Abstract
The present study was designed to investigate the characteristics of gintonin, one of components isolated from Korean Ginseng on secretion of catecholamines (CA) from the isolated perfused model of rat adrenal gland and to clarify its mechanism of action. Gintonin (1 to 30 µg/ml), perfused into an adrenal vein, markedly increased the CA secretion from the perfused rat adrenal medulla in a dose-dependent fashion. The gintonin-evoked CA secretion was greatly inhibited in the presence of chlorisondamine (1 µM, an autonomic ganglionic bloker), pirenzepine (2 µM, a muscarinic M1 receptor antagonist), Ki14625 (10 µM, an LPA1/3 receptor antagonist), amiloride (1 mM, an inhibitor of Na+/Ca2+ exchanger), a nicardipine (1 µM, a voltage-dependent Ca2+ channel blocker), TMB-8 (1 µM, an intracellular Ca2+ antagonist), and perfusion of Ca2+-free Krebs solution with 5mM EGTA (a Ca2+chelater), while was not affected by sodium nitroprusside (100 µM, a nitrosovasodialtor). Interestingly, LPA (0.3~3 µM, an LPA receptor agonist) also dose-dependently enhanced the CA secretion from the adrenal medulla, but this facilitatory effect of LPA was greatly inhibited in the presence of Ki 14625 (10 µM). Moreover, acetylcholine (AC)-evoked CA secretion was greatly potentiated during the perfusion of gintonin (3 µg/ml). Taken together, these results demonstrate the first evidence that gintonin increases the CA secretion from the perfused rat adrenal medulla in a dose-dependent fashion. This facilitatory effect of gintonin seems to be associated with activation of LPA- and cholinergic-receptors, which are relevant to the cytoplasmic Ca2+ increase by stimulation of the Ca2+ influx as well as by the inhibition of Ca2+ uptake into the cytoplasmic Ca2+ stores, without the increased nitric oxide (NO). Based on these results, it is thought that gintonin, one of ginseng components, can elevate the CA secretion from adrenal medulla by regulating the Ca2+ mobilization for exocytosis, suggesting facilitation of cardiovascular system. Also, these findings show that gintonin might be at least one of ginseng-induced hypertensive components.
Lim and his coworkers [123] have found that all of TGS, panaxadiol and panaxatriol cause the increased secretion of catecholamines (CA) from the isolated perfused rabbit adrenal glands in a Ca2+-dependent fashion, which are mediated by the activation of cholinergic (both nicotinic and muscarinic) receptors and partly the direct action on the rabbit adrenomedullary chromaffin cells. However, it has been reported that TGS shows the inhibitory effect on the CA release evoked by stimulation of nicotinic receptors in the perfused adrenal medulla of rats [4] and SHRs [5], and also that several ginseng saponins (ginsenosides) from the ginseng root inhibit the CA secretion from bovine adrenal chromaffin cells stimulated by ACh [6789].
Moreover, it has been known that ginsenosides or ginseng extracts cause the hypotensive action [1011121314] while they can rather produces the hypertensive action [15161718]. TGS is found to produce the pressor and depressor actions in the anesthetized normotensive rats [19].
Furthermore, ginseng, when given at small dose in spontaneously hypertensive rat (SHR), causes pressor response, but at relatively large dose rather produces dose-dependent hypotensive response with decreased plasma renin activity [202122]. Sokabe and his coworkers [23] have shown that administration of Korean Red ginseng powder for 11 weeks has no effect on blood pressure in normotensive Donryu (DON) rats, SHR and renal hypertensive rats, whereas it elevates slightly blood pressure in deoxycorticosterone salt hypertensive rats. Recently, it has also been reported that long-term use of North American ginseng had no effect on 24-hour BP and renal function in hypertensive individuals [24].
In the conscious rats, the releasing effect of NO of KRG may be partly contributed to the hypotensive effect of KRG [12]. It has also been shown that Rg3 relaxes the rat thoracic aorta as a consequence of NO production [25]. Han and his co-workers [26] have found evidence that NO levels in exhaled breath of human volunteers by KRG were increased along with decreased blood pressure and heart rate. In a series of studies, it has been found that ginsenosides reduce blood pressure via increases in production of endothelial nitric oxide [10] and that Rg3 is the most potent ginsenoside that activates eNOS in rat aorta [27]. Although some investigators have demonstrated that Rg3 induces eNOS activation in the vasculature of animal models [1028], Hien and his co-workers [29] have found that Rg3 activates eNOS via eNOS phosphorylation in ECV 304 human endothelial cells and increases in eNOS expression. Meanwhile, some studies have shown that ginseng contains a novel G protein-coupled lysophosphatidic acid (LPA) receptor ligand, known as gintonin [303132]. Recently, it has been reported that treatment of gintonin to PC12 cells increased intracellular calcium ([Ca2+]i) as well as the release of dopamine in a concentration-dependent manner [33]. It was concluded that this gintonin-mediated [Ca2+]i elevation is coupled to dopamine release via LPA receptor activation.
Despite of these many studies, there is still controversy about the functional effects of ginsenosides on the cardiovascular system, especially blood pressure and adrenal CA secretion. Therefore, the aim of the present study was to investigate the ability of gintonin, known as a novel G protein-coupled LPA receptor ligand, on the CA secretion in the perfused model of rat adrenal glands, and to establish its mechanism of action.
All procedures involving animal experiments were conducted in strict accordance with the guidance for the care and use of Laboratory Animals of the National Institutes of Health (NIH Publications No. 80-23; revised in 1996), and approved by the Committee of Experimental Animals, School of Medicine, Chosun University.
Male Sprague-Dawley rats, weighing 180 to 250 grams, were anesthetized with thiopental sodium (40 mg/kg) intraperitoneally. The adrenal gland was isolated by the methods described previously [34]. The abdomen was opened by a midline incision, and the left adrenal gland and surrounding area were exposed by placing three hook retractors. The stomach, intestine and portion of the liver were not removed, but pushed over to the right side and covered by saline-soaked gauge pads and urine in bladder was removed in order to obtain enough working space for tying blood vessels and cannulations.
A cannula, used for perfusion of the adrenal gland, was inserted into the distal end of the renal vein after all branches of adrenal vein (if any), vena cava and aorta were ligated. Heparin (400 IU/ml) was injected into vena cava to prevent blood coagulation before ligating vessels and cannulations. A small slit was made into the adrenal cortex just opposite entrance of adrenal vein. Perfusion of the gland was started, making sure that no leakage was present, and the perfusion fluid escaped only from the slit made in adrenal cortex. Then the adrenal gland, along with ligated blood vessels and the cannula, was carefully removed from the animal and placed on a platform of a leucite chamber. The chamber was continuously circulated with water heated at 37 ±1oC.
The adrenal glands were perfused by means of ISCO pump (WIZ Co.) at a rate of 0.33 ml/min. The perfusion was carried out with Krebs-bicarbonate solution of following composition (mM): NaCl, 118.4; KCl, 4.7; CaCl2, 2.5; MgCl2, 1.18; NaHCO3, 25; KH2PO4, 1.2; glucose, 11.7. The solution was constantly bubbled with 95% O2+5% CO2 and the final pH of the solution was maintained at 7.4~7.5. The solution contained disodium EDTA (10 µg/ml) and ascorbic acid (100 µg/ml) to prevent oxidation of catecholamine.
Perfusion of gintonin (1~30 µg/ml) for 4 min or single injection of acetylcholine (5.32 mM) in a volume of 0.05 ml were made into perfusion stream via a three way stopcock. In the preliminary experiments it was found that upon administration of the above drugs, secretory responses to gintonin and acetylcholine returned to preinjection level in 4 min. Generally, the adrenal glands were perfused with normal Krebs solution for about one hour before the experimental protocols are initiated.
As a rule, prior to stimulation with gintonin, samples were collected (4 min) to determine the spontaneous secretion of CA ("background sample"). Immediately after the collection of the "background sample", collection of the perfusate was continued in another tube as soon as the perfusion medium containing gintonin reached the adrenal gland. Each perfusate was collected for 4 min at 15 min interval. The amounts secreted in the "background sample" have been subtracted from those from secreted the "stimulated sample" to obtain the net secretion value of CA, which is shown in all of the figures.
To study the effect of a test agent on the spontaneous and drug-evoked secretion, the adrenal gland was perfused with Krebs solution containing the agent for 90 min. Then the perfusate was collected for a specific time period ("background sample"), and then the medium was changed to the one containing the test agent or along with a specific antagonist, and the perfusate were collected for the same period as that for the "background sample". The adrenal perfusate was collected in chilled tubes.
CA content of perfusate was measured directly by the fluorometric method of Anton and Sayre [35] without the intermediate purification alumina for the reasons described earlier [34] using fluorospectrophotometer (Kontron Co. Italy).
A volume of 0.2 ml of the perfusate was used for the reaction. The CA content in the perfusate of stimulated glands by secretagogues used in the present work was high enough to obtain readings several folds greater than the reading of control samples (unstimulated). The sample blanks were also lowest for perfusates of stimulated and non-stimulated samples. The content of CA in the perfusate was expressed in terms of norepinephrine (base) equivalents.
The statistical significance between groups was determined by the Student's t- and ANOVA- tests. A p-value of less than 0.05 was considered to represent statistically significant changes unless specifically noted in the text. Values given in the text refer to means and the standard errors of the mean (S.E.M.). The statistical analysis of the experimental results was made by computer program described by Tallarida and Murray [36].
The following drugs were used: acetylcholine chloride, norepinephrine bitartrate, nicardipine hydrochioride, amiloride hydrochloride hydrate, 3.4.5-trimethoxy benzoic acid 8-(diethylamino) octylester (TMB-8), pirenzepine hydrochloride, ethyleneglycol tetraacetic acid (EGTA), Ki16425, and Oleoyl-L-α-lysophosphatidic acid sodium salt from Sigma-Aldrich Chemical Co., U.S.A., and chlorisondamine chloride from Ciba Co., U.S.A. Gintonin, devoid of ginseng saponins, was prepared from Panax ginseng according to previously described methods [32], and supplied by professor Seung-Yeol Nah who is a professor of Konkuk University, Seoul, Korea. Gintonin was dissolved in deionized water and then diluted with Krebs solution before use. LPA and Ki16425 was dissolved in DMSO and then diluted with Krebs solution before use. Drugs were dissolved in distilled water (stock) and added to the normal Krebs solution as required. Concentrations of all drugs used are expressed in terms of molar base.
