Abstract
Non-gastrointestinal stromal tumor (GIST) soft tissue sarcomas (STSs) are a heterogeneous group of neoplasms whose classification and management continues to evolve with better understanding of their biologic behavior. The 2013 World Health Organization (WHO) has revised their classification based on new immunohistochemical and cytogenetic data. In this article, we will provide a brief overview of the revised WHO classification of soft tissue tumors, discuss in detail the radiology and management of the two most common adult non-GIST STS, namely liposarcoma and leiomyosarcoma, and review some of the emerging histology-driven targeted therapies in non-GIST STS, focusing on the role of the radiologist.
Soft-tissue sarcomas (STSs) are rare mesenchymal neoplasms accounting for approximately 1% of all adult malignancies (12). The classification of STS and approach to treatment has evolved in recent years with increasing understanding of the molecular pathogenesis and complex cytogenetics of these tumors. Conventional histology and immunohistochemistry still remain the cornerstones for diagnosis, with cytogenetics being increasingly incorporated into the diagnostic work up. Similarly, treatment of advanced STS is shifting from generic “one-fits-all” 106cytotoxic chemotherapy to histology-driven therapy aimed at targeting specific mutations and signaling pathways unique to that subtype of sarcoma (34). The earliest and the most successful example was the discovery of kit mutations in gastrointestinal stromal tumor (GIST), and subsequent development of the selective tyrosine kinase inhibitor imatinib, which revolutionized the treatment of GIST (3). The role of imaging in GISTs and response assessment is elaborated in a separate article in this issue given its significance.
In this article, we will provide a brief overview of the revised World Health Organization (WHO) classification of soft tissue tumors, discuss in detail the radiology and management of the two most common adult non-GIST STS, namely liposarcoma and leiomyosarcoma, and review some of the emerging histology-driven targeted therapies in non-GIST STS, focusing on the role of the radiologist.
In 2013, based on the new immunohistochemical and cytogenetic data available, the WHO published its revised soft tissue tumor classification (5). As per the revised classification, depending on their biological behavior, soft tissue tumors are classified as benign (usually do not recur), intermediate-locally aggressive (often recur but do not metastasize), intermediate-rarely metastasizing (often recur and may metastasize in < 2% cases), or malignant (most common and have a high risk of metastases). Examples of these four are lipoma, well-differentiated liposarcoma, solitary fibrous tumor, and dedifferentiated liposarcoma, respectively (67).
The emphasis in the revised classification is on cytogenetics and molecular pathways, indicating the potential for treatment by molecular targeted therapies (MTTs) (67). Table 1 lists the molecular mechanisms for sarcomagenesis in a few important sarcomas. Based on cytogenetics, sarcomas tend to fall in either of two major subsets. One group is cytogenetically simple with a few chromosomal rearrangements leading to the formation of fusion proteins (891011). Examples of sarcomas in this group include synovial sarcoma with the t(X; 18) translocation resulting in SYT-SSX1 or SYT-SSX2 fusion protein (512) and Ewing sarcoma family of tumors with the classic t(11; 22) translocation forming EWS-FL1 fusion transcript, which acts as a c-myc pathway activator (1314). The other group includes sarcomas with complex karyotypes and multiple chromosomal abnormalities such as losses and gains, unbalanced translocations, and lack of fusion proteins (4101115). Examples of these include dedifferentiated liposarcoma, leiomyosarcoma, and pleomorphic sarcoma. Eventually, both these groups cause dysregulation of terminal signal pathways such as the vascular endothelial growth factor (VEGF), insulin-like growth factor 1, platelet-derived growth factor (PDGF), C-kit, phosphatidylinositol 3-kinase (PI3)/AKT/mechanistic target of rapamycin (mTor), and c-Met pathways (4915). All these factors are potential targets of MTTs (Fig. 1). Similar pathways are also responsible for carcinogenesis, and as such, many MTTs currently being used against carcinomas are also now increasingly used to treat sarcomas.
The revised classification and treatment options can have important implications for the radiologist especially from the aspect of treatment response. The increased use of MTTs has led to different manifestations of treatment response beyond the traditional size criteria, with tumor morphology and density becoming important aspects as well for the radiologist to factor in (16). Apart from the size-based Response Evaluation Criteria in Solid Tumors (RECIST), the Choi, mass, attenuation, size, and structure (MASS), and immune related response criteria have been introduced, amongst others, ushering in the era of personalized medicine and personalized radiology (16). Similarly, several new class-specific and drug-specific toxicities have been recognized (34).
Based on the tissue that they histologically resemble, STS are divided into 12 different categories (Table 2) (5). Most of the categories are relatively self-explanatory; for example, liposarcoma, leiomyosarcoma, and angiosarcoma show adipocytic, smooth muscle, and vascular differentiation, respectively. While discussing each category in detail is beyond the scope of this article, the salient changes compared to the 2002 classification are the inclusion of GISTs and peripheral nerve sheath tumors for the first time under STS, and the introduction of a new category of undifferentiated/unclassified tumors (167). The category ‘tumors of uncertain differentiation’ includes a heterogeneous subset of sarcomas whose tissue of origin is yet not clear; it includes tumors often seen in young adults such as synovial sarcoma, clear cell sarcoma, alveolar soft part sarcoma, extraskeletal Ewing sarcoma, and epithelioid sarcoma (57). ‘Undifferentiated/unclassified sarcomas’ is a new category that includes tumors in which no clear line of differentiation could be identified (167). Depending on the predominant cellular morphology, they could be classified as undifferentiated spindle cell, pleomorphic, round cell, or epithelioid sarcoma. Majority of the tumors previously classified as ‘malignant fibrous histiocytoma’ would now be included in this category (567).
