrocytes: a study in rabbits. Acta Orthop Scand. 2002; 73:352–358.