When the adrenal gland was perfused with oxygenated Krebs-bicarbonate solution for 60 min before experimental protocol is initiated, the spontaneous CA secretion reached steady state. The basal CA release from the perfused rat adrenal medulla amounted to 21±2 ng for 2 min from 10 experiments. The releasing effects to the perfusion of gintonin for 4 min are shown in Fig. 1 (upper). Infusion of gintonin (1~30 µg/mL) into the perfusion stream for 4 min at 15 min-intervals gradually dose-dependently exerted significant CA secretory responses from 149±21 ng to 1451±43 ng over the background release. These observations are largely consistent with results described previously that ginseng saponins cause the increased CA secretion from the isolated perfused rabbit adrenal glands [123] while rather opposite to the inhibitory effects on the CA secretion in rat adrenal gland [4] and SHRs [5], and in bovine adrenal chromaffin cells [67937].
In order to examine the tachyphylaxis to gintonin-evoked CA releasing effects, gintonin at the concentration of 10 µg/mL was given into the perfusion stream for 4 min seven times consecutively at 15 min-intervals, respectively. In 6 rat adrenal glands, there was no statistically significant difference in CA secretion (1344±29 ng~1451±43 ng) between periods (Fig. 1-upper). Therefore, in all subsequent experiments, 10 µg/mL of gintonin was used.
Since the physiological secretion of CA and dopamine-β-hydroxylase from the perfused cat adrenal gland is found to be dependent on the extracellular calcium concentration [38], it was of particular interest to test whether gintonin-induced CA secretory effect is also associated to extracellular calcium ions. Thus, the adrenal gland was perfused with calcium-free Krebs solution containing 5×10–3 M EGTA for 20 min prior to introduction of gintonin. Under the perfusion of calcium-free Krebs solution containing EGTA in 6 rat glands, gintonin (10 µg/mL)-evoked CA secretory responses were significantly inhibited to from 73±2% (0~4 min, p<0.01) to 23±1% (75~94 min, p<0.01) of the corresponding control response (1109±26 ng/0~4 min), as shown in Fig. 1 (lower).
In order to investigate the effect of nicardipine, which is known to be a voltage-dependent L-type Ca2+ channel blocker and a dihydropyridine derivative [39], on gintonin-evoked CA secretion, nicardipine (10–6 M) was preloaded into the adrenal gland for 20 min. In the presence of nicardipine effect, gintonin (10 µg/mL)-induced CA was markedly reduced from 73±2% (0~4 min, p<0.01) to 23±1% (75~94 min, p<0.01), as compared with the corresponding control response (1024±40 ng/0~4 min) in 5 rat glands. Fig. 2 (upper) shows that nicardipine inhibits gintonininduced CA secretory responses relatively in time-dependent fashion.
It has been reported that muscarinic, but not nicotinic activation causes CA secretion independent of extracellular calcium in the perfused cat adrenal glands [40]. It suggests that the presence of an intracellular calcium pool is linked to muscarinic receptors, and that TMB-8, an intracellular calcium antagonist, inhibits both nicotinic and muscarinic stimulation-induced CA release in the rat adrenal glands [41]. Therefore, it was attempted to examine the effects of TMB-8 on gintonin-induced CA secretion. In 8 rat adrenal glands, gintonin (10 µg/mL)-induced CA secretion after preloading with TMB-8 (10 µM) for 20 min were greatly reduced from 88±2% (0~4 min, p<0.01) to 24±2% (75~94 min, p<0.01) in comparison with their corresponding control response (1056±21 ng/0~4 min), as shown in Fig. 2 (lower).
It was found that gintonin-evoked CA secretion is greatly inhibited by treatment with Ca2+-free Krebs, nicardipine or TMB-8, respectively. To examine the involvement of Na+ channel, Na+/Ca2+ exchanger and Na+/H+ exchanger in gintonin-induced increase in CA secretion, adrenal glands were treated with their specific inhibitor. Perfusion of adrenal gland with amiloride, which is known Na+/Ca2+ exchange inhibitors [4243], for 90 min caused a significant decrease in gintonin-induced CA secretion, as shown in Fig. 3 (upper). In the presence of amiloride (1 mM), gintonin (10 µg/mL)-induced CA secretion was markedly reduced from 78±4% (0~4 min, p<0.01) to 52±3% (75~94 min, p<0.01) relatively in time-dependent fashion, as compared with the corresponding control response (1120±21 ng/0~4 min) from 6 rat adrenal glands.
In 5 rat adrenal glands, to study the relationship between NO and gintonin-induced CA secretion, sodium nitroprusside (100 µM) was perfused into the adrenal gland for 90 min. In the presence of sodium nitroprusside, gintonin (10 µg/mL)-induced CA secretory response was not affected as compared with its control secretion as shown in Fig. 3 (lower). There was no statistical significance in difference between the gintonin-evoked CA secretory responses before and after pretreatment with nitroprusside.
In order to certify the effect of chlorisondamine, a selective neuronal nicotinic receptor antagonist, on gintonin-induced CA release, the rat adrenal gland was loaded with chlorisondamine (1 µM) for 90min. In the presence of chlorisondamine, gintonin (10 µg/mL)-induced CA seretion were greatly inhibited from 57±2 ng (0~4 min) to 23±1 ng (90~94 min) of the control release (1120±20 ng/0~4 min) from 8 glands as shown in Fig. 4 (upper).
In order to observe the effect of muscarinic receptors, it would be interesting to examine the effect of pirenzepine on gintonin-induced CA secretion. In the present work, gintonin-induced CA output was significantly reduced in the rat adrenal gland preloaded with pirenzepine (2 µM) for 90 min. In 8 rat adrenal glands, gintonin (10 µg/mL)-induced CA secretory responses after treatment with pirenzepine were greatly inhibited to 86~23% of the control release (1120±20 ng/0~4 min) as shown in Fig. 4 (lower).
Since it has been previously reported that ginseng contains a novel G protein-coupled lysophosphatidic acid (LPA) receptor ligand, known as gintonin [303132], it is very interesting to examine the effect of LPA receptor antagonist on gintonin-induced CA secretory response. As shown in Fig. 5 (upper), in the presence of Ki16425 (10 µM), selective antagonist of LPA receptors [44], gintonin(10 µg/mL)-induced CA secretory responses were significantly inhibited to 85±4% (15~64 min, p<0.01) and 76±1% (75~94 min, p<0.01) as compared to their control secretion (832±37 ng/0~4 min). Moreover, in order to examine whether LPA can evoked CA secretion from the perfused art adrenal medulla, perfusion of LPA (0.3~3.0 µM) into the adrenal gland for 4 min at 15 min-intervals greatly enhanced the CA secretion from 683±27 ng (0.3 µM) to 2194±18 ng (3.0 µM) over the background release (21±2 ng) in a dose-dependent fashion (Fig. 5-lower). However, in the presence of Ki16425 (10 µM), LPA (1 µM)-induced CA secretory responses were markedly reduced to 85±4% (p<0.01)~57±4% (p<0.01) as compared to their control secretion (1225±26 ng/0~4 min) as shown in Fig. 6 (upper).
In terms of the facts that gintonin-induced CA release was markedly inhibited by the pretreatment with chlorisondamine and pirenzepine as in Fig. 4, it was of interest to examine the influence of gintonin perfusion on ACh-induced CA secretory responses. The adrenal gland was perfused with gintonin (10 µg/mL) for 90 min. In the presence of gintonin, ACh-induced CA output was greatly enhanced to 128±2% (0~49 min, p<0.01), 124±1% (60~64 min, p<0.01) and 120±2% (75~94 min, p<0.01) as shown in Fig. 6 (lower).
This is the first report demonstrating that gintonin greatly enhances CA secretion in a calcium-dependent fashion from the isolated perfused model of the rat adrenal medulla through activation of LPA (LPA1 and LPA3) receptors as well as neuronal cholinergic (nicotinic and muscarinic) receptors located on the rat adrenomedullary chromaffin cells.
In the present study, gintonin-evoked CA secretory responses were significantly inhibited in the presence of Ki16425, the LPA1/3 receptor antagonist. Moreover, LPA also greatly caused the facilitation of CA secretion in the perfused rat adrenal medulla in a dose-dependent fashion. However, this LPA-induced CA secretion was also markedly impeded in the presence of Ki16425. It is well-known that Ki16425 selectively inhibits LPA receptor-mediated actions, especially through LPA1 and LPA3; therefore, it may be useful in evaluating the role of LPA and its receptor subtypes involved in biological actions [44]. Therefore, these results indicate that gintonin-evoked CA secretion might be at least due to activation of LPA receptors located on adrenomedullary chromaffin cells.
In support of this idea, gintonin is found to be a novel G protein-coupled LPA receptor ligand [303132]. More recently, it has been shown that gintonin-mediated intracellular Ca2+ transients and dopamine release via LPA receptor activation in PC12 cells could be one mechanism of gintonin-mediated inter-neuronal modulation in the nervous system [33]. Furthermore, LPA is also known to facilitate dopamine release from PC12 cells, indicating that LPA stimulates dopamine release via specific LPA receptors presumably by activating the IP3 mediated Ca2+ release [45]. Also, Pan and his co-workers suggested the differential effects of LPA and S1P in releasing Ca2+ from the intracellular Ca2+ stores and modulating the stimulus-secretion coupling in bovine chromaffin cells [46]. In view of these findings, the present study demonstrates that gintonin can enhance the CA secretion from the perfused rat adrenal medulla through activation of LPA1/3 receptors.
In the present work, upon the repeated administration of gintonin at 15 min intervals into the perfused rat adrenal gland, the CA secretory response was no changed. Tachyphylaxis to releasing effects of CA evoked by gintonin was not observed by the repeated administration of concentrations used in this study.
Generally, the adrenal medulla has been employed as the model system to study numerous cellular functions involving not only noradrenergic nerve cells but also neurons. One of such functions is neurosecretion. During the neurogenic stimulation of the adrenal medulla, ACh is released from the splanchnic nerve endings and activated cholinergic receptors on the chromaffin cell membrane [47]. This activation triggers a series of events known as stimulus-secretion coupling, culminating in the exocytotic release of CA and their components of the secretory vesicles into the extracellular space. ACh, the physiological presynaptic transmitter at the adrenal medulla, releases CA and dopamine-β-hydroxylase by calcium-dependent secretory process [3848].
