Abstract
Purpose
The aim of this study is to evaluate the effect of dexmedetomidine on corrected QT (QTc) and Tp-e intervals in patients undergoing spinal anesthesia.
Materials and Methods
We studied 50 patients who were scheduled to undergo spinal anesthesia before orthopedic surgeries. Patients were allocated to receive either an infusion of dexmedetomidine or normal saline after spinal anesthesia.
Results
QTc intervals were significantly prolonged after spinal anesthesia, and the prolonged QTc interval returned to baseline values 10 minutes after either normal saline or dexmedetomidine administration in both groups. The QTc interval values after dexmedetomidine administration were significantly shorter compared to the QTc interval values just before dexmedetomidine administration.
Sedation provides a comfortable and relaxed condition for patients undergoing regional anesthesia and reduces the anxiety of surgery by preventing unpleasant memories. To produce these effects, dexmedetomidine, which has sedative, analgesic, and sympatholytic effects, has been widely used. There is some concern that dexmedetomidine might cause circulatory complications, including hypertension, hypotension, and bradycardia.1 However, there are few reports about its effect on cardiac electrophysiology, and the findings from these reports are controversial.2-4 The corrected QT (QTc) interval prolongation is frequently caused by drugs which block the rapid and slow components of the potassium-delayed rectifying current.5,6 The interaction of dexmedetomidine with alpha-2-adrenoreceptors results in an alteration in ion channel conductance and decreased neuronal activation,7,8 which can alter cardiac electrophysiology.
The aim of this study was to examine the effect of dexmedetomidine on QTc interval and the interval from the peak of the T wave to the end of the T wave (Tp-e) in patients undergoing spinal anesthesia for elective orthopedic surgeries.
After obtaining approval from the Yonsei University College of Medicine Institutional Review Board and written informed consent from all participants, 50 patients (age 20 to 60 years, American Society of Anesthesiologists physical status I) who were scheduled for orthopedic surgery and spinal anesthesia were enrolled. We excluded patients with a preoperative QTc interval value >440 ms, preoperative electrocardiogram (ECG) abnormalities of conductivity or arrhythmias, a history of heart disease or circulatory insufficiency, any preoperative electrolyte abnormalities or medications known to prolong the QTc interval, of if they required general anesthesia due to incomplete block or high level of spinal anesthesia.
All study data were collected in the morning (8:30 AM to 11:30 AM) to avoid the effects of day-night changes on the QTc interval. No premedication was administered. After patients were taken into the operating room, pulse oximetry and non-invasive blood pressure monitoring were established. Data from a continuous ECG via lead II were collected using LabChart® software (Version 6: AD Instruments, Colorado Springs, CO, USA) and a data acquisition system (PowerLab®; AD Instruments) by a single author. For each patient, the data were saved in a separate file and coded using the patient enrollment number. The QT interval from the onset of the QRS complex to the end of the T wave and Tp-e interval from the peak of the T wave to the end of the T wave were measured automatically. If the automatic measurement did not correctly detect the earliest onset of the QRS complex or the end of the T wave, the QT and Tp-e intervals were measured manually, using the cursor from the earliest onset of the QRS complex to the latest end of the T wave, where its terminal limb joined the baseline.9 The QT interval and Tp-e interval were measured by a different author who was blinded to the group allocation of each patient. Each recorded measurement of QT and Tp-e interval was averaged from four successive beats. Heart rate (HR) correction was performed according to the Fridericia formula: QTc=QT3√(R-R interval).10
