Abstract
Purpose
To investigate the general characteristics of glucose metabolism distribution and the functional deficit in the brain of children with developmental language delay (DLD), we compared functional neuroradiological studies such as positron emission tomography (PET) of a patient group of DLD children and a control group of attention-deficit hyperactivity disorder (ADHD) children.
Patients and Methods
Seventeen DLD children and 10 ADHD children under 10 years of age were recruited and divided into separate groups consisting of children less than 5 years of age or between 5 and 10 years of age. The PET findings of 4 DLD children and 6 control children whose ages ranged from 5 to 10 years were compared by Statistical Parametric Mapping (SPM) analysis.
Results
All of the DLD children revealed grossly normal findings in brain MRIs, however, 87.5% of them showed grossly abnormal findings in their PET studies. Abnormal findings were most frequent in the thalamus. The patient group showed significantly decreased glucose metabolism in both frontal, temporal and right parietal areas (p < 0.005) and significantly increased metabolism in both occipital areas (p < 0.05) as compared to the control group.
Conclusion
This study reveals that DLD children may show abnormal findings on functional neuroradiological studies, even though structural neuroradiological studies such as a brain MRI do not show any abnormal findings. Frequent abnormal findings on functional neuroradiological studies of DLD children, especially in the subcortical area, suggests that further research with quantitative assessments of functional neuroradiological studies recruiting more DLD children and age-matched normal controls could be helpful for understanding the pathophysiology of DLD and other disorders confined to the developmental disorder spectrum.
Language impairment in children is defined as any kind of difficulty in communication stemming from language delay or abnormal acquisition of language ability caused by a biophysiological deficit of the brain structures, which are responsible for language understanding and expression. Language delay could be either a simple case which has problems only in language territory, or a complicated case combined with problems in various territories, such as mental retardation, autistic disorder, motor delay, hearing defect or lack of environmental stimulation. Therefore, it is important for the optimal treatment of language delay and better prognosis to distinguish if language impairment is a simple or complicated case.
Developmental language disorder (DLD) is a common disorder estimated to occur in as many as 3-7% of otherwise normal children.1,2 This term is applied to children who have (1) significant deficit in language ability, (2) absence of other cognitive impairments (normal IQ, non verbal IQ > 85), (3) no hearing difficulty, (4) no neurological abnormalities or related medications regarding seizures, cerebral palsy or brain trauma, and (5) no impairment on phonological structures or no psychosocial relationship disorder.3 DLD often has a favorable result with spontaneous improvement without any treatment.4 On the other hand, frequent underestimation of its severity causes delay of treatment and worsens the disease course. Following difficulty in communication with peers finally results in a learning disorder or other psychosocial impairments.5 Therefore, it is necessary to have sufficient understanding of the pathophysiology of DLD in order to explain the importance of early diagnosis and treatment.
There are limited studies examining the underlying causes or related neurobiological deficits of DLD because many DLD children do not visit a hospital or do not continue to undergo treatment. Furthermore, a greater number of limitations exist due to difficulties related to ethical issues in performing functional neuroradiological studies in children. Therefore, this study was designed to investigate the general characteristics of glucose metabolism distribution and functional deficits in the brain of DLD children by comparing functional neuroradiological by positron emission tomography (PET) studies between the patient group of DLD children and the control group of attention-deficit hyperactivity disorder (ADHD) children.
The patient group consisted of 17 right-handed DLD children recruited among patients who visited outpatient clinics with the chief complaint of language delay and were diagnosed as DLD at Severance Hospital and Yongdong Severance Hospital, Yonsei University Medical Center, Seoul, Korea from 1999 to March 2006. All of the children underwent psychological evaluation, language evaluation and neuroradiological study (by PET and MRI). Eligibility criteria were normal school performance, normal neurological examination and normal developmental history. Exclusionary criteria included hearing or gross sensorimotor deficits, any neurologic disorders such as seizure, clinical evidence of progressive encephalopathy, anticonvulsant or psychotropic medications, and overtly evident focal brain lesions, brain atrophy or ventriculomegaly.
Psychological evaluations with Korean-Wechsler Preschool and Primary Scales of Intelligence (K-WPPSI) or Korean Educational Developmental Institute-Wechsler Intelligence Scale of Children (KEDI-WISC) indicated general mental and social development within normal limits. Subjects showed developmental delay only in language territory, not in cognition, motor or social territories. Patients with a nonverbal IQ of less than 85 in KEDI-WISC were excluded.
Language ability was tested using the Peabody Picture Vocabulary Test (PPVT), Receptive-Expressive Emergent Language Scale (REEL), Korean-Northwestern Syntax Screening Test (K-NSST), Sequenced Language Scale for Infants (SELSI) and Preschool Receptive-Expressive Language Scale (PRES). DLD classification required relative but significant deficiency in language measures, in other words, either a mean length of utterance score that is 2 standard deviations below the mean for the child's chronological age, or a 1 year delay below the child's chronological age. Receptive language quotient (receptive language age divided by chronological age) and expressive language quotient (expressive language age divided by chronological age) were used to compare language delay among subjects.