In the present work, the gintonin-induced release of CA seems to be due to exocytosis of CA storage vesicles subsequent through activation of nicotinic ACh receptors in the rat adrenomedullary chromaffin cells, because it was greatly abolished in the presence of chlorisondamine. Chlorisondamine is known to be a selective antagonist of neuronal nicotinic cholinergic receptors [39]. Also, in this study, the gintonin-evoked CA secretory response was significantly inhibited in the presence of pirenzepine, which is a selective antagonist of muscarinic M1-receptors. This finding indicates that gintonin-evoked CA release is mediated at least by stimulation of muscarinic ACh receptors. In general, subtypes of muscarinic receptors have been recognized in many tissues [49]. Receptor binding studies have supported the classification of muscarinic receptors into M1 and M2 on the basis of the selectivity profile of pirenzepine; receptors with a high affinity for pirenzepine are designated as M1 and those with low affinity as M2 receptors [5051]. Doods and his colleagues [52] have classified muscarinic receptors into M1 (pirenzepine sensitive, neuronal), M2 (cardiac) and M3 (smooth muscle and glandular). It has been shown that that combination of extracts of ginseng and ginkgo biloba significantly increased the level of ACh in whole brain of amyloid beta-protein treated rats [53]. This ginseng-induced improvement of behavioral impairment in amyloid beta-protein treated rats seems to be due to the increased ACh level, which results from the decreased AChE activities [53]. Su and his coworkers [54] have suggested that ginseng root has the ability to increase the release of ACh from nerve terminals in wild rats so as to stimulate muscarinic receptors activity located in the pancreatic cells for the secretion of insulin, which in turn lower plasma glucose. More recently, Kim and his coworkers [55] reported that activation of G protein-coupled LPA receptors by gintonin is coupled to the regulation of cholinergic functions. Furthermore, they also found that gintonin could be a novel agent for the restoration of cholinergic system damages due to amyloid beta-protein and could be utilized for prevention or therapy of Alzheimer's disease. In view of above studies, gintonin-evoked CA release seems to be mediated partly through activation of nicotinic as well as muscarinic receptors in the perfused rat adrenal medulla.
In the present study, gintonin-evoked CA releasing effect was not influenced in presence of sodium nitroprusside, known as an NO donor. This result demonstrates that gintonin-evoked CA secretion is not relevant to NO release in the perfused rat adrenal medulla.
The indispensable role of calcium in the neurosecretory process has been well established. Calcium is well-known to play the crucial role in the process of depolarization-neurotransmitter release coupling in many types of secretory cells [565758]. Furthermore, it has been found that nicotinic (but not muscarinic) stimulation also releases soluble ACh from the chromaffin cells by a calcium-dependent mechanism [59]. The activation of nicotinic receptors stimulates CA secretion by increasing Ca2+ entry through receptor-linked and/or voltage-dependent Ca2+ channels in the perfused rat adrenal glands [60] and isolated bovine adrenal chromaffin cells [616263].
In the present study, removal of extracellular Ca2+ markedly depressed gintonin-evoked CA secretion. Furthermore, treatment of nicardipine, a dihydropyridine derivative and L-type Ca2+ channel blocker, also blocked the gintonin-evoked CA release. The secretory effect of gintonin seems to be apparently dependent on extracellular Ca2+. However, in the present study, the reason for considerable response to gintonin in Ca2+ -free Krebs plus EGTA solution as well as in the presence of nicardipine is not clear. However, in the presence of TMB-8, an inhibitor of the intracellular Ca2+ store, gintonin-evoked CA secretion was greatly inhibited in this perfused rat adrenal medulla. This result indicates that gintonin-evoked CA secretion is associated with intracellular Ca2+ release from endoplasmic reticulum (Ca2+ store) of the rat adrenal chromaffin cells. In support of this notion, TMB-8 is also known to inhibit caffeine-induced 45Ca2+ release from, but not the uptake of 45Ca2+ in isolated bovine adrenomedullary cells [6465]. Moreover, it has been shown that caffeine-evoked CA secretion is also inhibited from the perfused adrenal gland of the cat [66] and the rat [67] in the absence of extracellular calcium. TMB-8 reduced the resting [Ca2+]i and attenuated histamine-, 5-HT-, and glutamate-induced increases of [Ca2+]i in basilar artery smooth [68] as well as in brain cells [69]. TMB-8 is reported to inhibit the Ca2+ release from intracellular stores or increased the up-take of Ca2+ into sarcoplasmic reticulum and the inhibition of Ca2+-influx from extracellular site may be an indirect mechanism [70]. Recently, it has also been shown that gintonin-mediated [Ca2+]i transients are coupled to the stimulation of dopamine release in PC12 cells [33]. Thus, in light of these findings, our result shows that gintonin can trigger Ca2+ release from the intracellular Ca2+ store of the rat adrenomedullary chromaffin cells that participates in the CA secretion. Such a store may not be easily depleted by mere removal of extracellular calcium or voltage-dependent L-type Ca2+ channel blocker.
In the present study, in the presence of gintonin (continuous infusion), ACh-evoked CA secretion were significantly potentiated. This result indicates that mechanism of gintonin-evoked CA secretion is quite different from that of Ach-evoked CA secretion.
In the present study, gintonin-evoked CA secretion was clearly shown to be inhibited markedly by treatment of arniloride in the perfused rat adrenal medulla. This result suggests that the CA secretion evoked by gintonin is probably due to the activation of Na+/Ca2+ exchange process, resulting from the inhibition of Na+/K+-ATPase in nerve endings. In support of this idea, it has already been reported that amiloride inhibits Na+/Ca2+ exchanger in various cells and tissues [717273]. In view of these findings, amiloride is considered to inhibit gintonin-evoked CA secretion, presumably through its blocking action on the Na+/Ca2+ exchange system, which may be stimulated by the elevation of intracellular Na+ concentration, resulting from the inhibition of Na+/K+-ATPase in the plasma membranes. Moreover, LPA has also been found to stimulate extracellular Na+-dependent 45Ca2+ efflux from cultured bovine adrenal chromaffin cells, probably through its stimulatory effect on membrane Na+/Ca2+ exchange [74]. In light of these findings, gintonin-evoked CA secretory response seems to be relevant to activation of Na+/Ca2+ exchange in the rat adrenal chromaffin cells.
However, in contrast to above the described results, Pan and his coworkers [75] have found that both sphingosine-1-phosphate and LPA inhibit inward Ca2+ current in a concentration-dependent manner in isolated bovine chromaffin cells. They also showed that LPLs reduce repetitive firing of action potentials (Aps) and inhibit both exocytosis and endocytosis, indicating that S1P and LPA at physiological concentrations attenuate the excitability of chromaffin cells and hint at the possible blockade of CA secretion induced by sympathetic neurons. Therefore, it seems that there is some difference between cultured bovine chromaffin cells and perfused rat adrenal medulla in exocytosis evoked LPA and gintonin. Further experiments will be pursued to characterize how gintonin and LPLs modulate the exocytosis, especially in the isolated perfused model of the rat adrenal medulla in near future.
Previously, it has been reported that some ginsenosides, including Rb2 and Rg3 as well as total ginseng saponin inhibit CA release evoked by stimulation of cholinergic receptors through inhibition of influx of Na+ and Ca2+ as well as reduction of intracellular Ca2+ release from its store, which is relevant to elevation of NO release [456789]. Whereas gintonin known as a novel G protein-coupled LPA receptor ligand [303132] enhances CA release through facilitation of influx of Na+ and Ca2+ as well as intracellular Ca2+ release via stimulation of cholinergic and LPA receptors. Thus, gintonin-mediated regulation of CA release is quite different from that of ginsenosides in the perfused rat adrenal medulla. Future studies will remained to be elucidated what the clinical roles of these two components are in their differential regulation of CA release.
As shown in Fig. 7, taken together, these results demonstrate the first evidence that gintonin increases the CA secretion from the perfused rat adrenal medulla in a dose-dependent fashion. This facilitatory effect of gintonin seems to be associated with activation of LPA- and cholinergic-receptors, which are relevant to the cytoplasmic Ca2+ increase by stimulation of the Na+ and Ca2+ influx as well as by the inhibition of Ca2+ uptake into the cytoplasmic Ca2+ stores, without the increased nitric oxide (NO). Based on these results, it is thought that gintonin, one of ginseng components, can elevate the CA secretion from adrenal medulla by regulating the Ca2+ mobilization for exocytosis, suggesting facilitation of cardiovascular effects. Also, these findings demonstrate that gintonin might be at least one of ginseng-induced hypertensive components.
ACKNOWLEDGEMENTS
This study was supported partly by a research grant from Chosun University, Gwangju, Korea (2013~2014).
Notes
Author contributions: D.Y.L., S.Y.N., and K.H.K. designed. M.S.C. and D.Y.L. carried out the experiments. K.H.K. and M.S.C. acquired data. S.Y.N. and D.Y.L. analyzed and interpreted data. K.H.K. drafted the article. M.S.C. performed statistical analysis. S.Y.N. and D.Y.L. supervised and coordinated the study. D.Y.L. wrote the manuscript.
References
1. Lim DY, Park KB, Kim KH, lee KS, Moon JK, Kim YH. Influence of total ginseng saponin on secretion of catecholamines in the isolated adrenal gland of rabbits. Korean Biochem J. 1987; 20:230–238.
2. Lim DY, Park KB, Kim KH, Choi CH, Bae JW, Kim MW. Studies on secretion of catecholamines evoked by panaxadiol in the isolated rabbit adrenal gland. Korean J Pharmacol. 1988; 24:31–42.
3. Lim DY, Choi CH, Kim CD, Kim KH, Kim SB, Lee BJ, Chung MH. Influnce of panaxatriol-type saponin on secretion of catecholamine from isolated perfused rabbit adrenal gland. Arch Pharm Res. 1989; 12:166–175.
4. Hong SP, Chi H, Cho SH, Lee YK, Woo SC, Kim IS, Oh SH, Yang WH, Lim DY. Influence of total Ginseng saponin on nicotinic stimulation-induced catecholamine secretion from the perfused rat adrenal gland. J Korean Soc Hypertens. 1999; 5:159–168.
5. Jang SJ, Lim HJ, Lim DY. Inhibitory Effects of Total Ginseng Saponin on Catecholamine Secretion from the Perfused Adrenal Medulla of SHRs. J Ginseng Res. 2011; 35:176–190. PMID: 23717060.