The most common non-GIST STS include undifferentiated (pleomorphic) sarcoma, liposarcoma, and leiomyosarcoma (2417). Undifferentiated pleomorphic sarcoma presents as non-specific heterogeneous soft tissue masses with no reported characteristic features on imaging (1), and is not further discussed. We will review liposarcoma and leiomyosarcoma in detail and use them as prototypes to discuss the impact of the new classification and the role of the radiologist in management of sarcomas.
The major liposarcoma subtypes are well-differentiated, dedifferentiated, myxoid, and pleomorphic (5). Each of these subtypes is very different from each other on histopathology and has an associated specific radiologic correlate, making the role of the radiologist very useful in diagnosis and follow-up (181920).
Well-differentiated liposarcoma or atypical lipomatous tumor (ATL) is the most common liposarcoma, and is intermediate-locally aggressive, with a predilection for extremities, retroperitoneum, inguinal, and paratesticular regions (119). The term ATL is used in the extremities where they can be potentially completely resected and essentially cured. However, the term ‘well-differentiated liposarcoma’ is preferred in areas like the retroperitoneum where margin-negative resection is usually not possible (Fig. 2) (5719). It is often difficult to differentiate well-differentiated liposarcomas/ATL from lipomas on imaging, with imaging being sensitive but not specific for the same. Thick/nodular septations and nodular non-lipomatous component in an otherwise fatty lesion is suggestive of ATL (21222324). Large size (> 10 cm) has also been reported to be more common with an ATL (2224). On pathology, in addition to the histological findings, the presence of MDM2 and CDK4 positivity due to 12q13-15 amplification is a useful marker, a finding also seen in dedifferentiated liposarcoma (7).
Dedifferentiated liposarcomas are usually deep-seated, with the retroperitoneum being the most common site (likely as these present later) (18). The dedifferentiated component usually resembles pleomorphic sarcoma on pathology (in 90% of cases), and appears on imaging as a nodular non-lipomatous component adjacent to a predominantly fat attenuation tumor (Fig. 3) (181921). These tumors present as (usually) large predominantly fatty masses with a > 1 cm non-lipomatous component(s) that may be soft tissue, fluid or mixed density on CT, the former being most common. The pathological correlate of these different densities is uncertain, but may be due to inflammatory or myxoid component (21). Recurrence can be local in the form of a non-lipomatous lesion with or without fatty component, or as peritoneal sarcomatosis or distant metastases to the liver or lungs (19). A retrospective study of 60 patients observed fluid density lesions to grow faster and often convert to soft tissue density, although this was not statistically significant (21). It is important for the radiologist to pay attention to these non-lipomatous components rather than measuring the entire tumor when following up these patients for restaging or surveillance. Similarly, fluid density should not be ignored as post-surgical in etiology, and should be monitored closely on the follow-up studies as it may represent recurrent disease (19).
Myxoid liposarcoma is the second most common subtype, and unlike dedifferentiated liposarcoma, occurs more commonly in the extremities (181925). It is a cytogenetically simple tumor, demonstrating the t(12; 16) translocation to form the TLS-CHOP oncogene (167). Round cell liposarcoma, previously a separate category, is now understood to represent high grade myxoid liposarcoma and demonstrates the same translocation. The 2013 WHO classification accordingly discarded the term ‘round cell liposarcoma’ from the classification. These tumors are now considered to represent high-grade myxoid liposarcoma, with the prognosis worsening with increasing round cell component (> 5%) (192526).
The myxoid component of the tumor appears as fluid density on CT and extremely T2 hyperintense and T1 hypointense on MRI resembling fluid, but unlike cysts, demonstrates contrast enhancement (Fig. 4) (181925). Macroscopic fat is the clincher if observed on imaging, but may constitute < 10% of tumor volume and may be difficult to visualize on imaging (192527). Visualization of a non-fatty non-myxoid enhancing component may correspond to the round cell component, if present, and should be targeted for biopsy (2526). Given the presence of these various components, visualization of a true ‘cyst mimic’ (a tumor which could be misdiagnosed as a cyst without administering intravenous contrast) is uncommon (20). A retrospective study of the MRI features of extremity liposarcomas found lack of encapsulation and presence of solid nodular enhancement to be associated with higher tumor grade in myxoid liposarcomas (20). Presence of peritumoral edema and heterogeneous T2 appearance of the tumor was also found to indicate a more aggressive myxoid tumor and was predictive of the development of pulmonary metastases (20). The metastatic pattern is also unusual in these tumors, with retroperitoneal, opposite extremity, paraspinal, and bony involvement often preceding pulmonary involvement (1927). Bony metastases may be difficult to discern on CT and positron emission tomography (have variable fluorodeoxy glucose uptake), but are T2/short tau inversion recovery (STIR) bright and easily detected on MRI, and whole body MRI may be used for surveillance imaging (2528).
Pleomorphic liposarcoma is the least common and most aggressive subtype of liposarcoma, It most commonly occurs in the extremities, and has poor prognosis if metastatic (181927). It presents as an indeterminate heterogeneous soft tissue mass with absent or minimal fat within it (Fig. 5) (181927). The MRI features often overlap with high-grade myxoid liposarcoma although lack of septations and presence of T2 heterogeneity were found to differentiate pleomorphic liposarcoma from high-grade myxoid liposarcoma (20).