The control group consisted of 10 right-handed ADHD children recruited among children who were on psychiatric treatment under the diagnosis of ADHD at Severance Hospital and Yongdong Severance Hospital, Yonsei University Medical Center, Seoul, Korea from 1999 to March 2006. All of the children underwent neuroradiological study (by PET and MRI). Subject eligibility required normal developmental history. Exclusionary criteria included language delay and psychotropic medications.
Visual interpretation of MRI and PET images was done by radiologists who did not have any clinical information regarding the subjects. Focal or diffuse functional abnormalities of glucose metabolism were found in F-18 FDG PET results, which were constructed as coronal, sagittal and axial planes.
The results from 18F-FDG-PET measurements at rest in 2 groups of DLD and ADHD children were compared by Statistical Parametric Mapping (SPM) analysis. For this analysis the subjects were limited to children whose age ranged from 5 to 10 years and who underwent a PET study at Severance Hospital to prevent bias resulting from age differences or non-identical PET machinery.
Spatial pre-processing and statistical analysis of 18F-FFMZ-PET were performed using Statistical Parametric Mapping (SPM2, Institute of Neurology, University College of London, UK). All 18F- FDG-PET images were normalized into a group 18F-FDG-PET template to remove the inter-subject anatomical variability. The 18F-FDG-PET template of both the DLD and ADHD group was spatially normalized into the MNI (Montreal Neurological Institute, McGill University, CA, USA) standard 18F-FDG-PET template with nonlinear transformation. Spatially normalized images were smoothed by convolution with an isotropic Gaussian kernel with 8mm FWHM to increase the signal-to-noise ratio and accommodate the variations in subtle anatomical structures. The effects of global uptake were removed by normalizing the count of each voxel to the mean count of the brain (proportional scaling in SPM). A significant decrease and increase of the adjusted regional uptake were obtained using t-statistics at every voxel from both DLD and ADHD controls. The t-values were transformed to Z-scores in the standard Gaussian distribution. The clusters consisting of a minimum of 50 contiguous voxels with the threshold of uncorrected p < 0.05 for increased uptake sites and p < 0.005 for decreased uptake sites were considered statistically significant.
The patient group consisted of 17 DLD children including 15 boys (83.3%), aged 2.3-8.6 years (mean age: 4.7 ± 2.1 years), 10 children under 5 years and 7 children over 5 years. The control group consisted of 10 ADHD children including 9 boys (90.0%), aged 3.2-8.7 years (mean age: 7.0 ± 1.8 years), 2 children under 5 years and 8 children over 5 years.
The mean receptive language quotient of the patient group was 57.8 ± 22.7, and the mean expressive language quotient of the patient group was 53.0 ± 19.4. Sixteen DLD children (94.1%) had mixed receptive-expressive impairment, and 1 DLD child (5.9%) had expressive impairment.
Eight DLD children who underwent MRIs had normal results. However, 87.5% of the children showed grossly abnormal PET findings. Patient group 1 consisting of 7 children over 5 years of age revealed grossly abnormal PET findings in 6 cases (85.7%). Abnormal PET findings were shown most frequently in the thalamus, followed by cerebellum, basal ganglia, caudate nucleus and the left temporal lobe (Table 1).
The patient group 2 consisting of 10 children aged less than 5 years, revealed grossly abnormal PET findings in 7 cases (70.0%). Abnormal PET findings were shown most frequently in the thalamus, followed by the basal ganglia, cerebellum, frontal lobe and caudate nucleus (Table 2).
The PET findings of 4 DLD children and 6 ADHD children whose age ranged from 5 to 10 years were compared by SPM analysis. The patient group showed significantly decreased glucose metabolism in both frontal, temporal and right parietal areas (p < 0.005, Fig. 1) and significantly increased glucose metabolism in both occipital areas (p < 0.05, Fig. 2).
Recently, the development in functional neuroimaging techniques such as single photon emission computed tomography (SPECT) and PET allows unique approaches to study neuronal networks engaged in language processing.6-9 This contributes to the accumulation of basic knowledge of the developing brain and the pathophysiology of developmental disorders since structural neuroradiological studies such as brain MRI have not resulted in thorough information. As previous studies revealed, many DLD children have functional abnormalities in their PET or SPECT studies without any structural abnormality in their brain MRI. Therefore, functional neuroradiological studies are expected to provide more clinically and academically valuable information. However, currently there are limited reports on the subject of functional neuroimaging studies despite its necessity.10-17
Establishing quantitative indices of the developmental brain is important because the focal brain functions of children whose brains develop rapidly are very different from the adult brain.18-20 Large studies recruiting healthy children to get normal indices for functional neuroimaging studies are not easy to perform due to ethical issues. There are only a few studies with a small number of normal subjects,21 or the use of a control group of children with other disorders, such as ADHD.22 For this reason we chose to have control subjects with ADHD children as well.
More fundamental studies to discover the relationship between neurobiological structures and language development are needed. Research within the last decade indicate a weakened asymmetry in Broca's area of specific language impairment (SLI) children,11abnormal right to left symmetry of cerebral blood flow in SLI children, and decreased cerebral blood flow in the right parietal lobe and subcortical areas of SLI as compared to ADHD children.22 There are several disputes with these studies regarding their explanation of the pathophysiology of DLD. Locke et al.23 explained that the pathophysiology was due to compensated overgrowth of the right hemisphere in circumstances where the left hemisphere is not allowed to develop normally. Gauger and colleagues12 claimed an abnormal proportion of the brain hemisphere does not result from overgrowth of the right hemisphere but rather from the decreased growth of both hemispheres. On the other hand, Galaburda et al.24 reported on the abnormal neurological pathway responsible for language development, and Leonard and colleagues25 discussed the abnormal cell migration by hereditary background.
