Abstract
Obesity is a critical risk factor for the hypertension. Although angiotensin II (Ang II) in obese individuals is known to be upregulated in obesity-induced hypertension, direct evidence that explains the underlying mechanism for increased vascular tone and consequent increase in blood pressure (BP) is largely unknown. The purpose of this study is to investigate the novel mechanism underlying Ang II-induced hyper-contractility and hypertension in obese rats. Eight-week old male Sprague-Dawley rats were fed with 60% fat diet or normal diet for 4 months. Body weight, plasma lipid profile, plasma Ang II level, BP, Ang II-induced vascular contraction, and expression of regulatory proteins modulating vascular contraction with/without Ang II stimulation were measured. As a result, high fat diet (HFD) accelerated age-dependent body weight gaining along with increased plasma Ang II concentration. It also increased BP and Ang II-induced aortic contraction. Basal expression of p-CPI-17 and myosin light chain (MLC) kinase was increased by HFD along with increased phosphorylation of MLC. Ang II-induced phosphorylation of CPI-17 and MLC were also higher in HFD group than control group. In conclusion HFD-induced hypertension is through at least in part by increased vascular contractility via increased expression and activation of contractile proteins and subsequent MLC phosphorylation induced by increased Ang II.
World-wide population of obese people is growing fast [1]. Obesity is an important risk factor for hypertension [2]. Obese individuals have increased plasma level of renin-angiotensin-aldosterone system (RAAS) component including renin, angiotensinogen, angiotensin II (Ang II), and aldosterone [3]. RAAS plays a critical role in the elevation of blood pressure via regulating vascular contractility and blood volume [4]. High fat diet (HFD)-induced hypertension is established animal model to study renal and cardiovascular factors for the obesity-related hypertension [5678]. There are lines of evidence that inhibition of Ang II prevents HFD-induced hypertension [9].
Most forms of hypertension ultimately result from an increased vascular tone that leads to an elevated peripheral resistance [10]. Despite the clear role of Ang II in hypertension through increased vascular contraction [11] and increase in plasma level of Ang II by obesity [3], most studies on Ang II in obesity hypertension focused on water retention and blood volume expansion [12]. Thus far, a few studies on the mechanism of Ang II-induced vascular hyper-contractility in HFD-fed individuals have revealed that increased upstream mediators such as Ang II type 1 (AT1) receptor or angiotensin converting enzyme (ACE) are responsible for vascular smooth muscle (VSM) hypercontractility. Experiment using VSM cell model of metabolic syndrome demonstrated that increased release of reactive oxygen species (ROS) elevated Ang II-induced Ca2+ release and AT1 expression supporting augmented aortic contraction in response to Ang II [1314]. However, the clear role of increased basal level of Ang II in maintained resting VSM hyper-contractility in obese hypertensive individuals is not elucidated yet. Here, we studied the resting status of VSM contractile proteins affected by HFD and the mechanism underlying Ang II-induced hyper-contractility focusing on the alterations of VSM contractile proteins to elucidate the mechanism of HFD-induced hypertension.
VSM contraction absolutely depends on 20 KDa MLC phosphorylation that is determined by balance of MLC kinase (MLCK) and MLC phosphatase (MLCP) [15]. MLCK is activated by intracellular calcium and then phosphorylates MLC resulting in VSMC contraction. On the other hand MLCP is inhibited in calcium-independent manner that is occurred by phosphorylation of myosin phosphatase target subunit1 (MYPT1) at Thr853 residue or inhibition of catalytic subunit pp1c by C-kinase potentiated Protein phosphatase-1 Inhibitor (CPI17) resulting in increased MLC phosphorylation and VSM contraction [16]. Therefore altered amount and activation status of these contractile mediators by HFD should be critical for the obesity-induced hypertension and hyper-reactivity to Ang II. In this study, we tested the hypothesis that altered regulatory proteins for the vascular contraction such as MLCK and MLCP inhibitor CPI-17 are responsible for HFD-induced hyper-contractility and hypertension.
All animal experiments were conducted in accordance with guidelines of the National Institutes of Health for the care and use of laboratory animals. Experimental protocol (KM-2014-43) was approved by institutional Animal Care and Use Committee at Keimyung University. Eight week-old male Sprague-Dawley rats were purchased from Koatec (Daegu, Korea) and used. They were fed with 60% Kcal fat diet (TD.06414, Harlan Teklad, Madison, WI, USA) or its control diet containing 10% Kcal fat diet (TD.94048, Harlan Teklad, Madison, WI, USA) and had free access to water for 16 weeks as others induced diet-induced obesity previously [17].
The plasma levels of total cholesterol, HDL-cholesterol, LDL cholesterol, triglycerides, and free fatty acids were measured by an automatic biochemical Analyzer (Roche Diagnostics, Basel, Switzerland).