Surgical resection is the treatment of choice for these tumors. Additional adjuvant or neoadjuvant radiation may be given in dedifferentiated, myxoid and pleomorphic liposarcomas (18192129). Ifosfamide and anthracycline-based conventional chemotherapy is used in the neoadjuvant or adjuvant setting, as well as in patients with inoperable or metastatic disease. Trabectedin, a TLS-CHOP inhibitor, was Food and Drug Administration (FDA) approved as targeted therapy for liposarcoma and leiomyosarcoma in 2015, and is effective in myxoid and dedifferentiated liposarcoma (25313233). The role of the radiologist includes accurate tumor diagnosis and mapping for resection, tumor surveillance for recurrence, and response assessment. Apart from a decrease in size, chemotherapy or radiation may also induce the development of macroscopic fat in regions of previous tumor soft tissue (adipocytic maturation), which can be considered as a radiological marker of response (Fig. 6) (303435). The etiology of adipocytic maturation is uncertain, and may be due to treatment induced differentiation in tumor cells, or replacement of tumor cells with mature adipocytes. The clinical significance of this observation is however uncertain, with no definite change in patient prognosis (30343536). Unlike other liposarcomas, pleomorphic liposarcomas often respond to radiation therapy with an increase in size secondary to intratumoral hemorrhage and necrosis, and it is important for the radiologist to be aware of this fact (30). Given the MDM2 and CDK4 positivity of well-differentiated and dedifferentiated liposarcomas, early studies are currently underway to test MTTs against these receptors, with promising initial results (3738).
Leiomyosarcoma, the fourth most common sarcoma, can potentially arise from any structure containing smooth muscle, but most commonly arise in the uterus, retroperitoneum, extremities and large blood vessels (394041). Uterine leiomyosarcoma arise de novo from the uterus, with malignant transformation from a pre-existing fibroid being rare (424344). They affect middle to old-aged ladies (> 40 years) and present as large heterogeneous uterine masses on imaging with hemorrhage and necrosis (4245). Differentiating them from degenerating or cellular fibroids is particularly difficult in premenopausal women, as both can show a large size, rapid growth, and a heterogeneous appearance (although these features should be viewed with suspicion in postmenopausal women) (424344). Studies have suggested that MRI features such as ill-defined margins, greater than 50% T2 hyperintense signal, T1 hyperintense foci (due to hemorrhage or coagulative necrosis), low apparent diffusion coefficient values, and early enhancement on the dynamic contrast-enhanced study should be considered suspicious for leiomyosarcomas (45464748). Unlike fibroids, calcifications are consistently absent in leiomyosarcomas (49).
Smooth muscle tumor of uncertain malignant potential (STUMP) is a rare borderline entity that demonstrated non-benign features such as mild atypia or borderline mitotic activity, without any overt features to suggest leiomyosarcoma on pathology (4344). These most commonly arise from the uterus and cannot be definitively differentiated from fibroids or leiomyosarcoma on imaging, and may appear homogenous and T2 dark on MRI or may be heterogeneously enhancing (434445). Tumors may recur as STUMP or leiomyosarcoma (434450). This makes surveillance imaging extremely important.
Retroperitoneal and extremity leiomyosarcomas present as large heterogeneous masses, often with areas of necrosis, cystic change and hemorrhage, although subcutaneous/superficial tumors may appear smaller and homogeneous due to early detection (Figs. 7, 8) (394151). Large vessel leiomyosarcomas most commonly arise from the inferior vena cava (IVC), and may be intra or extraluminal (Fig. 9). The differential diagnosis of a soft tissue lesion arising from a large vessel would be an angiosarcoma or a tumor arising from adjacent structures and secondarily involving the vessel (3952). A recent study described an indiscernible IVC at the point of maximum contact with a retroperitoneal mass to be the most useful feature to predict an IVC leiomyosarcoma, with a negative embedded organ sign useful to exclude the same (53).
Leiomyosarcomas commonly metastasize to the lung, bones, and liver, with intra-abdominal tumors also often involving the peritoneum, and surveillance scans should cover the chest, abdomen and pelvis (394154). Treatment options include conventional chemotherapy, trabectedin and pazopanib (3233). The mechanism of action of trabectedin, which has been approved for use against STS in Europe, is not known but is believed to involve multiple DNA cellular pathways (including secondarily inhibiting the TLS-CHOP oncogene), causing changes in the tumor microenvironment (455). Toxicities include cytopenias, hepatitis, and fluid retention due to capillary leak syndrome leading to anasarca or pulmonary edema on imaging (455). Pazopanib is a VEGF inhibitor and secured FDA approval in 2012 for the treatment of advanced non-GIST non-adipocytic soft tissue sarcomas. Class-specific and drug-specific toxicities associated with it include diarrhea (secondary to colitis), nausea and vomiting, cardiovascular toxicities including hypertension, myocardial dysfunction, and thromboembolism, and hepatitis (5657).
Given the similar molecular pathways which lead to both carcinogenesis and sarcomagenesis, MTTs which were originally developed for treating carcinomas and common sarcomas like GIST are now being employed for treating rare sarcomas which were considered previously untreatable. In this section, we will discuss a few such sarcomas with specific molecular mechanisms and treatment options.
Imatinib, a small molecule tyrosine kinase inhibitor approved for management of GIST, can inhibit several receptor tyrosine kinases (C-kit, PDGF, colony stimulating factor receptor) and therefore can be effective in other sarcomas which have activating mutations of these kinases. Aggressive fibromatosis (desmoid tumor) is an intermediate-locally aggressive tumor that has a C-kit and platelet-derived growth factor receptor (PDGFR) driven mechanism of sarcomagenesis, and imatinib has been used in its treatment (5859). Imatinib has been found to be effective in dermatofibrosarcoma protuberans, a rare infiltrative cutaneous tumor (256061) which is driven by the t(17; 22) translocation and its resultant COL1A1-PDGFB fusion gene, which activates the PDGFR β receptor (6263). Few early studies have shown the efficacy of imatinib in pigmented villonodular synovitis (56465) which exhibits the translocation t(1; 2) and overstimulation of the macrophage colony stimulating factor gene (666768).