After obtaining the baseline ECG, an intravenous preload of 300 mL to 400 mL 0.9% normal saline or Hartmann's solution was infused. Spinal anesthesia was performed at the level of L3-4 or L4-5 interspace in the lateral decubitus position, using a 22G or 25G spinal needle. After identification of the subarachnoid space (outflow of the cerebrospinal fluid), 8 mg to 13 mg 0.5% hyperbaric bupivacaine solution (Marcaine Spinal 0.5% Heavy; AstraZeneca, Södertälje, Sweden) was administered. Immediately after drug injection, the patient was placed in a supine position. We recorded the upper sensory block level at 15 minutes after bupivacaine injection using a thermal test.
Participants were split into two groups: Group C (control, n=25) and Group D (dexmedetomidine, n=25). Patients who did not want to sleep were allocated to Group C, and those who wanted to sleep to Group D. Immediately after assessment of the sensory block level, 0.6 µg/kg of dexmedetomidine was administered intravenously over a 10 min period, followed by a continuous infusion at a dose of 0.4 µg/kg/hr in Group D. In Group C, volume-matched normal saline infusion was administered as a placebo. The ECG was continuously recorded during the entire study period. A decrease in mean arterial pressure (MAP) greater than 20% after administration of spinal anesthesia was defined as hypotension. Hypotension was initially treated with fluid resuscitation and, if the MAP did not respond, 4-8 mg IV ephedrine was administered. Bradycardia (<45 beats per minute, bpm) was treated with 0.25-0.5 mg atropine. Time points for the ECG analysis were: baseline, 5, 10, and 15 minutes after spinal anesthesia and 10, 40, and 70 minutes after infusion of dexmedetomidine or normal saline. The HR and MAP were recorded at the same time as the ECG measurements. The infusion of dexmedetomidine or normal saline continued until the surgery was complete.
Statistical analyses were performed using SPSS version 20 (SPSS Inc., Chicago, IL, USA) for Windows. All values were expressed as mean (SD). Sample size was calculated with 80% power (p=0.05, β=0.2) to detect a mean difference of 20 ms in the QTc interval between baseline and after spinal block and a standard deviation of 23 ms with a paired t-test. Using this formula, we required a minimum sample size of 21 patients per group. Demographic data were analyzed using the chi-squared test or Student's t-test, as appropriate. Student's t-tests were used to compare the baseline values of the QTc, Tp-e, HR, and MAP between the two groups. The differences within groups were analyzed using repeated measures ANOVA, and post hoc Bonferroni correction was applied where multiple comparisons were made. A value of p<0.05 was considered statistically significant.
Fifty patients were recruited to the study (27 male, 23 female). One patient in Group C and two patients in Group D were excluded from the final analysis to avoid the confounding effects of other agents because 0.25-0.5 mg atropine was administered due to bradycardia (<45 bpm). Patient characteristics in Group C and D are shown in Table 1, and were similar for the two groups.
All patients had normal baseline ranges of HR, MAP, and QTc intervals at rest, and there were no statistically significant differences in baseline HR, MAP, QTc, and Tp-e value between the two groups. In Group C, the QTc interval was significantly prolonged at 10 and 15 minutes after induction of spinal anesthesia compared to baseline value (p<0.05). There was no difference in QTc interval at 10, 40, and 70 minutes after administration of placebo (normal saline) compared to either the baseline QTc value or the QTc value at 15 minutes after spinal anesthesia. In Group D, the QTc interval was significantly prolonged at 5, 10, and 15 minutes (p<0.05, 0.01 and 0.05, respectively) after spinal anesthesia compared to the baseline value. The values of QTc interval at 10, 40, and 70 minutes (p<0.001, 0.05 and 0.001, respectively) after dexmedetomidine administration were significantly shortened when compared to the QTc interval value at 15 minutes after spinal anesthesia (just before dexmedetomidine administration), but those QTc values were not different from the baseline value in Group D. No significant changes in the Tp-e interval from baseline were observed in either group at any measurement time (Table 2). There was no significant change of MAP in either group among patients, who did not require administration of ephedrine (Fig. 1). The HR did not change during the study period in Group C, but the HR at 10, 40, 70 minutes after administration of dexmedetomidine was significantly decreased compared to the baseline value (p<0.01, 0.001, and 0.001, respectively) and the value at 15 minutes after spinal anesthesia (p<0.05, 0.01, and 0.01, respectively) in Group D (Fig. 2). We did not observe any complications, such as significant changes in MAP or arrhythmias, in either group in the post-anesthetic care unit.