Angiotensin II enzyme immunoassay kit (Cat. EK-002-12; Phoenix Pharmaceutical Inc., Burlingame, CA) was used for measuring the concentration of angiotensin II in rat plasma. Fifty microliter of rat plasma in duplicate was used for this assay. All the procedures were conducted according to the instruction of the assay kit.
Blood pressure (BP) of rats was measured by tail cuff method. Rats were preheated on a hot plate at 35℃ for 10 min and then placed in restrainer. A cuff with a pneumatic pulse sensor was attached to the tail. BP values were recorded on a CODA High Throughput Noninvasive Blood Pressure system (Kent Scientific, Torrington, CT, USA) with heating and were averaged from ten consecutive readings obtained from each rat.
To minimize the pain and stress, rats were anesthetized with pentobarbital sodium (50 mg/kg ip) before sacrifice. The thoracic aorta was excised from the rats under anesthesia. By excising aorta, rats were sacrificed. The excised aorta was placed into ice-cold modified Krebs-Henseleit buffer, pH 7.4. The aortae were cleaned of all adherent connective tissue and cut into rings (4 mm long). The endothelium was removed by gentle rubbing of the intimal surface using tip of small forceps.
The aortic rings were horizontally mounted in 3 ml organ baths filled with Krebs-Henseleit buffer (pH 7.4), continuously bubbling with gas containing 95% O2 and 5% CO2. The changes in isometric tension of the rings were measured with a digital force isometric transducer (Grass, West Warwick, RI, USA) connected to data acquisition system (AD Instruments, New South Wales, Australia). The rings were equilibrated for 1 h under an initial tension of 2 g, with the buffer being changed every 10 min. After equilibration, the rings were challenged with 50 mM KCl and force was measured. After wash out and 1 h of equilibrium final concentration of 10 nM Ang II were added to the baths to obtain vascular contractile response. The contractions were induced at a basal tension of 2 g and calculated as grams tension per aortic ring.
Protein samples were prepared and western blot analysis were performed as previously described [18]. Protein samples were electrophoresed on 10~12% polyacrylamide gel with 0.1% SDS and transferred to PVDF membranes, and then subjected to an immunoblotting with antibodies against pMLC at Thr18 and Ser19 (Santa Cruz, CA, USA ), p-MYPT1 at Thr853 (Santa Cruz, CA, USA), p-CPI-17 at Thr38 (Millipore, Darmstadt, Germany), and MLCK (Santa Cruz, CA, USA). For the loading control anti-GAPDH antibody (Novus Biologicals, CO, USA) was used. Horseradish peroxidase-conjugated secondary antibodies (anti-rabbit: Santa Cruz, CA, USA; anti-goat: Bethyl, Montgomery, TX, USA; anti-mouse: Bethyl, Montgomery, TX, USA) were applied, and immunoblots were visualized using chemiluminescence reagent (PerkinElmer Life Sciences, MA, USA). Densities of immunoblots were quantified using image analysis software ImageJ (NIH, Bethesda, ML, USA).
From 8 week of age, rats were randomly divided into 2 groups for normal diet (ND) or HFD. At 8 week old, there was no difference in the body weight between groups (258.2±4.4 g, 257.6±3.8 g for ND and HFD group respectively). With time, both ND group and HFD group gained weight. At 24 weeks, body weights were 498.2±6.5 g and 546.6±12.8 g for ND and HFD group respectively. ND group gained 240.0 g while HFD group gained 289.0 g showing greater weight gaining by 20% in HFD group compared to ND group (p=0.003) (Fig. 1).
At starting point systolic BP was 123.2±2.9 mmHg and 120.2±5.8 mmHg, and diastolic BP was 94.2±2.8 mmHg and 93.1±6.8 mmHg for ND and HFD group respectively showing no difference between groups (Fig. 2a). With differential diet for 16 weeks, systolic BP and diastolic BP of both groups increased. Systolic BP was 155.1±6.9 mmHg and 176.0±7.0 mmHg, and diastolic BP was 112.9±6.6 mmHg and 128.7±6.3 mmHg for ND and HFD group respectively showing accelerated increase in HFD group compared to ND group (p=0.023, p=0.049 for systolic BP and diastolic BP respectively) (Fig. 2b).
For the plasma lipid profiling, LDL cholesterol was higher in HFD group than ND group (p=0.032) but other lipids such as HDL cholesterol, triglycerides, free fatty acids did not show any difference between groups (Fig. 3a to e). Plasma Ang II was higher in HFD group (0.432±0.082 ng/µl) than in ND group (0.275±0.016 ng/µl) (p=0.034) (Fig. 3f).
In order to test whether HFD-induced hypertension is caused by increased Ang II-induced vascular contraction, I determined vascular contraction against Ang II normalized by KCl-induced contraction to minimize the difference in the initial viability between aorta rings. KCl-induced contraction was 2.6±0.1 g and 3.1±0.4 g (Fig. 4a). Ang II-induced contraction was 0.6±0.1 g in ND and 1.0±0.1 g in HFD group (Fig. 4b). Normalized Ang II-induced contraction was 22.7±2.5% in ND group and 32.1±4.1% in HFD group showing greater vascular contractility in HFD group (p=0.035) by 41.4% (Fig. 4c).
In order to investigate mechanism underlying increased vascular tone we examined expression and phosphorylation status of regulator proteins for the vascular contraction with/without HFD and Ang II stimulation. As a result, basal MLCK expression and p-CPI-17 were greatly higher in HFD group than ND group (p=0.003, 0.011 respectively) (Fig. 5b). Ang II-induced phosphorylation of CPI-17 were greatly higher in HFD group than ND group (p=0.025) (Fig. 5c). MYPT1 had a tendency of higher phosphorylation in HFD group than ND group (Fig. 5d). Consequently, MLC phosphorylation induced by Ang II was much greater in HFD group than ND group (p=0.020) (Fig. 5e) supporting enhanced contraction in HFD group.