Inhibitors of mTOR, a protein kinase that is integral to the phosphatidylinositol 3-kinase pathway, are FDA approved for second line treatment of metastatic renal cell carcinoma (6970). mTOR also plays an important role in the pathogenesis of some sarcomas notably malignant perivascular epithelioid cell tumors which are uncommon tumors of the retroperitoneum and genitourinary tract (717273). For radiologists, knowledge of the toxicity profile of mTOR inhibitors especially pneumonitis is important as it may result in discontinuation of drug or dose reduction (Fig. 10).
Anaplastic lymphoma kinase (ALK) inhibitors like crizotinib which are FDA-approved for the treatment of ALK-driven non-small cell lung cancer (74) have been found to be effective in inflammatory myofibroblastic tumor (also known as inflammatory pseudotumor), an intermediate-rarely metastasizing tumor that affects children and young adults (575767778). Papillary renal cell cancer is an example of a c-Met driven carcinoma, and targeted inhibitors have shown promising results in early trials (7980). Alveolar soft part sarcoma, an uncommon tumor of the extremities in adolescent with a high incidence of pulmonary and brain metastases (81) has the t(X; 17) translocation activating the microphthalmia transcription factor (MIF) and the c-Met pathway. Clear cell sarcoma is another example of an MIF-driven tumor (82). Both of these sarcomas can be potentially treated with met inhibitors, with a recent phase II trial demonstrating safety of the drug with modest action (83).
Since the success of imatinib in treating GISTs, multiple other STS with known molecular mechanism of action are being treated with MTTs. In this era of precision medicine, in order to contribute meaningfully in their multidisciplinary management, it is important for the radiologist to be aware of the variable imaging features of STS, their management with conventional and targeted chemotherapy, and accurate assessment of tumor response and drug toxicities.
Figures and Tables
Fig. 1
Sarcomagenesis and potential target pathways for molecular targeted therapy (indicated by strikethrough).
PDGF = platelet-derived growth factor, VEGF = vascular endothelial growth factor
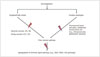
Fig. 2
61-year-old woman with well differentiated retroperitoneal liposarcoma.
A. Axial contrast-enhanced CT image at time of diagnosis demonstrates large predominantly fat containing mass in left retroperitoneum (arrows). Presence of heterogeneous non-lipomatous component differentiates it from simple lipoma. B, C. Axial T1-out-of-phase and post-gadolinium fat-suppressed T1-weighted images demonstrate mass to have areas of signal drop on out-of-phase imaging with no significant enhancement (arrows). Patient underwent surgery and histopathology showed well-differentiated liposarcoma.
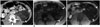
Fig. 3
50-year-old woman with dedifferentiated retroperitoneal liposarcoma.
Axial contrast-enhanced CT images demonstrate large heterogeneous predominantly soft tissue containing mass in right retroperitoneum (arrows). Superior component of mass has fatty attenuation area (arrowheads) raising possibility of dedifferentiated retroperitoneal liposarcoma which was confirmed at histopathology.
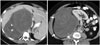
Fig. 4
41-year-old man with myxoid liposarcoma.
A. Axial T2-weighted image demonstrates homogeneously T2 hyperintense lesion mimicking cyst in anterior compartment of leg (arrow). B, C. Pre and post gadolinium fat-suppressed T1-weighted images demonstrate lesion to be T1 isointense with heterogeneous internal enhancement suggesting it to be solid mass (arrows). Patient underwent surgery and histopathology showed myxoid liposarcoma. T2 bright signal was due to myxoid component in tumor.
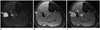
Fig. 5
61-year-old man with pleomorphic liposarcoma.
A. Axial STIR image demonstrates large heterogeneously T2 hyperintense mass in anterior compartment of left thigh associated with marked surrounding T2 hyperintensity (arrows). B. Axial T1-weighted non-fat-suppressed image shows mass to be T1 isointense with no hyperintense areas to suggest macroscopic fat in mass (arrows). C. Post-gadolinium fat-suppressed T1-weighted images demonstrate heterogeneous enhancement of mass (arrows). Histopathology showed pleomorphic liposarcoma. STIR = short tau inversion recovery
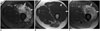
Fig. 6
64-year-old woman with dedifferentiated retroperitoneal liposarcoma treated with trabectedin.
A. Axial contrast-enhanced CT images before demonstrates large heterogeneous right perihepatic soft tissue mass (arrow). B. Follow-up CT 3 months after start of treatment shows decrease in density of mass with new fat attenuation component consistent with adipocytic maturation (arrow).
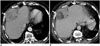
Fig. 7
60-year-old woman with leiomyosarcoma of deep soft tissues of extremity.
A. Axial T2 non-fat-suppressed images demonstrates large well circumscribed solid cystic/necrotic T2 hyperintense mass in anterior compartment of right thigh. Note that peripheral T2 hyperintense component has low signal compared to subcutaneous fat (arrows). B, C. Pre and post gadolinium fat-suppressed T1-weighted images demonstrate thick rim of peripheral enhancement in mass (arrows). Histopathology confirmed leiomyosarcoma.
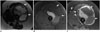
Fig. 8
81-year-old woman with retroperitoneal leiomyosarcoma.