6. Kudo K, Akasaka Y, Miyate Y, Takahashi E, Tachikawa E, Kashimoto T. Effects of red ginseng fractions on catecholamine secretion from bovine adrenal medullary cells. J Med Pharm Soc Wakan-Yaku. 1992; 9:236–239.
7. Tachikawa E, Kudo K, Kashimoto T, Takahashi E. Ginseng saponins reduce acetylcholine-evoked Na+ influx and catecholamine secretion in bovine adrenal chromaffin cells. J Pharmacol Exp Ther. 1995; 273:629–636. PMID: 7752064.
8. Kudo K, Tachikawa E, Kashimoto T, Takahashi E. Properties of ginseng saponin inhibition of catecholamine secretion in bovine adrenal chromaffin cells. Eur J Pharmacol. 1998; 341:139–144. PMID: 9543231.


9. Tachikawa E, Kudo K, Nunokawa M, Kashimoto T, Takahashi E, Kitagawa S. Characterization of ginseng saponin ginsenoside-Rg(3) inhibition of catecholamine secretion in bovine adrenal chromaffin cells. Biochem Pharmacol. 2001; 62:943–951. PMID: 11543730.
10. Kim ND, Kang SY, Schini VB. Ginsenosides evoke endotheliumdependent vascular relaxation in rat aorta. Gen Pharmacol. 1994; 25:1071–1077. PMID: 7875528.