Because basal MLCK and CPI-17 were upregulated, I checked that basal MLC phosphorylation was also increased in HFD group. I used 3 times more protein sample because the basal phosphorylation level of MLC was very low. Because of the difference in magnitude between basal and Ang II-stimulated phosphorylation, I retested only basal level of phosphorylation in ND and HFD group. As a result, basal MLC phosphorylation was 35 times higher in HFD group than ND group (p=0.020) (Fig. 5g, h).
Herein, I investigated the mechanism underlying HFD-induced hypertension and hyper-reactivity in response to Ang II. The most important new finding of this study is that HFD upregulated VSM contraction regulatory proteins such as MLCK and MLCP inhibitor protein CPI-17 in both resting and Ang II-stimulated condition resulting in increased Ang II-induced VSM contraction and BP. Our data suggest that MLCK and CPI-17 could be a novel target for the treatment of hypertension in obese individuals particularly induced by HFD, which is one of the most common causes of obesity in modern society.
Because rapidly growing obese population is highly associated with increase in serious cardiovascular disease and hypertension with consequent disability and early death [119], treating obesity-induced hypertension is very important. Based on small controlled trials and clinical experiences angiotensin converting enzyme inhibitor, thiazides, calcium channel blockers, and statins turned to be useful in treating obesity-related hypertension [20]. However, these drugs draw adverse effects including tolerability [21], renal dysfunction [22], angina, arrhythmias, hypotension, arthritis, asthma etc. [23]. Therefore, more specific targets are needed to treat obesity-induced hypertension safely and effectively.
It is well known that circulating RAAS components are increased and positively correlated with BP in the obese hypertensives [2425]. Our result also demonstrated increased plasma Ang II level in HFD-fed mice. It has been reported that Ang II involves in HFD-induced hypertension through various pathways including leptin-related nephropathy [26], restoration of sympathetic over-reactivity [27], megalin-dependent uptake of angiotensinogen into adipocytes [28], vascular oxidative stress and endothelial dysfunction [29], and increased VSM reactivity [131430]. Increased VSM reactivity to Ang II in HFD-fed individuals is reported to be attributed to increased expression of AT1R and ACE 2 [13], and reactive oxygen species (ROS) overproduction and enhanced release of Ca2+ induced by Ang II [14]. However drugs inhibiting most of these upstream mediators of Ang II have undesirable effects as mentioned here earlier. Thus in this study, VSM contractile proteins as downstream effectors of Ang II activation in HFD-induced hypertension was studied.
Activation of AT1 receptor by Ang II in VSM causes phospholipase C activation leading to generation of inositol triphosphate (IP3) and diacylglycerol (DAG), that activates intracellular Ca2+ release and protein kinase C respectively [31]. Increased Ca2+ activates MLCK resulting in MLC phosphorylation and VSMC contraction [1631]. In the present study, MLCK expression was increased by HFD, likely enhancing this event. I presume that it maintains vasoconstriction and blood pressure elevation. On the other hand PKC enhances potency of CPI-17 to inhibit MLCP by approximately 1000 folds through phosphorylating threonine 38 residue of CPI-17 [32]. The results also revealed that both basal and Ang II-induced phosphorylation of CPI-17 in HFD group was greatly higher than ND group explaining increased phosphorylated MLC and blood pressure.
The data showed that 16 weeks of HFD significantly increased plasma LDL cholesterol concentration while other lipids did not change suggesting moderate condition of metabolic syndrome. LDL cholesterol is known to cause impairment of vascular relaxation via superoxide-mediated endothelial dysfunction in metabolic disease such as diabetes [33] suggesting that LDL cholesterol also likely affected abnormal vascular tension in HFD-fed rats in this study.
In conclusion HFD increased plasma Ang II level, MLCK expression and inhibited MLCP by activation of CPI-17 resulting in enhanced MLC phosphorylation. I suggest that enhanced basal MLC phosphorylation and consequent VSM hyper-contractility by increased level of Ang II is responsible for HFD-induced hypertension.
ACKNOWLEDGEMENTS
This research was supported by the Bisa Research Grant of Keimyung University in 2014.
I thank Wyju Jin for the technical support in the experiments.
Notes
References
2. Mark AL, Correia M, Morgan DA, Shaffer RA, Haynes WG. State-of-the-art-lecture: Obesity-induced hypertension: new concepts from the emerging biology of obesity. Hypertension. 1999; 33:537–541. PMID: 9931161.
3. Goodfriend TL, Calhoun DA. Resistant hypertension, obesity, sleep apnea, and aldosterone: theory and therapy. Hypertension. 2004; 43:518–524. PMID: 14732721.
4. Gu P, Xu A. Interplay between adipose tissue and blood vessels in obesity and vascular dysfunction. Rev Endocr Metab Disord. 2013; 14:49–58. PMID: 23283583.