Axial contrast-enhanced CT images of abdomen demonstrate large heterogeneous mass in left lower retroperitoneum (arrows), confirmed to be leiomyosarcoma on pathology.
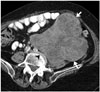
Fig. 9
58-year-old woman with inferior vena cava leiomyosarcoma.
Axial contrast-enhanced CT images demonstrate large heterogeneous mass in right retroperitoneum (arrows). IVC is not separately seen below level of renal veins and displaced anteriorly above level of renal veins (arrowhead). Right kidney is compressed and displaced laterally. IVC = inferior vena cava
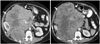
Fig. 10
72-year-old man with metastatic malignant PEComa treated with mTOR inhibitor.
A, B. Axial contrast-enhanced CT image of abdomen demonstrate heterogeneous mass in right lobe of liver (arrows) consistent with biopsy proven metastasis from malignant PEComa. CT image of chest at same time shows clear lung bases. C, D. Follow-up CT after 3 months of treatment shows decrease in density of liver metastasis (arrows). Second lesion which was previously occult also shows decrease in density (arrowhead). Concurrent chest CT shows new peripheral subpleural patchy ground glass opacities in lung bases consistent with drug associated pneumonitis. mTOR = mechanistic target of rapamycin, PEComa = perivascular epithelioid cell tumors
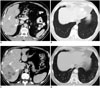
Table 1
Molecular Genetics Involved in Few Important Sarcomas
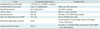
Table 2
Revised 2013 WHO Classification of Soft Tissue Sarcomas
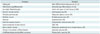
References
1. Baheti AD, O'Malley RB, Kim S, Keraliya AR, Tirumani SH, Ramaiya NH, et al. Soft-tissue sarcomas: an update for radiologists based on the revised 2013 World Health Organization classification. AJR Am J Roentgenol. 2016; 206:924–932.
2. Ducimetière F, Lurkin A, Ranchère-Vince D, Decouvelaere AV, Pèoc’h M, Istier L, et al. Incidence of sarcoma histotypes and molecular subtypes in a prospective epidemiological study with central pathology review and molecular testing. PLoS One. 2011; 6:e20294.
3. Tirumani SH, Jagannathan JP, Krajewski KM, Shinagare AB, Jacene H, Ramaiya NH. Imatinib and beyond in gastrointestinal stromal tumors: a radiologist's perspective. AJR Am J Roentgenol. 2013; 201:801–810.
4. Tirumani SH, Jagannathan JP, O'Regan K, Kim KW, Shinagare AB, Krajewski KM, et al. Molecular targeted therapies in non-GIST soft tissue sarcomas: what the radiologist needs to know. Cancer Imaging. 2013; 13:197–211.
5. Fletcher CDM, Bridge JA, Hogendoorn PCW, Mertens F. World Health Organization classification of tumours of soft tissue and bone: pathology and genetics of tumours of soft tissue and bone. 4th ed. Lyon: IARC Press;2013.
6. Fletcher CD. The evolving classification of soft tissue tumours -an update based on the new 2013 WHO classification. Histopathology. 2014; 64:2–11.
7. Jo VY, Fletcher CD. WHO classification of soft tissue tumours: an update based on the 2013 (4th) edition. Pathology. 2014; 46:95–104.
8. Helman LJ, Maki RG. Sarcomas of soft tissue. 5th ed. Philadelphia: Elsevier;2014.
9. Quesada J, Amato R. The molecular biology of soft-tissue sarcomas and current trends in therapy. Sarcoma. 2012; 2012:849456.
10. Matushansky I, Maki RG. Mechanisms of sarcomagenesis. Hematol Oncol Clin North Am. 2005; 19:427–449. v
11. Norberg SM, Movva S. Role of genetic and molecular profiling in sarcomas. Curr Treat Options Oncol. 2015; 16:24.
12. Baheti AD, Tirumani SH, Sewatkar R, Shinagare AB, Hornick JL, Ramaiya NH, et al. Imaging features of primary and metastatic extremity synovial sarcoma: a single institute experience of 78 patients. Br J Radiol. 2015; 88:20140608.
13. Murphey MD, Senchak LT, Mambalam PK, Logie CI, Klassen-Fischer MK, Kransdorf MJ. From the radiologic pathology archives: ewing sarcoma family of tumors: radiologic-pathologic correlation. Radiographics. 2013; 33:803–831.
14. Somarouthu BS, Shinagare AB, Rosenthal MH, Tirumani H, Hornick JL, Ramaiya NH, et al. Multimodality imaging features, metastatic pattern and clinical outcome in adult extraskeletal Ewing sarcoma: experience in 26 patients. Br J Radiol. 2014; 87:20140123.
15. Helman LJ, Maki RG. Sarcomas of soft tissue. In : Niederhuber JE, Armitage JO, Doroshow JH, Kastan MB, Tepper JE, editors. Abeloff's Clinical Oncology. 5th ed. Philadelphia: Elsevier;2014. p. 1753–1791.
16. Nishino M, Jagannathan JP, Krajewski KM, O'Regan K, Hatabu H, Shapiro G, et al. Personalized tumor response assessment in the era of molecular medicine: cancer-specific and therapy-specific response criteria to complement pitfalls of RECIST. AJR Am J Roentgenol. 2012; 198:737–745.
17. What are the key statistics about soft tissue sarcomas? Accessed Mar 29, 2016. Web site. http://www.cancer.org/cancer/sarcoma-adultsofttissuecancer/detailedguide/sarcoma-adult-soft-tissue-cancer-key-statistics.