As mentioned above, the reported findings are currently inconclusive. Further more, previous reports have potential limitations in study design. Park et al.7 concluded that neuroradiological studies cannot replace an early evaluation of language ability. However, there was no quantitative analysis of SPECT findings in that report, and the subject group was too small to give any significance to the functional neuroimaging study in DLD. Kim's study,26 which revealed decreased cerebral blood flow in the thalamus and parietal areas of SLI children, also has limitations such as no quantitative analysis but a superficial analysis and no consideration of the characteristics of the developing brain. Hwang and colleagues27 reported decreased blood flow in the basal ganglia and right parietal area of DLD children but without consideration of age differences between the control and patient group.
The developing brain has different neuroradiological findings from the adult brain, for an active metabolic lesion continues to change during developmental periods. Absolute glucose metabolism of the neonate is as low as 30% of an adult's, continues to increase until 4 years of age when it is twice that of adult's, begins to decrease by 10 years of age, and becomes similar to adult's at 16-18 years of age.28 Muzik29 reported that children who are older than 6 years have a similar pattern of glucose metabolism with that of the adult brain. Therefore, analysis of neuroradiological studies without consideration of age differences could result in potential errors. In the present study, only 4 of 17 DLD children were included in SPM analysis because of age differences between the patient and control group. Usually, DLD children visit a hospital at a younger age than ADHD children. Therefore, regarding age differences, we selected age-matched patients and control subjects for the SPM analysis. This is the first study involving age considerations and quantitative analysis of functional neuroimaging studies by PET of DLD children, although it has a limitation due to the small number of study subjects. In addition, this is the first study, which revealed decreased glucose metabolism not only in the left hemisphere but also in the right frontal and temporal areas of DLD children.
The thalamus is an input modulator where external sensory stimuli enter the cortex of the brain. Apart from this study, decreased glucose metabolism in the thalamus is often observed in other studies regarding the developmental disorder spectrum.7,17,30,31 Normally, cerebral blood flow of the developing brain reaches its peak at 2-4 years32 of age, and the blood flow of the thalamus is significantly increased in the cerebral hemisphere as compared to other areas until 2 months after birth.33 From these reports, it can be concluded that the thalamus plays an important role in brain development, especially in the early period. The present result of widely decreased glucose metabolism in both frontal and temporal lobes of DLD children is relevant with respect to Gauger and colleagues' explanation that the underlying cause of DLD is decreased development in both sides of the cerebral hemisphere and not only in one side.12 There are some problems at the beginning period of brain development that can be presumed, such as a congenital factor or a functional deficit in the subcortical areas including the thalamus, which can widely influence brain development and result in various developmental disorders.14 Therefore, in future research related to the developmental disorder spectrum, the importance of subcortical areas including the thalamus should be recognized.22
Contrary to our expectations and previous reports, glucose metabolism of the subcortical and cerebellum area of DLD children was not decreased in this study when compared to the ADHD controls. This effect might be explained by the use of ADHD children as the control group, as they might also have abnormal cerebral blood flow in the thalamus, basal ganglia and cerebellum.21,34 Decreased glucose metabolism in the right parietal area of DLD children as compared to ADHD children is relevant in regards to a previous report.22 Because this study compared the glucose metabolism between two groups, abnormal metabolic pattern of ADHD children could affect the result. Possibly increased glucose metabolism in the parietal areas of ADHD children34 could affect the relatively decreased metabolism in the parietal area observed in the DLD children. Increased glucose metabolism in both occipital areas of the patient group could be explained as a result of overactive metabolic compensation in an environment where both frontal and temporal lobes are not normally activated. Although statistically different, a bias could be present from the abnormal control group or from the small size of the subject study group, which may not represent the true characteristics of DLD children. Therefore, further studies recruiting a greater number of DLD children and different control groups such as those without deficits or developmental disorders are needed.
Although this study has some limitations, such as a small subject study group size and the choice of ADHD children as the control group, it is useful since it is the first research with DLD children that considers age differences and compares functional neuroradiological images by quantification. Broader research with additional patient groups is necessary for a clearer understanding of normal language development and DLD. Further research revealing structural microabnormality and neurobiological deficits through quantitative structural and functional neuroradiological studies will play an important role in treating not only DLD but also other diseases confined to the developmental disorder spectrum.
Figures and Tables
Fig. 1
Brain areas with significantly decreased glucose metabolism in DLD children as compared to ADHD controls (p < 0.005).
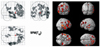
Fig. 2
Brain areas with significantly increased glucose metabolism in DLD children as compared to ADHD controls (p < 0.05).
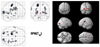
References
1. Tomblin JB, Records NL, Buckwalter P, Zhang X, Smith E, O'Brien M. Prevalence of specific language impairment in kindergarten children. J Speech Lang Hear Res. 1997. 40:1245–1260.