5. Uemura K, Mori N. Influence of age and sex on high-fat diet-induced increase in blood pressure. Nagoya J Med Sci. 2006; 68:109–114. PMID: 16967776.
6. Davies MR, Gleich K, Katerelos M, Lee M, Mount PF, Power DA. The Thiazide-Sensitive Co-Transporter Promotes the Development of Sodium Retention in Mice with Diet-Induced Obesity. Kidney Blood Press Res. 2015; 40:509–519. PMID: 26418861.


7. Vargas-Robles H, Rios A, Arellano-Mendoza M, Escalante BA, Schnoor M. Antioxidative diet supplementation reverses high-fat diet-induced increases of cardiovascular risk factors in mice. Oxid Med Cell Longev. 2015; 2015:467471. PMID: 25922641.


8. Boustany-Kari CM, Gong M, Akers WS, Guo Z, Cassis LA. Enhanced vascular contractility and diminished coronary artery flow in rats made hypertensive from diet-induced obesity. Int J Obes (Lond). 2007; 31:1652–1659. PMID: 16819529.


9. Wang Y, Song Y, Suo M, Jin X, Tian G. Telmisartan prevents high-fat diet-induced hypertension and decreases perirenal fat in rats. J Biomed Res. 2012; 26:219–225. PMID: 23554752.


10. Wirth A, Benyó Z, Lukasova M, Leutgeb B, Wettschureck N, Gorbey S, Orsy P, Horváth B, Maser-Gluth C, Greiner E, Lemmer B, Schütz G, Gutkind JS, Offermanns S. G12-G13-LARG-mediated signaling in vascular smooth muscle is required for salt-induced hypertension. Nat Med. 2008; 14:64–68. PMID: 18084302.


11. Brooks DP, Ruffolo RR Jr. Pharmacological mechanism of angiotensin II receptor antagonists: implications for the treatment of elevated systolic blood pressure. J Hypertens Suppl. 1999; 17:S27–S32. PMID: 10465064.
12. Hall JE, do Carmo JM, da Silva AA, Wang Z, Hall ME. Obesity-induced hypertension: interaction of neurohumoral and renal mechanisms. Circ Res. 2015; 116:991–1006. PMID: 25767285.
13. Kadakol A, Malek V, Goru SK, Pandey A, Bagal S, Gaikwad AB. Esculetin attenuates alterations in Ang II and acetylcholine mediated vascular reactivity associated with hyperinsulinemia and hyperglycemia. Biochem Biophys Res Commun. 2015; 461:342–347. PMID: 25887801.