We observed that spinal anesthesia provoked QTc interval prolongation in patients without cardiovascular disorders, and that the prolonged QTc interval returned to baseline values after 15 min following spinal anesthesia. Administration of dexmedetomidine promoted the return of the prolonged QTc interval that was induced by spinal blockade and did not modify the Tp-e interval.
Although there have been few reports about the effect of spinal anesthesia on cardiac electrophysiology, Owczuk, et al.11 reported that spinal anesthesia induced significant QTc interval prolongation in healthy patients, which is in accordance with our results. Although the mechanism is still unclear, prolongation of the QTc interval may be attributed to sympathetic stimulation rather than the drug itself for regional anesthesia.12,13 It is well recognized that sympathetic activation provokes changes in cardiac repolarization14 and repolarization abnormalities.15 Therefore, the agents including opioids or β-adrenergic blockers, which can reduce the sympathetic stimulation, are considered for patients who are vulnerable to QTc interval prolongation.16,17 Our patients were not premedicated before spinal anesthesia for fear of a potential influence of premedication agents on cardiac electrophysiology. Therefore, the absence of premedication might lead to the activation of the sympathetic nervous system. Another possible reason for QTc interval prolongation during spinal anesthesia is that it is a compensatory mechanism of thoracic sympathetic stimulation by cardiac accelerating nerve fibers (T1-T4), which were preserved after spinal blockade.18,19 The QTc interval was maximally prolonged at 10 min after spinal blockade in both groups, and the prolonged average value of QTc interval value was about 11 ms and 14 ms in Group C and Group D, respectively. The degree of the QTc interval may not be clinically harmful to healthy patients because none of the enrolled patients showed a QTc interval over 440 ms, and the extent of QTc interval prolongation was less than 20 ms, which was regarded as a prolongation of the QTc interval.5,6 Additionally, the Tp-e interval, which is considered to be a more reliable predictor of risk for torsades de pointes, was not modified at any time after spinal anesthesia compared to baseline values in either group. The Tp-e interval is a surface ECG marker of transmural dispersion of repolarization across the myocardial wall, and its exaggeration increases the risk of torsades de pointes.20 Our findings support the rationale that spinal anesthesia is safe despite a prolonged QTc interval in clinical fields.
Dexmedetomidine increased the dysrhythmogenic threshold of epinephrine during halothane anesthesia in canines2 and showed an inhibitory effect on ventricular tachyarrhythmia in a rabbit model of acquired long QT syndrome.3 On the contrary, Hammer, et al.4 showed that dexmedetomidine significantly prolonged QTc intervals in pediatric patients, which contrasts with our results. It is unclear why previous results are inconsistent with our findings, but in the latter study, concurrent administration of drugs, such as propofol and ketamine, and the different disease statuses of patients with cardiac conduction abnormalities might complicate the interpretation of the pure effect of dexmedetomidine on QTc. Moreover, the administered drug doses may have some influence on the degree of QTc interval prolongation.21,22 Our administered dose (0.6 µg/kg over 10 minutes followed by 0.4 µg/kg/hr) was lower than the doses reported by Hammer, et al.4 (1.0 µg/kg over 10 minutes followed by 0.7 µg/kg each hour). However, our doses may be more clinically applicable during spinal anesthesia because large doses of dexmedetomidine lead to severe bradycardia that require intervention and, hence, raise safety concerns.23 Further study of a dose-response relationship between dexmedetomidine and QTc interval are needed in the future.