2. Whitehurst GJ, Fischel JE. Practitioner review: early developmental language delay: what, if anything, should the clinician do about it? J Child Psychol Psychiatry. 1994. 35:613–648.


3. Leonard LB. Children with specific language impairment. 1988. Cambridge, MA: MIT Press.
4. Bishop DV, Edmundson A. Language-impaired 4-year-olds: distinguishing transient from persistent impairment. J Speech Hear Disord. 1987. 52:156–173.
5. Silva PA. The prevalence, stability and significance of developmental language delay in preschool children. Dev Med Child Neurol. 1980. 22:768–777.


6. Moon JL, Lee BN, Shin JE, Song DH, Kim EN. Tc-99m-ECD brain single photon emission computed tomography findings in cerebral palsy: comparison with magnetic resonance imaging findings. J Korean Acad Rehabil Med. 2003. 27:868–874.
7. Park ES, Park CI, Chang JC, Shin JC, Park JE. Comparison of speech-language, mental-motor development and brain radiologic findings in children with cerebral palsy and other delayed development. J Korean Acad Rehabil Med. 1999. 23:918–925.
8. Lee ZI, Park SM, Ahn SH, Jang SH, Son SM, Byun WM. Evidence of microstructural abnormality on descending motor pathway in cerebral palsied children with periventricular leukomalacia: diffusion tensor MRI study. J Korean Acad Rehabil Med. 2003. 27:340–343.
9. Nakagawa T, Murata Y, Kojima T, Shinkai Y, Yamaya Y, Kato M, et al. Prognostic value of brain perfusion single-photon emission computed tomography (SPECT) for language recovery in patients with aphasia. Nucl Med Commun. 2005. 26:919–923.


10. Balsamo LM, Xu B, Grandin CB, Petrella JR, Braniecki SH, Elliott TK, et al. A functional magnetic resonance imaging study of left hemisphere language dominance in children. Arch Neurol. 2002. 59:1168–1174.


11. De Fosse L, Hodge SM, Makris N, Kennedy DN, Caviness VS Jr, McGrath L, et al. Language-association cortex asymmetry in autism and specific language impairment. Ann Neurol. 2004. 56:757–766.


12. Gauger LM, Lombardino LJ, Leonard CM. Brain morphology in children with specific language impairment. J Speech Lang Hear Res. 1997. 40:1272–1284.


13. Herbert MR, Ziegler DA, Deutsch CK, O'Brien LM, Kennedy DN, Filipek PA, et al. Brain asymmetries in autism and developmental language disorder: a nested whole-brain analysis. Brain. 2005. 128:213–226.