14. Zhang X, Yan SM, Zheng HL, Hu DH, Zhang YT, Guan QH, Ding QL. A mechanism underlying hypertensive occurrence in the metabolic syndrome: cooperative effect of oxidative stress and calcium accumulation in vascular smooth muscle cells. Horm Metab Res. 2014; 46:126–132. PMID: 24108391.


15. Savineau JP, Marthan R. Modulation of the calcium sensitivity of the smooth muscle contractile apparatus: molecular mechanisms, pharmacological and pathophysiological implications. Fundam Clin Pharmacol. 1997; 11:289–299. PMID: 9263758.


16. Somlyo AP, Somlyo AV. Ca2+ sensitivity of smooth muscle and nonmuscle myosin II: modulated by G proteins, kinases, and myosin phosphatase. Physiol Rev. 2003; 83:1325–1358. PMID: 14506307.
17. Margolis M, Perez O Jr, Martinez M, Santander AM, Mendez AJ, Nadji M, Nayer A, Bhattacharya S, Torroella-Kouri M. Phospholipid makeup of the breast adipose tissue is impacted by obesity and mammary cancer in the mouse: Results of a pilot study. Biochimie. 2015; 108:133–139. PMID: 25450252.


18. Kim JI, Jung SW, Yang E, Park KM, Eto M, Kim IK. Heat shock augments angiotensin II-induced vascular contraction through increased production of reactive oxygen species. Biochem Biophys Res Commun. 2010; 399:452–457. PMID: 20688045.


20. Soltani Z, Washco V, Morse S, Reisin E. The impacts of obesity on the cardiovascular and renal systems: cascade of events and therapeutic approaches. Curr Hypertens Rep. 2015; 17:7. PMID: 25620635.


21. Dharmarajan TS, Dharmarajan L. Tolerability of Antihypertensive Medications in Older Adults. Drugs Aging. 2015; 32:773–796. PMID: 26442857.


22. Abraham HM, White CM, White WB. The comparative efficacy and safety of the angiotensin receptor blockers in the management of hypertension and other cardiovascular diseases. Drug Saf. 2015; 38:33–54. PMID: 25416320.


23. Curb JD, Schneider K, Taylor JO, Maxwell M, Shulman N. Antihypertensive drug side effects in the Hypertension Detection and Follow-up Program. Hypertension. 1988; 11:II51–II55. PMID: 3350594.


24. Massiéra F, Bloch-Faure M, Ceiler D, Murakami K, Fukamizu A, Gasc JM, Quignard-Boulange A, Negrel R, Ailhaud G, Seydoux J, Meneton P, Teboul M. Adipose angiotensinogen is involved in adipose tissue growth and blood pressure regulation. FASEB J. 2001; 15:2727–2729. PMID: 11606482.


25. Cooper R, McFarlane-Anderson N, Bennett FI, Wilks R, Puras A, Tewksbury D, Ward R, Forrester T. ACE, angiotensinogen and obesity: a potential pathway leading to hypertension. J Hum Hypertens. 1997; 11:107–111. PMID: 9140797.


26. Li H, Li M, Liu P, Wang Y, Zhang H, Li H, Yang S, Song Y, Yin Y, Gao L, Cheng S, Cai J, Tian G. Telmisartan ameliorates nephropathy in metabolic syndrome by reducing leptin release from perirenal adipose tissue. Hypertension. 2016; 68:478–490. PMID: 27296996.


27. Maneesai P, Bunbupha S, Kukongviriyapan U, Prachaney P, Tangsucharit P, Kukongviriyapan V, Pakdeechote P. Asiatic acid attenuates renin-angiotensin system activation and improves vascular function in high-carbohydrate, high-fat diet fed rats. BMC Complement Altern Med. 2016; 16:123. PMID: 27121076.


28. Yiannikouris F, Wang Y, Shoemaker R, Larian N, Thompson J, English VL, Charnigo R, Su W, Gong M, Cassis LA. Deficiency of angiotensinogen in hepatocytes markedly decreases blood pressure in lean and obese male mice. Hypertension. 2015; 66:836–842. PMID: 26303292.