11. Han KH, Choe SC, Kim HS, Sohn DW, Nam KY, Oh BH, Lee MM, Park YB, Choi YS, Seo JD, Lee YW. Effect of red ginseng on blood pressure in patients with essential hypertension and white coat hypertension. Am J Chin Med. 1998; 26:199–209. PMID: 9799972.


12. Jeon BH, Kim CS, Kim HS, Park JB, Nam KY, Chang SJ. Effect of Korean red ginseng on blood pressure and nitric oxide production. Acta Pharmacol Sin. 2000; 21:1095–1100. PMID: 11603282.
13. Jeon BH, Kim CS, Park KS, Lee JW, Park JB, Kim KJ, Kim SH, Chang SJ, Nam KY. Effect of Korea red ginseng on the blood pressure in conscious hypertensive rats. Gen Pharmacol. 2000; 35:135–141. PMID: 11744235.


14. Sung J, Han KH, Zo JH, Park HJ, Kim CH, Oh BH. Effects of red ginseng upon vascular endothelial function in patients with essential hypertension. Am J Chin Med. 2000; 28:205–216. PMID: 10999439.


15. Siegel RK. Ginseng abuse syndrome. Problems with the panacea. JAMA. 1979; 241:1614–1615. PMID: 430716.


16. Baldwin CA, Anderson LA, Phillipson JA. What pharmacists should know about ginseng. Pharm J. 1986; 237:583–586.
17. Miller LG. Herbal medicinals: selected clinical considerations focusing on known or potential drug-herb interactions. Arch Intern Med. 1998; 158:2200–2211. PMID: 9818800.
18. Klepser TB, Klepser ME. Unsafe and potentially safe herbal therapies. Am J Health Syst Pharm. 1999; 56:125–138. PMID: 10030529.