18. Murphey MD, Arcara LK, Fanburg-Smith J. From the archives of the AFIP: imaging of musculoskeletal liposarcoma with radiologic-pathologic correlation. Radiographics. 2005; 25:1371–1395.
19. O'Regan KN, Jagannathan J, Krajewski K, Zukotynski K, Souza F, Wagner AJ, et al. Imaging of liposarcoma: classification, patterns of tumor recurrence, and response to treatment. AJR Am J Roentgenol. 2011; 197:W37–W43.
20. Wortman JR, Tirumani SH, Jagannathan JP, Tirumani H, Shinagare AB, Hornick JL, et al. Primary extremity liposarcoma: MRI features, histopathology, and clinical outcomes. J Comput Assist Tomogr. 2016; 40:791–798.
21. Tirumani SH, Wagner AJ, Tirumani H, Shinagare AB, Jagannathan JP, Hornick JL, et al. Is the nonlipomatous component of dedifferentiated liposarcoma always soft tissue on CT? Analysis of CT densities and correlation with rate of growth in 60 patients. Abdom Imaging. 2015; 40:1248–1254.
22. Brisson M, Kashima T, Delaney D, Tirabosco R, Clarke A, Cro S, et al. MRI characteristics of lipoma and atypical lipomatous tumor/well-differentiated liposarcoma: retrospective comparison with histology and MDM2 gene amplification. Skeletal Radiol. 2013; 42:635–647.
23. O'Donnell PW, Griffin AM, Eward WC, Sternheim A, White LM, Wunder JS, et al. Can experienced observers differentiate between lipoma and well-differentiated liposarcoma using only MRI? Sarcoma. 2013; 2013:982784.
24. Kransdorf MJ, Bancroft LW, Peterson JJ, Murphey MD, Foster WC, Temple HT. Imaging of fatty tumors: distinction of lipoma and well-differentiated liposarcoma. Radiology. 2002; 224:99–104.
25. Baheti AD, Tirumani SH, Rosenthal MH, Howard SA, Shinagare AB, Ramaiya NH, et al. Myxoid soft-tissue neoplasms: comprehensive update of the taxonomy and MRI features. AJR Am J Roentgenol. 2015; 204:374–385.
26. Löwenthal D, Zeile M, Niederhagen M, Fehlberg S, Schnapauff D, Pink D, et al. Differentiation of myxoid liposarcoma by magnetic resonance imaging: a histopathologic correlation. Acta Radiol. 2014; 55:952–960.
27. van Vliet M, Kliffen M, Krestin GP, van Dijke CF. Soft tissue sarcomas at a glance: clinical, histological, and MR imaging features of malignant extremity soft tissue tumors. Eur Radiol. 2009; 19:1499–1511.
28. Seo SW, Kwon JW, Jang SW, Jang SP, Park YS. Feasibility of whole-body MRI for detecting metastatic myxoid liposarcoma: a case series. Orthopedics. 2011; 34:e748–e754.
29. Keung EZ, Hornick JL, Bertagnolli MM, Baldini EH, Raut CP. Predictors of outcomes in patients with primary retroperitoneal dedifferentiated liposarcoma undergoing surgery. J Am Coll Surg. 2014; 218:206–217.
30. Wortman JR, Tirumani SH, Tirumani H, Shinagare AB, Jagannathan JP, Hornick JL, et al. Neoadjuvant radiation in primary extremity liposarcoma: correlation of MRI features with histopathology. Eur Radiol. 2016; 26:1226–1234.
31. Di Giandomenico S, Frapolli R, Bello E, Uboldi S, Licandro SA, Marchini S, et al. Mode of action of trabectedin in myxoid liposarcomas. Oncogene. 2014; 33:5201–5210.
32. Demetri GD, von Mehren M, Jones RL, Hensley ML, Schuetze SM, Staddon A, et al. Efficacy and safety of trabectedin or dacarbazine for metastatic liposarcoma or leiomyosarcoma after failure of conventional chemotherapy: results of a phase III randomized multicenter clinical trial. J Clin Oncol. 2016; 34:786–793.
33. Saponara M, Stacchiotti S, Gronchi A. The safety and efficacy of trabectedin for the treatment of liposarcoma or leiomyosarcoma. Expert Rev Anticancer Ther. 2016; 16:473–484.
34. Tirumani SH, Wagner AJ, Tirumani H, Shinagare AB, Jagannathan JP, Morgan JA, et al. Radiologic signs of adipocytic maturation (AM) in dedifferentiated liposarcoma (ddLPS) patients treated with trabectedin (T): correlation with disease control [abstract]. J Clin Oncol. 2014; 32:15 Suppl. Abstract no.10561.
35. Wang WL, Katz D, Araujo DM, Ravi V, Ludwig JA, Trent JC, et al. Extensive adipocytic maturation can be seen in myxoid liposarcomas treated with neoadjuvant doxorubicin and ifosfamide and pre-operative radiation therapy. Clin Sarcoma Res. 2012; 2:25.
36. Engström K, Bergh P, Cederlund CG, Hultborn R, Willen H, Aman P, et al. Irradiation of myxoid/round cell liposarcoma induces volume reduction and lipoma-like morphology. Acta Oncol. 2007; 46:838–845.
37. Dickson MA, Tap WD, Keohan ML, D'Angelo SP, Gounder MM, Antonescu CR, et al. Phase II trial of the CDK4 inhibitor PD0332991 in patients with advanced CDK4-amplified well-differentiated or dedifferentiated liposarcoma. J Clin Oncol. 2013; 31:2024–2028.