29. Kosaka S, Pelisch N, Rahman M, Nakano D, Hitomi H, Kobori H, Fukuoka N, Kobara H, Mori H, Masaki T, Cervenka L, Matsumura Y, Houchi H, Nishiyama A. Effects of angiotensin II AT1-receptor blockade on high fat diet-induced vascular oxidative stress and endothelial dysfunction in Dahl salt-sensitive rats. J Pharmacol Sci. 2013; 121:95–102. PMID: 23337436.
30. Viswanad B, Srinivasan K, Kaul CL, Ramarao P. Effect of tempol on altered angiotensin II and acetylcholine-mediated vascular responses in thoracic aorta isolated from rats with insulin resistance. Pharmacol Res. 2006; 53:209–215. PMID: 16412660.


31. Wynne BM, Chiao CW, Webb RC. Vascular smooth muscle cell signaling mechanisms for contraction to angiotensin II and endothelin-1. J Am Soc Hypertens. 2009; 3:84–95. PMID: 20161229.


32. Walsh MP, Susnjar M, Deng J, Sutherland C, Kiss E, Wilson DP. Phosphorylation of the protein phosphatase type 1 inhibitor protein CPI-17 by protein kinase C. Methods Mol Biol. 2007; 365:209–223. PMID: 17200564.


33. Kobayashi T, Matsumoto T, Kamata K. The PI3-K/Akt pathway: roles related to alterations in vasomotor responses in diabetic models. J Smooth Muscle Res. 2005; 41:283–302. PMID: 16557003.