19. Lim DY, Park KB, Kim KH, Moon JK, Lee KS, Kim YK, Chung YH, Hong SP. Influnce of total ginseng saponin on the blood pressure of the rat. Korean Ciru J. 1987; 17:491–499.
20. Sohn ES, Park SC, Huh BY, Lee CK, Rhim HK, Ham JS, Yang CM, Han CS, Park CW, Kim HJ. An animal experiental study on the effect of Ginseng on blood pressure and plasma renin activity in spontaneously hypertensive rat. J Korean Med Assoc. 1979; 22:731–746.
21. Sohn ES, Park SC, Huh BY, Lee DH, Rhim HK, Young CM, Han CS, Song BS Kim, SJ , Park CW, Kim HJ. An animal experimental study of the effect of Korean Ginseng on body weight and blood pressure in spontaneously hypertensive rat with oral administration. J Korean Med Assoc. 1980; 23:37–48.
22. Seok SE, Park CH, Nam SH, Choi HS, Lee JI, Lee DH, Huh BY, Soh ES. An experimental study on the antihypertensive effects of Korea Ginseng to spontanously rats (SHR) in labile stage of hypertension. J Korean Med Assoc. 1981; 24:509–515.
23. Sokabe H, Kishi K, Watanabe TX. Effects of Korean red ginseng powder administered orally on blood pressure in hypertensive rats. Proceeding of the 4th international ginseng symposium. Seoul: Korea Ginseng Research Institute;1984. p. 57.
24. Stavro PM, Woo M, Leiter LA, Heim TF, Sievenpiper JL, Vuksan V. Long-term intake of North American ginseng has no effect on 24-hour blood pressure and renal function. Hypertension. 2006; 47:791–796. PMID: 16520410.


25. Kim ND, Kim EM, Kang KW, Cho MK, Choi SY, Kim SG. Ginsenoside Rg3 inhibits phenylephrine-induced vascular contraction through induction of nitric oxide synthase. Br J Pharmacol. 2003; 140:661–670. PMID: 14534150.


26. Han K, Shin IC, Choi KJ, Yun YP, Hong JT, Oh KW. Korea red ginseng water extract increases nitric oxide concentrations in exhaled breath. Nitric Oxide. 2005; 12:159–162. PMID: 15797844.


27. Kim ND, Kang SY, Park JH, Schini-Kerth VB. Ginsenoside Rg3 mediates endothelium-dependent relaxation in response to ginsenosides in rat aorta: role of K+ channels. Eur J Pharmacol. 1999; 367:41–49. PMID: 10082263.
28. Kang SY, Schini-Kerth VB, Kim ND. Ginsenosides of the protopanaxatriol group cause endothelium-dependent relaxation in the rat aorta. Life Sci. 1995; 56:1577–1586. PMID: 7723586.


29. Hien TT, Kim ND, Pokharel YR, Oh SJ, Lee MY, Kang KW. Ginsenoside Rg3 increases nitric oxide production via increases in phosphorylation and expression of endothelial nitric oxide synthase: essential roles of estrogen receptor-dependent PI3-kinase and AMP-activated protein kinase. Toxicol Appl Pharmacol. 2010; 246:171–183. PMID: 20546771.


30. Hwang SH, Shin TJ, Choi SH, Cho HJ, Lee BH, Pyo MK, Lee JH, Kang J, Kim HJ, Park CW, Shin HC, Nah SY. Gintonin, newly identified compounds from ginseng, is novel lysophosphatidic acidsprotein complexes and activates G protein-coupled lysophosphatidic acid receptors with high affinity. Mol Cells. 2012; 33:151–162. PMID: 22286231.


31. Nah SY, Kim DH, Rhim H. Ginsenosides: are any of them candidates for drugs acting on the central nervous system? CNS Drug Rev. 2007; 13:381–404. PMID: 18078425.


32. Pyo MK, Choi SH, Hwang SH, Shin TJ, Lee BH, Lee SM, Lim YH, Kim DH, Nah SY. Novel glycoproteins from ginseng. J Ginseng Res. 2011; 35:92–103.
33. Hwang SH, Lee BH, Choi SH, Kim HJ, Jung SW, Kim HS, Shin HC, Park HJ, Park KH, Lee MK, Nah SY. Gintonin, a novel ginsengderived lysophosphatidic acid receptor ligand, stimulates neurotransmitter release. Neurosci Lett. 2015; 584:356–361. PMID: 25445364.


34. Wakade AR. Studies on secretion of catecholamines evoked by acetylcholine or transmural stimulation of the rat adrenal gland. J Physiol. 1981; 313:463–480. PMID: 7277230.


35. Anton AH, Sayre DF. A study of the factors affecting the aluminum oxide-trihydroxyindole procedure for the analysis of catecholamines. J Pharmacol Exp Ther. 1962; 138:360–375. PMID: 14013351.
36. Tallarida RJ, Murray RB. Manual of pharmacologic calculation with computer programs. 2nd ed. New York: Speringer-Verlag;1987. p. 132.
37. Tachikawa E, Kudo K, Hasegawa H, Kashimoto T, Sasaki K, Miyazaki M, Taira H, Lindstrom JM. In vitro inhibition of adrenal catecholamine secretion by steroidal metabolites of ginseng saponins. Biochem Pharmacol. 2003; 66:2213–2221. PMID: 14609746.


38. Dixon WR, Garcia AG, Kirpekar SM. Release of catecholamines and dopamine beta-hydroxylase from the perfused adrenal gland of the cat. J Physiol. 1975; 244:805–824. PMID: 1133780.


39. Hardman JG, Limbird LE, Molinoff PB, Ruddon RR, Gilman AG. Goodman & Gilman's the pharmacological basis of therapeutics. 9th ed. New York: McGrraw-Hill;1995. p. 193–195.
40. Nakazato Y, Ohga A, Oleshansky M, Tomita U, Yamada Y. Voltage-independent catecholamine release mediated by the activation of muscarinic receptors in guinea-pig adrenal glands. Br J Pharmacol. 1988; 93:101–109. PMID: 3349226.


41. Lim DY, Kim CD, Ahn GW. Influence of TMB-8 on secretion of catecholamines from the perfused rat adrenal glands. Arch Pharm Res. 1992; 15:115–125.


42. Yu L, Netticadan T, Xu YJ, Panagia V, Dhalla NS. Mechanisms of lysophosphatidylcholine-induced increase in intracellular calcium in rat cardiomyocytes. J Pharmacol Exp Ther. 1998; 286:1–8. PMID: 9655835.
43. Rathi SS, Saini HK, Xu YJ, Dhalla NS. Mechanisms of low Na+-induced increase in intracellular calcium in KCl-depolarized rat cardiomyocytes. Mol Cell Biochem. 2004; 263:151–162.
44. Ohta H, Sato K, Murata N, Damirin A, Malchinkhuu E, Kon J, Kimura T, Tobo M, Yamazaki Y, Watanabe T, Yagi M, Sato M, Suzuki R, Murooka H, Sakai T, Nishitoba T, Im DS, Nochi H, Tamoto K, Tomura H, Okajima F. Ki16425, a subtype-selective antagonist for EDG-family lysophosphatidic acid receptors. Mol Pharmacol. 2003; 64:994–1005. PMID: 14500756.