The prolongation of the QTc interval persisted for 15 minutes after spinal blockade, and then the prolonged QTc interval returned to the baseline value in both groups. Infusion of dexmedetomidine significantly shortened the QTc interval compared to the QTc interval at just before administration of dexmedetomidine. Our findings suggest that dexmedetomidine could accelerate the normalization of a prolonged QTc interval that was induced by spinal anesthesia or might shorten the value of the baseline QTc interval in cases where patients did not have a central blockade. It is not easy to infer the mechanism by which dexmedetomidine shortens the spinal anesthesia-induced prolonged QTc interval, but it is probably associated with decreasing sympathetic activity. Alpha-2-adrenoreceptor is distributed throughout the brain stem, peripheral vasculature, and presympathetic nervous terminal as an auto-suppressive receptor. The receptor suppresses its own adrenal release from the terminal reticulum to the effecter, including the brain system, vasculature, and the heart, achieving sedation, analgesia, decreased blood pressure and bradycardia.3 Alpha-2-adrenoreceptor agonist modifies the cardiac autonomic state not only by suppressing cardiac noradrenaline release, but also by activating vagal acetylcholine release.24,25 Therefore dexmedetomidine is probably preferable for vulnerable patients with a prolonged QTc interval, especially when acceleration of the sympathetic nervous system is anticipated in the operating room.
A limitation of our study was that the effects of spinal anesthesia on myocardial repolarization could not be separated from the effects of dexmedetomidine. However, the use of dexmedetomidine may be desirable for sedation during spinal anesthesia in patients at risk of long QT syndrome if bradycardia can be properly managed. When the HR is slow, diminished accumulation of slowly-activating delayed rectifier potassium currents prolongs the duration of the action potential.2,26 Therefore, a decreased HR may enhance cardiac susceptibility to torsades de pointes in patients with prolonged QT interval. Although dexmedetomidine has complex and counteracting cardiovascular effects, adverse effects such as bradycardia and hypotension are generally easily managed. Another limitation of this study is non-randomization in allocating patients. Randomization was not possible because this study was not done in volunteers and sedation was provided for the patients only who requested it before anesthesia. Hence, the baseline activity of sympathetic nervous system might be different between two groups since it was expected that the patients who wanted sleep would have a tendency to have more anxiety and higher sympathetic activity in comparison to the patients who did not want to sleep. Measurement of heart rate variability or plasma catecholamine would be useful for the assessment of baseline activity of sympathetic nervous system. However, we could indirectly assume that the sympathetic activities of two groups were similar since the baseline HR, MAP, and QTc intervals were comparable.
In conclusion, spinal anesthesia prolonged the QTc interval in healthy patients, but it did not increase the Tp-e interval, indicating low or no risk of torsades de pointes. Dexmedetomidine could promote the return to baseline of prolonged QTc intervals induced by spinal anesthesia. This suggests that dexmedetomidine could be useful for patients with prolongation of the QTc interval who are susceptible to the development of arrhythmia.
Figures and Tables
Fig. 1
Mean arterial pressure before anesthesia (baseline) and after spinal anesthesia (SA) and after administration of drug (normal saline in Group C and dexmedetomidine in Group D, respectively). Error bars are SD.
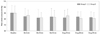
Fig. 2
Heart rate before anesthesia (baseline) and after spinal anesthesia (SA) and after administration of drug (normal saline in Group C and dexmedetomidine in Group D, respectively). *p<0.01 and †p<0.001 compared with the baseline value, ‡p<0.05 and §p<0.01 compared with the SA+15 min value. Errors bar are SD.
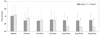
References
2. Hayashi Y, Sumikawa K, Maze M, Yamatodani A, Kamibayashi T, Kuro M, et al. Dexmedetomidine prevents epinephrine-induced arrhythmias through stimulation of central alpha 2 adrenoceptors in halothane-anesthetized dogs. Anesthesiology. 1991; 75:113–117.