Fig. 1
High fat diet (HFD) accelerated weight gaining.
The graph summarized body weights for normal or HFD-fed group at 8 week (before differential diet) and 24 week (16 weeks of differential diet) of age. Body weight increase for 16 weeks of diet was accelerated in HFD group. Data are presented as means±SE (n=4). **p<0.01 vs. normal diet group of same age.
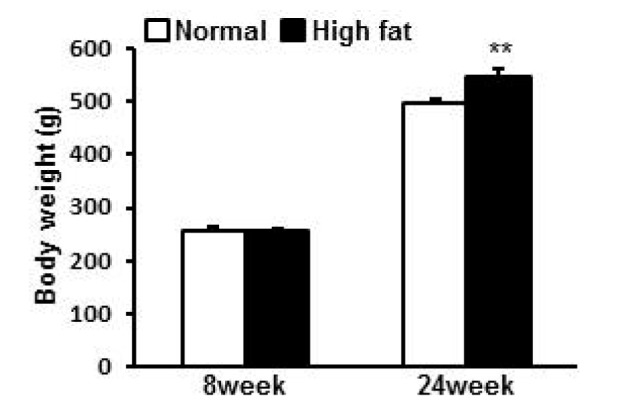
Fig. 2
HFD elevated blood pressure (BP).
The graph summarized blood pressure before HFD at 8 week (a) and after HFD for 16 week at 24 week of age (b). Both systolic and diastolic BP was higher in HFD group than ND group. Data are presented as means±SE (n=4). *p<0.05 vs. ND group.
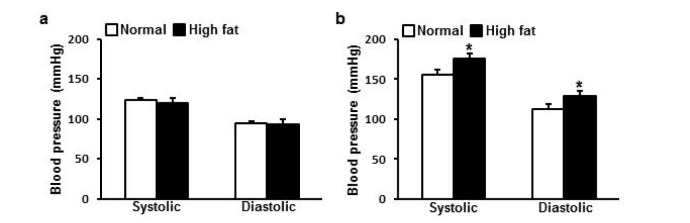
Fig. 3
Lipids and Angiotensin II (Ang II) in the plasma of normal or HFD-fed rats.
The graph summarized total cholesterol (a), high density lipoprotein (HDL) (b), low density lipoprotein (LDL) (c), triglyceride (d), free fatty acid (e), and Ang II (f ) in the plasma of normal diet (ND) or HFD-fed rats. Plasma LDL and Ang II were higher in HFD group than ND group. Data are presented as means±SE (n=4). *p<0.05 vs. ND group. N, normal; HF, high fat.
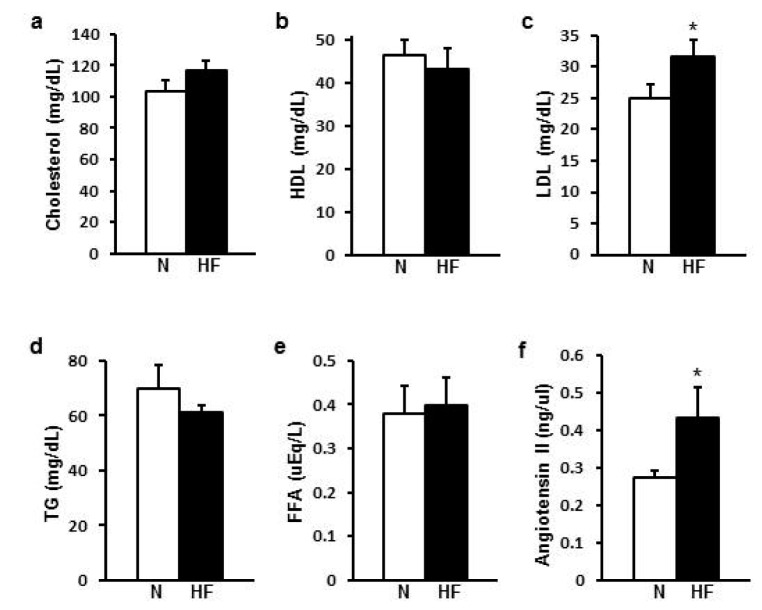
Fig. 4
HFD increased Ang II-induced vascular smooth muscle contraction.
The graph summarized KCl-induced