38. Ray-Coquard I, Blay JY, Italiano A, Le Cesne A, Penel N, Zhi J, et al. Effect of the MDM2 antagonist RG7112 on the P53 pathway in patients with MDM2-amplified, well-differentiated or dedifferentiated liposarcoma: an exploratory proof-of-mechanism study. Lancet Oncol. 2012; 13:1133–1140.
39. O'Sullivan PJ, Harris AC, Munk PL. Radiological imaging features of non-uterine leiomyosarcoma. Br J Radiol. 2008; 81:73–81.
40. Rosenberg AE. WHO classification of soft tissue and bone, fourth edition: summary and commentary. Curr Opin Oncol. 2013; 25:571–573.
41. Gordon RW, Tirumani SH, Kurra V, Shinagare AB, Jagannathan JP, Hornick JL, et al. MRI, MDCT features, and clinical outcome of extremity leiomyosarcomas: experience in 47 patients. Skeletal Radiol. 2014; 43:615–622.
42. Tirumani SH, Ojili V, Shanbhogue AK, Fasih N, Ryan JG, Reinhold C. Current concepts in the imaging of uterine sarcoma. Abdom Imaging. 2013; 38:397–411.
43. Shah SH, Jagannathan JP, Krajewski K, O'Regan KN, George S, Ramaiya NH. Uterine sarcomas: then and now. AJR Am J Roentgenol. 2012; 199:213–223.
44. D'Angelo E, Prat J. Uterine sarcomas: a review. Gynecol Oncol. 2010; 116:131–139.
45. Tanaka YO, Nishida M, Tsunoda H, Okamoto Y, Yoshikawa H. Smooth muscle tumors of uncertain malignant potential and leiomyosarcomas of the uterus: MR findings. J Magn Reson Imaging. 2004; 20:998–1007.
46. Schwartz LB, Zawin M, Carcangiu ML, Lange R, McCarthy S. Does pelvic magnetic resonance imaging differentiate among the histologic subtypes of uterine leiomyomata? Fertil Steril. 1998; 70:580–587.
47. Goto A, Takeuchi S, Sugimura K, Maruo T. Usefulness of Gd-DTPA contrast-enhanced dynamic MRI and serum determination of LDH and its isozymes in the differential diagnosis of leiomyosarcoma from degenerated leiomyoma of the uterus. Int J Gynecol Cancer. 2002; 12:354–361.
48. Sato K, Yuasa N, Fujita M, Fukushima Y. Clinical application of diffusion-weighted imaging for preoperative differentiation between uterine leiomyoma and leiomyosarcoma. Am J Obstet Gynecol. 2014; 210:368.e1–368.e8.
49. Amant F, Coosemans A, Debiec-Rychter M, Timmerman D, Vergote I. Clinical management of uterine sarcomas. Lancet Oncol. 2009; 10:1188–1198.
50. Guntupalli SR, Ramirez PT, Anderson ML, Milam MR, Bodurka DC, Malpica A. Uterine smooth muscle tumor of uncertain malignant potential: a retrospective analysis. Gynecol Oncol. 2009; 113:324–326.
51. Cooley CL, Jagannathan JP, Kurra V, Tirumani SH, Saboo SS, Ramaiya NH, et al. Imaging features and metastatic pattern of non-IVC retroperitoneal leiomyosarcomas: are they different from IVC leiomyosarcomas? J Comput Assist Tomogr. 2014; 38:687–692.
52. Rajiah P, Sinha R, Cuevas C, Dubinsky TJ, Bush WH Jr, Kolokythas O. Imaging of uncommon retroperitoneal masses. Radiographics. 2011; 31:949–976.
53. Webb EM, Wang ZJ, Westphalen AC, Nakakura EK, Coakley FV, Yeh BM. Can CT features differentiate between inferior vena cava leiomyosarcomas and primary retroperitoneal masses? AJR Am J Roentgenol. 2013; 200:205–209.
54. Tirumani SH, Deaver P, Shinagare AB, Tirumani H, Hornick JL, George S, et al. Metastatic pattern of uterine leiomyosarcoma: retrospective analysis of the predictors and outcome in 113 patients. J Gynecol Oncol. 2014; 25:306–312.
55. D'Incalci M, Galmarini CM. A review of trabectedin (ET-743): a unique mechanism of action. Mol Cancer Ther. 2010; 9:2157–2163.
56. van der Graaf WT, Blay JY, Chawla SP, Kim DW, Bui-Nguyen B, Casali PG, et al. Pazopanib for metastatic soft-tissue sarcoma (PALETTE): a randomised, double-blind, placebo-controlled phase 3 trial. Lancet. 2012; 379:1879–1886.
57. Rajendra R, Jones RL, Pollack SM. Targeted treatment for advanced soft tissue sarcoma: profile of pazopanib. Onco Targets Ther. 2013; 6:217–222.
58. Penel N, Le Cesne A, Bui BN, Perol D, Brain EG, Ray-Coquard I, et al. Imatinib for progressive and recurrent aggressive fibromatosis (desmoid tumors): an FNCLCC/French Sarcoma Group phase II trial with a long-term follow-up. Ann Oncol. 2011; 22:452–457.
59. Chugh R, Wathen JK, Patel SR, Maki RG, Meyers PA, Schuetze SM, et al. Efficacy of imatinib in aggressive fibromatosis: Results of a phase II multicenter Sarcoma Alliance for Research through Collaboration (SARC) trial. Clin Cancer Res. 2010; 16:4884–4891.
60. Torreggiani WC, Al-Ismail K, Munk PL, Nicolaou S, O'Connell JX, Knowling MA. Dermatofibrosarcoma protuberans: MR imaging features. AJR Am J Roentgenol. 2002; 178:989–993.