45. Shiono S, Kawamoto K, Yoshida N, Kondo T, Inagami T. Neurotransmitter release from lysophosphatidic acid stimulated PC12 cells: involvement of lysophosphatidic acid receptors. Biochem Biophys Res Commun. 1993; 193:667–673. PMID: 8390248.
46. Pan CY, Lee H, Chen CL. Lysophospholipids elevate [Ca2+]i and trigger exocytosis in bovine chromaffin cells. Neuropharmacology. 2006; 51:18–26. PMID: 16616768.
47. Viveros OH. Mechanism of secretion of catecholaminies from adrenal medulla. In : Blaschko H, Sayers G, Smith DA, editors. Handbook of physiology, Endocrinology. Vol VI, Sect 7, The adrenal gland. Washington DC: American physiological society;1975. p. 389–426. .
48. Viveros OH, Arqueros LC, Kirshner N. Release of catecholanines and dopamine beta-hydroxylase from the adrenal medulla. Lifr Sci. 1968; 7:609–618.
49. Eglen RM, Whiting RL. Muscarinic receptor subtypes: a critique of the current classification and a proposal for a working nomenclature. J Auton Pharmacol. 1986; 6:323–346. PMID: 3546321.


50. Hammer R, Berrie CP, Birdsall NJ, Burgen AS, Hulme EC. Pirenzepine distinguishes between different subclasses of muscarinic receptors. Nature. 1980; 283:90–92. PMID: 7350532.


51. Hammer R, Giachetti A. Muscarinic receptor subtypes: M1 and M2 biochemical and functional characterization. Life Sci. 1982; 31:2991–2998. PMID: 6897666.
52. Doods HN, Mathy MJ, Davidesko D, van Charldorp KJ, de Jonge A, van Zwieten PA. Selectivity of muscarinic antagonists in radioligand and in vivo experiments for the putative M1, M2 and M3 receptors. J Pharmacol Exp Ther. 1987; 242:257–262. PMID: 3612532.
53. Liu JX, Cong WH, Xu L, Wang JN. Effect of combination of extracts of ginseng and ginkgo biloba on acetylcholine in amyloid betaprotein-treated rats determined by an improved HPLC. Acta Pharmacol Sin. 2004; 25:1118–1123. PMID: 15339385.
54. Su CF, Cheng JT, Liu IM. Increase of acetylcholine release by Panax ginseng root enhances insulin secretion in Wistar rats. Neurosci Lett. 2007; 412:101–104. PMID: 17123721.


55. Kim H, Lee BH, Choi SH, Kim HJ1, Jung SW, Hwang SH, Rhim H, Kim HC, Cho IH, Nah SY. Gintonin stimulates gliotransmitter release in cortical primary astrocytes. Neurosci Lett. 2015; 603:19–24. PMID: 26191656.


56. Douglas WW. Stimulus-secretion coupling: the concept and clues from chromaffin and other cells. Br J Pharmacol. 1968; 34:451–474. PMID: 4882190.


57. Schulz I, Stolze HH. The exocrine pancreas: the role of secretagogues, cyclic nucleotides, and calcium in enzyme secretion. Annu Rev Physiol. 1980; 42:127–156. PMID: 6105844.


58. Williams JA. Regulation of pancreatic acinar cell function by intracellular calcium. Am J Physiol. 1980; 238:G269–G279. PMID: 6155077.


59. Mizobe F, Livett BG. Nicotine stimulates secretion of both catecholamines and acetylcholinesterase from cultured adrenal chromaffin cells. J Neurosci. 1983; 3:871–876. PMID: 6834109.


60. Wakade AR, Wakade TD. Contribution of nicotinic and muscarinic receptors in the secretion of catecholamines evoked by endogenous and exogenous acetylcholine. Neuroscience. 1983; 10:973–978. PMID: 6139771.


61. Kilpatrick DL, Slepetis R, Kirshner N. Ion channels and membrane potential in stimulus-secretion coupling in adrenal medulla cells. J Neurochem. 1981; 36:1245–1255. PMID: 6259284.


62. Kilpatrick DL, Slepetis RJ, Corcoran JJ, Kirshner N. Calcium uptake and catecholamine secretion by cultured bovine adrenal medulla cells. J Neurochem. 1982; 38:427–435. PMID: 7108549.


63. Knight DE, Kesteven NT. Evoked transient intracellular free Ca2+ changes and secretion in isolated bovine adrenal medullary cells. Proc R Soc Lond B Biol Sci. 1983; 218:177–199. PMID: 6135214.
64. Misbahuddin M, Isosaki M, Houchi H, Oka M. Muscarinic receptor-mediated increase in cytoplasmic free Ca2+ in isolated bovine adrenal medullary cells. Effects of TMB-8 and phorbol ester TPA. FEBS Lett. 1985; 190:25–28. PMID: 4043396.
65. Sasakawa N, Yamamoto S, Ishii K, Kato R. Inhibition of calcium uptake and catecholamine release by 8-(N,N-diethylamino)-octyl-3,4,5-trimethoxybenzoate hydrochloride (TMB-8) in cultured bovine adrenal chromaffin cells. Biochem Pharmacol. 1984; 33:4063–4067. PMID: 6508853.
66. Yamada Y, Teraoka H, Nakazato Y, Ohga A. Intracellular Ca2+ antagonist TMB-8 blocks catecholamine secretion evoked by caffeine and acetylcholine from perfused cat adrenal glands in the absence of extracellular Ca2+. Neurosci Lett. 1988; 90:338–342. PMID: 3419642.
67. Lim DY, Lee JH, Kim WS, Kim SB, Lee EH, Lee BJ, Ko ST. Studies on secretion of catecholamine evoked by caffeine from the isolated perfused rat adrenal gland. Arch Pharm Res. 1991; 14:55–67. PMID: 10319123.


68. Wang B, Zhang XQ, Liu TP, Xiao JG. 8-(N,N-diethylamino)-n-octyl-3,4,5-trimethoxybenzoate reduced [Ca2+]i elevation induced by histamine, serotonin, and glutamate in cultured calf basilar artery smooth muscle cells. Zhongguo Yao Li Xue Bao. 1998; 19:251–253. PMID: 10375737.
69. Zhang XQ, Wang B, Zhang MY, Xiao JG. Inhibitory effects of 8-(N,N-diethylamino)-n-octyl-3,4,5-trimethoxybenzoate (TMB-8) on intracellular Ca2+ elevated by neurotransmitters in brain cells. Zhongguo Yao Li Xue Bao. 1999; 20:893–896. PMID: 11270987.
70. Zhang XQ, Wang B, Zhang MY, Xiao JG. [Effect of TMB-8 on the increase of intracellular free Ca2+ induced by NE and BHQ in dissociated single rat brain cell]. Yao Xue Xue Bao. 1997; 32:726–730. PMID: 11596212.
71. Benos DJ. Amiloride: a molecular probe of sodium transport in tissues and cells. Am J Physiol. 1982; 242:C131–C145. PMID: 7039345.