contraction (a), Ang II-induced contraction (b), and Ang II-induced contraction normalized by KCl-induced contraction (c) of ND or HFD-fed rat aorta. Endothelium denuded aortic ring was treated with 50 mM KCl or 10 nM of Ang II. Ang II-induced aortic contraction was higher in HFD group than ND group. Data are presented as means±SE (n=4). *p<0.05 vs. Ang II-induced contraction of ND-fed rat aorta. N, normal; HF, high fat. (d, e) Representative tracings of contractile responses in normal diet group (d) or high fat diet group (e). Traces show KCl-induced contraction followed by Ang II-induced contraction.
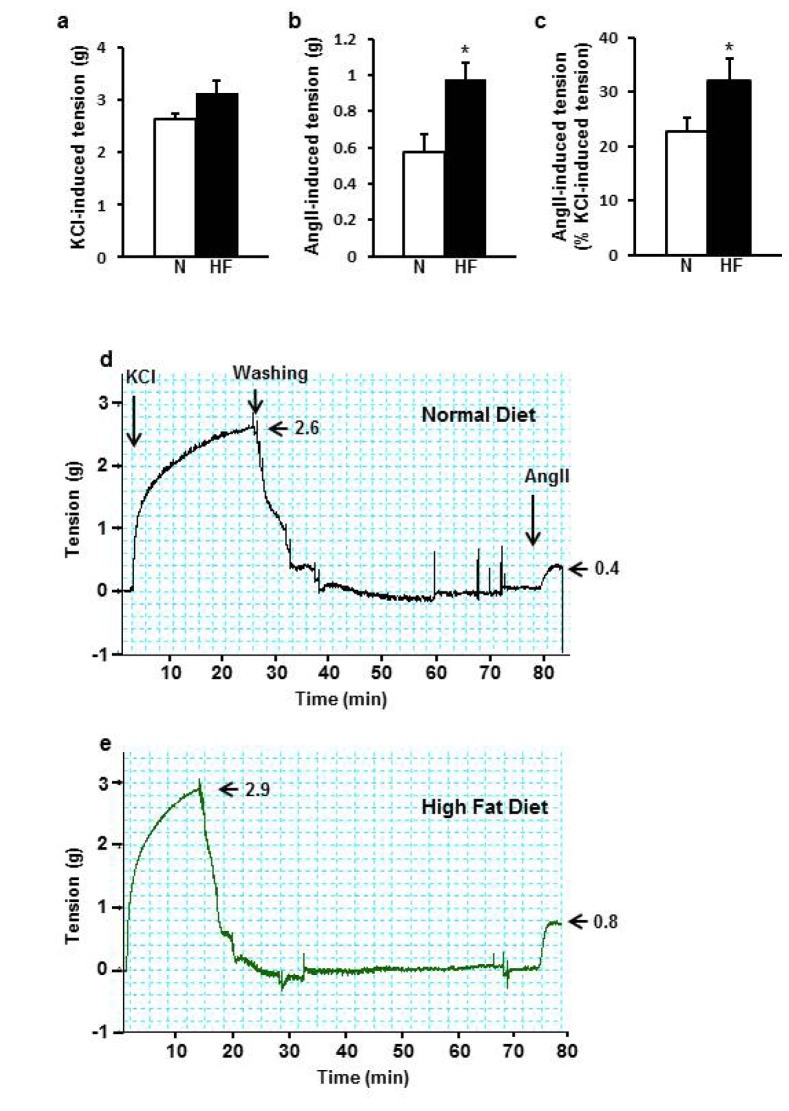
Fig. 5
HFD increased basal expression of MLCK and phosphorylation of CPI-17.
(a) Representative picture of western blot for phosphorylated MLC at Thr18 and Ser19, phosphorylated MYPT1 at Thr853, phosphorylated CPI-17 at Thr38, MLCK, and internal control GAPDH in ND or HFD-fed rat aorta with/without stimulation. (b~e) Summary of protein expression of MLCK (b), p-CPI-17 (c), p-MYPT1 (d), and p-MLC (e). (f) Representative picture of western blot for phodphorylated MLC at resting state in ND and HFD-fed rat aorta (g). Summary of phosphorylation of MLC. Basal expression of MLCK, p-CPI-17, p-MLC was increased by HFD. Ang II-induced phosphorylation of CPI-17 and MLC was also enhanced by HFD. Data are presented as means±SE (n=4). *p<0.05, **p<0.01.
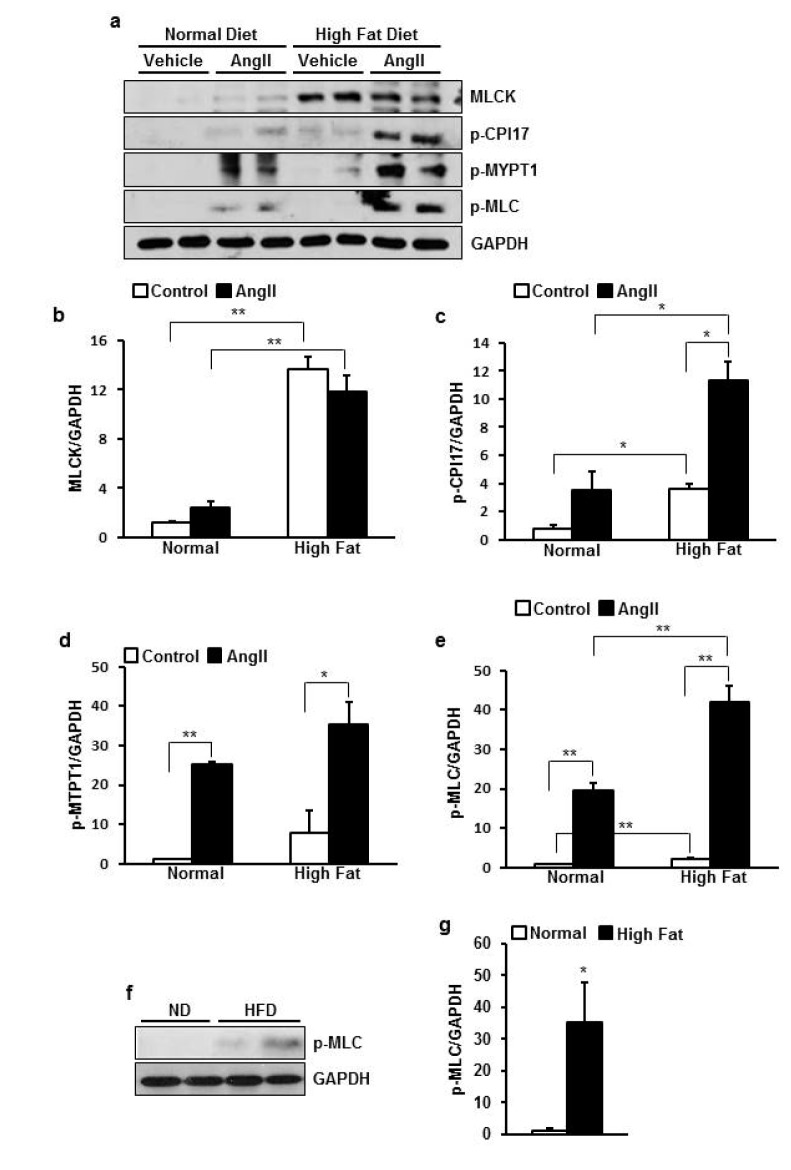