61. Kim GK. Status report on the management of dermatofibrosarcoma protuberans: is there a viable role for the use of imatinib mesylate? In which cases may it be therapeutically helpful and in which cases not? J Clin Aesthet Dermatol. 2011; 4:17–26.
62. Rutkowski P, Dębiec-Rychter M, Nowecki Z, Michej W, Symonides M, Ptaszynski K, et al. Treatment of advanced dermatofibrosarcoma protuberans with imatinib mesylate with or without surgical resection. J Eur Acad Dermatol Venereol. 2011; 25:264–270.
63. Labropoulos SV, Razis ED. Imatinib in the treatment of dermatofibrosarcoma protuberans. Biologics. 2007; 1:347–353.
64. Demetri GD, Antonia S, Benjamin RS, Bui MM, Casper ES, Conrad EU 3rd, et al. Soft tissue sarcoma. J Natl Compr Canc Netw. 2010; 8:630–674.
65. Imakiire N, Fujino T, Morii T, Honya K, Mochizuki K, Satomi K, et al. Malignant pigmented villonodular synovitis in the knee -report of a case with rapid clinical progression. Open Orthop J. 2011; 5:13–16.
66. Snoots WM, Watkins D, Dockery D, Mennel R, Cheek BS. Pigmented villonodular synovitis responsive to imatinib therapy. Proc (Bayl Univ Med Cent). 2011; 24:134–138.
67. Cassier PA, Gelderblom H, Stacchiotti S, Thomas D, Maki RG, Kroep JR, et al. Efficacy of imatinib mesylate for the treatment of locally advanced and/or metastatic tenosynovial giant cell tumor/pigmented villonodular synovitis. Cancer. 2012; 118:1649–1655.
68. Temple HT. Pigmented villonodular synovitis therapy with MSCF-1 inhibitors. Curr Opin Oncol. 2012; 24:404–408.
69. Mayer IA, Arteaga CL. The PI3K/AKT pathway as a target for cancer treatment. Annu Rev Med. 2016; 67:11–28.
70. Huang Z, Wu Y, Zhou X, Qian J, Zhu W, Shu Y, et al. Clinical efficacy of mTOR inhibitors in solid tumors: a systematic review. Future Oncol. 2015; 11:1687–1699.
71. Tirumani SH, Shinagare AB, Hargreaves J, Jagannathan JP, Hornick JL, Wagner AJ, et al. Imaging features of primary and metastatic malignant perivascular epithelioid cell tumors. AJR Am J Roentgenol. 2014; 202:252–258.
72. Dickson MA, Schwartz GK, Antonescu CR, Kwiatkowski DJ, Malinowska IA. Extrarenal perivascular epithelioid cell tumors (PEComas) respond to mTOR inhibition: clinical and molecular correlates. Int J Cancer. 2013; 132:1711–1717.
73. Wagner AJ, Malinowska-Kolodziej I, Morgan JA, Qin W, Fletcher CD, Vena N, et al. Clinical activity of mTOR inhibition with sirolimus in malignant perivascular epithelioid cell tumors: targeting the pathogenic activation of mTORC1 in tumors. J Clin Oncol. 2010; 28:835–840.
74. Lovly CM, Heuckmann JM, de Stanchina E, Chen H, Thomas RK, Liang C, et al. Insights into ALK-driven cancers revealed through development of novel ALK tyrosine kinase inhibitors. Cancer Res. 2011; 71:4920–4931.
75. Narla LD, Newman B, Spottswood SS, Narla S, Kolli R. Inflammatory pseudotumor. Radiographics. 2003; 23:719–729.
76. Patnana M, Sevrukov AB, Elsayes KM, Viswanathan C, Lubner M, Menias CO. Inflammatory pseudotumor: the great mimicker. AJR Am J Roentgenol. 2012; 198:W217–W227.
77. Butrynski JE, D'Adamo DR, Hornick JL, Dal Cin P, Antonescu CR, Jhanwar SC, et al. Crizotinib in ALK-rearranged inflammatory myofibroblastic tumor. N Engl J Med. 2010; 363:1727–1733.
78. Lovly CM, Gupta A, Lipson D, Otto G, Brennan T, Chung CT, et al. Inflammatory myofibroblastic tumors harbor multiple potentially actionable kinase fusions. Cancer Discov. 2014; 4:889–895.
79. de Bono JS, Yap TA. Future directions in the evaluation of c-MET-driven malignancies. Ther Adv Med Oncol. 2011; 3:1 Suppl. S51–S60.
80. Giubellino A, Linehan WM, Bottaro DP. Targeting the Met signaling pathway in renal cancer. Expert Rev Anticancer Ther. 2009; 9:785–793.
81. Sood S, Baheti AD, Shinagare AB, Jagannathan JP, Hornick JL, Ramaiya NH, et al. Imaging features of primary and metastatic alveolar soft part sarcoma: single institute experience in 25 patients. Br J Radiol. 2014; 87:20130719.
82. Davis IJ, McFadden AW, Zhang Y, Coxon A, Burgess TL, Wagner AJ, et al. Identification of the receptor tyrosine kinase c-Met and its ligand, hepatocyte growth factor, as therapeutic targets in clear cell sarcoma. Cancer Res. 2010; 70:639–645.
83. Wagner AJ, Goldberg JM, Dubois SG, Choy E, Rosen L, Pappo A, et al. Tivantinib (ARQ 197), a selective inhibitor of MET, in patients with microphthalmia transcription factor-associated tumors: results of a multicenter phase 2 trial. Cancer. 2012; 118:5894–5902.