14. Hugdahl K, Gundersen H, Brekke C, Thomsen T, Rimol LM, Ersland L, et al. FMRI brain activation in a finnish family with specific language impairment compared with a normal control group. J Speech Lang Hear Res. 2004. 47:162–172.


15. Liegeois F, Connelly A, Cross JH, Boyd SG, Gadian DG, Vargha-Khadem F, et al. Language reorganization in children with early-onset lesions of the left hemisphere: an fMRI study. Brain. 2004. 127:1229–1236.


16. Plante E, Swisher L, Vance R, Rapcsak S. MRI findings in boys with specific language impairment. Brain Lang. 1991. 41:52–66.
17. Lee BF, Yang P, Jong YJ, Hsu HY, Chen CC. Single photon emission computerized tomography in children with developmental language disorder-a preliminary report. Kaohsiung J Med Sci. 2002. 18:373–378.
18. Kim SE, Lee DS, Chung JK, Lee MC, Koh CS, Cho SC, et al. Regional distribution of cerebral blood flow in childhood measured by 99mTc-HMPAO SPECT reference values of semiquantitative indices and effect of age. Korean J Nucl Med. 1991. 25:6–16.
19. Chugani HT, Phelps ME. Maturational changes in cerebral function in infants determined by 18FDG positron emission tomography. Science. 1986. 231:840–843.


20. Chugani HT, Phelps ME, Mazziotta JC. Positron emission tomography study of human brain functional development. Ann Neurol. 1987. 22:487–497.


21. Kaya GC, Pekcanlar A, Bekis R, Ada E, Miral S, Emiroglu N, et al. Technetium-99m HMPAO brain SPECT in children with attention deficit hyperactivity disorder. Ann Nucl Med. 2002. 16:527–531.


22. Ors M, Ryding E, Lindgren M, Gustafsson P, Blennow G, Rosen I. SPECT findings in children with specific language impairment. Cortex. 2005. 41:316–326.


23. Locke JL. Gradual emergence of developmental language disorder. J Speech Hear Res. 1994. 37:608–616.
24. Galaburda AM. Neuroanatomic basis of developmental dyslexia. Neurol Clin. 1993. 11:161–173.
25. Leonard CM, Voeller KK, Lombardino LJ, Morris MK, Hynd GW, Alexander AW, et al. Anomalous cerebral structure in dyslexia revealed with magnetic resonance imaging. Arch Neurol. 1993. 50:461–469.


26. Kim SW, Shin JB, You S, Yang EJ, Lee SK, Chung HJ, et al. Diagnosis and clinical features of children with language delay. J Korean Acad Rehabil Med. 2005. 29:584–590.
27. Hwang JW, Lee JB, Kim BN, Lee HY, Lee DS, Shin MS, et al. Regional cerebral perfusion abnormalities in developmental language disorder: Statistical parametric mapping analysis. Eur Arch Psychiatry Clin Neurosci. 2006. 256:131–137.


28. Chugani HT. A critical period of brain development: studies of cerebral glucose utilization with PET. Prev Med. 1998. 27:184–188.


29. Muzik O, Chugani DC, Juhasz C, Shen C, Chugani HT. Statistical parametric mapping: assessment of application in children. Neuroimage. 2000. 12:538–549.


30. Park CI, Kim SW, Kim YC, Shin JC, Lee JD. Brain MRI and SPECT findings in children with cerebral palsy. J Korean Acad Rehabil Med. 1997. 21:1060–1067.
31. Lee JD, Kim DI, Ryu YH, Whang GJ, Park CI, Kim DG. Technetium-99m-EDC brain SPECT in cerebral palsy: Comparison with MRI. J Nucl Med. 1998. 39:619–623.
32. Chiron C, Raynaud C, Maziere B, Zilbovicius M, Laflamme L, Masure MC, et al. Changes in regional cerebral blood flow during brain maturation in children and adolescents. J Nucl Med. 1992. 33:696–703.
33. Rubinstein M, Denays R, Ham HR, Piepsz A, Van Pachterbeke T, Haumont D, et al. Functional imaging of brain maturation in humans using iodine-123 iodoamphetamine and SPECT. J Nucl Med. 1989. 30:1982–1985.
34. Kim BN, Lee JS, Shin MS, Cho SC, Lee DS. Regional cerebral perfusion abnormalities in attention deficit/hyperactivity disorder. Statistical parametric mapping analysis. Eur Arch Psychiatry Clin Neurosci. 2002. 252:219–225.