3. Tsutsui K, Hayami N, Kunishima T, Sugiura A, Mikamo T, Kanamori K, et al. Dexmedetomidine and clonidine inhibit ventricular tachyarrhythmias in a rabbit model of acquired long QT syndrome. Circ J. 2012; 76:2343–2347.


4. Hammer GB, Drover DR, Cao H, Jackson E, Williams GD, Ramamoorthy C, et al. The effects of dexmedetomidine on cardiac electrophysiology in children. Anesth Analg. 2008; 106:79–83.


6. Booker PD, Whyte SD, Ladusans EJ. Long QT syndrome and anaesthesia. Br J Anaesth. 2003; 90:349–366.


7. Nacif-Coelho C, Correa-Sales C, Chang LL, Maze M. Perturbation of ion channel conductance alters the hypnotic response to the alpha 2-adrenergic agonist dexmedetomidine in the locus coeruleus of the rat. Anesthesiology. 1994; 81:1527–1534.


8. Sculptoreanu A, Scheuer T, Catterall WA. Voltage-dependent potentiation of L-type Ca2+ channels due to phosphorylation by cAMP-dependent protein kinase. Nature. 1993; 364:240–243.


9. Moss AJ. Measurement of the QT interval and the risk associated with QTc interval prolongation: a review. Am J Cardiol. 1993; 72:23B–25B.


10. Fridericia LS. The duration of systole in an electrocardiogram in normal humans and in patients with heart disease. 1920. Ann Noninvasive Electrocardiol. 2003; 8:343–351.


11. Owczuk R, Sawicka W, Wujtewicz MA, Kawecka A, Lasek J, Wujtewicz M. Influence of spinal anesthesia on corrected QT interval. Reg Anesth Pain Med. 2005; 30:548–552.


12. Stewart J, Kellett N, Castro D. The central nervous system and cardiovascular effects of levobupivacaine and ropivacaine in healthy volunteers. Anesth Analg. 2003; 97:412–416.


13. Knudsen K, Beckman Suurküla M, Blomberg S, Sjövall J, Edvardsson N. Central nervous and cardiovascular effects of i.v infusions of ropivacaine, bupivacaine and placebo in volunteers. Br J Anaesth. 1997; 78:507–514.


14. Hung O. Understanding hemodynamic responses to tracheal intubation. Can J Anaesth. 2001; 48:723–726.


15. Haapalahti P, Viitasalo M, Perhonen M, Mäkijärvi M, Väänänen H, Oikarinen L, et al. Electrocardiographic interventricular dispersion of repolarization during autonomic adaptation in LQT1 subtype of long QT syndrome. Scand Cardiovasc J. 2008; 42:130–136.


16. Lindgren L, Rautiainen P, Klemola UM, Saarnivaara L. Haemodynamic responses and prolongation of QT interval of ECG after suxamethonium-facilitated intubation during anaesthetic induction in children: a dose-related attenuation by alfentanil. Acta Anaesthesiol Scand. 1991; 35:355–358.


17. Kweon TD, Nam SB, Chang CH, Kim MS, Lee JS, Shin CS, et al. The effect of bolus administration of remifentanil on QTc interval during induction of sevoflurane anaesthesia. Anaesthesia. 2008; 63:347–351.


18. Veering BT, Cousins MJ. Cardiovascular and pulmonary effects of epidural anaesthesia. Anaesth Intensive Care. 2000; 28:620–635.


19. Baron JF, Payen D, Coriat P, Edouard A, Viars P. Forearm vascular tone and reactivity during lumbar epidural anesthesia. Anesth Analg. 1988; 67:1065–1070.


20. Antzelevitch C. Arrhythmogenic mechanisms of QT prolonging drugs: is QT prolongation really the problem? J Electrocardiol. 2004; 37:Suppl. 15–24.


21. Saarnivaara L, Hiller A, Oikkonen M. QT interval, heart rate and arterial pressures using propofol, thiopentone or methohexitone for induction of anaesthesia in children. Acta Anaesthesiol Scand. 1993; 37:419–423.


22. Han DW, Park K, Jang SB, Kern SE. Modeling the effect of sevoflurane on corrected QT prolongation: a pharmacodynamic analysis. Anesthesiology. 2010; 113:806–811.
23. Tan JA, Ho KM. Use of dexmedetomidine as a sedative and analgesic agent in critically ill adult patients: a meta-analysis. Intensive Care Med. 2010; 36:926–939.


24. Shimizu S, Akiyama T, Kawada T, Sata Y, Mizuno M, Kamiya A, et al. Medetomidine, an α(2)-adrenergic agonist, activates cardiac vagal nerve through modulation of baroreflex control. Circ J. 2012; 76:152–159.


25. Parent BA, Munoz R, Shiderly D, Chrysostomou C. Use of dexmedetomidine in sustained ventricular tachycardia. Anaesth Intensive Care. 2010; 38:781.