72. Schellenberg GD, Anderson L, Swanson PD. Inhibition of Na+-Ca2+ exchange in rat brain by amiloride. Mol Pharmacol. 1983; 24:251–258. PMID: 6888368.
73. Siegl PK, Cragoe EJ Jr, Trumble MJ, Kaczorowski GJ. Inhibition of Na+/Ca2+ exchange in membrane vesicle and papillary muscle preparations from guinea pig heart by analogs of amiloride. Proc Natl Acad Sci U S A. 1984; 81:3238–3242. PMID: 6587348.
74. Houchi H, Okuno M, Tokumura A, Yoshizumi M, Fukuzawa K, Oka M. Lysophosphatidic acid as a stimulator of Na+-dependent Ca2+ efflux from adrenal chromaffin cells. Life Sci. 1995; 57:PL205–PL210. PMID: 7674825.
75. Pan CY, Wu AZ, Chen YT. Lysophospholipids regulate excitability and exocytosis in cultured bovine chromaffin cells. J Neurochem. 2007; 102:944–956. PMID: 17630986.


Fig. 1
Concentration-dependent effect of gintonin on secretion of catecholamines (CA) (upper panel) and the perfusion effect of Ca2+-free Krebs plus EGTA on gintonin-evoked CA secretion from the perfused rat adrenal glands (lower panel).
Gintonin (1~30 µg/ml) was infused into an adrenal vein for 4 min at 15 min intervals during 90 min. Perfusion of gintonin was made after perfusion with normal Krebs-bicarbonate solution for one hour before the experimental protocols were initiated. The data are expressed with mean±S.E. The statistical significance was compared between the periodic groups at each concentration. Abscissa: concentration of gintonin in M. The statistical difference was obtained by comparing gintonin-evoked CA secretory effect after treatment with Ca2+-free Krebs plus EGTA (5 mM) with its corresponding control. Ordinate: secretion of CA in ng or percentage for 4 min. The vertical columns and bars denote means and the standard errors, respectively. Number in the upper bracket indicates the number of animals used in the experiments. **p<0.01.
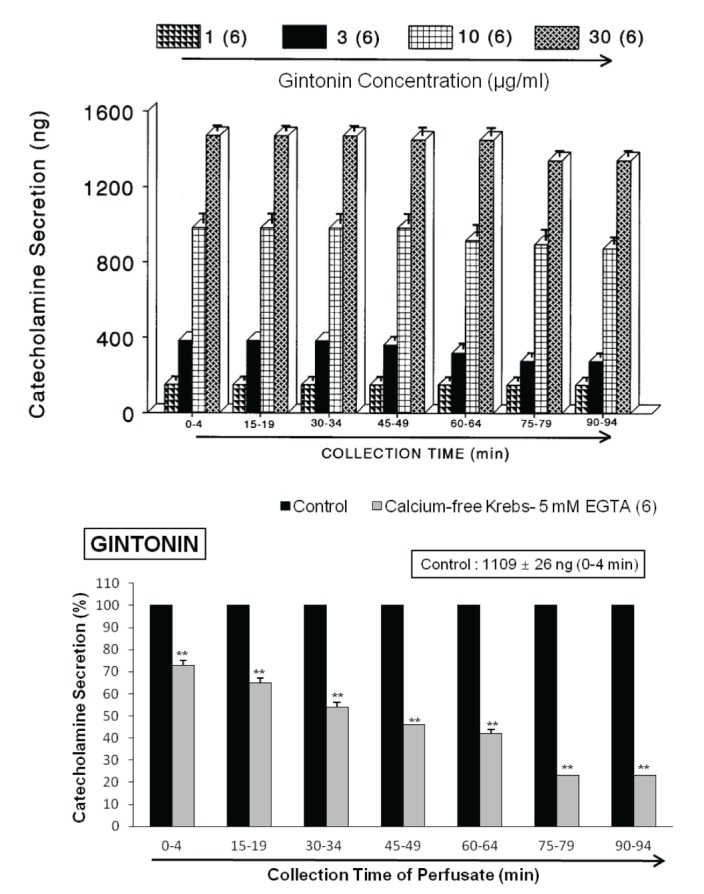
Fig. 2
The effects of nicardipine (upper) and TMB-8 (lower) on gintonin-evoked CA secretory responses in the perfused rat adrenal glands.
Gintonin (10 µg/ml)-evoked CA secretion was induced at 15 min intervals during the perfusion with nicardipine (1 µM) or TMB-8 (10 µM) for 90 min. Statistical differences were compared between amounts of CA evoked by gintonin before and after treatment with nicardipine or TMB-8. Other legends are the same as in Fig. 1. **p<0.01.
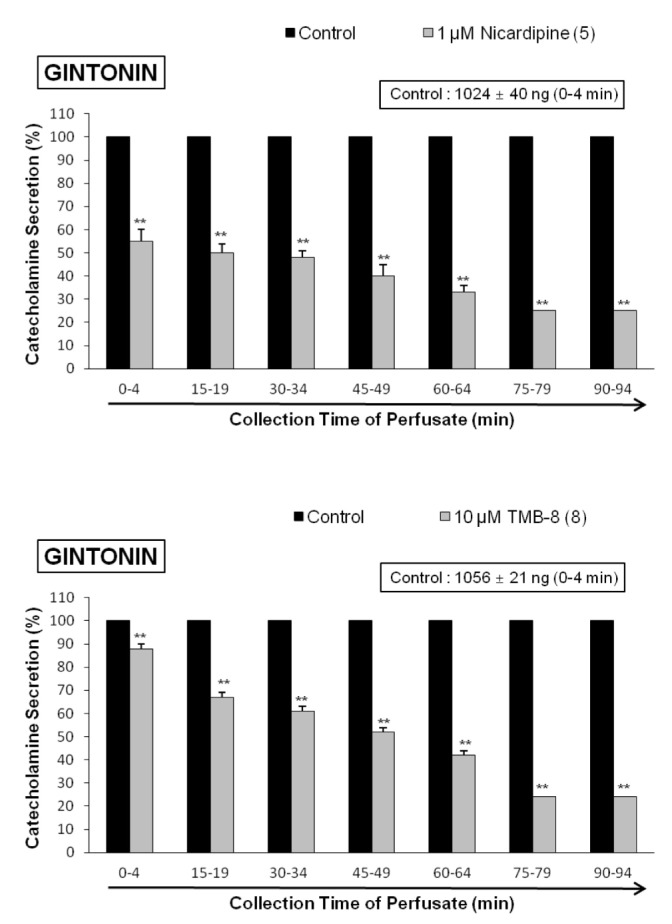
Fig. 3
The effects of amiloride (upper) and nitroprusside (lower) on gintonin-evoked CA secretory responses in the perfused rat adrenal glands.
Gintonin (10 µg/ml)-evoked CA secretion was induced at 15 min intervals during the perfusion with amiloride (1 mM) or nitroprusside (100 µM) for 90 min. Statistical differences were compared between amounts of CA evoked by gintonin before and after treatment with amiloride or nitroprusside. There was no significance between groups before and after treatment with nitroprusside. Other legends are the same as in Fig. 1. **p<0.01.
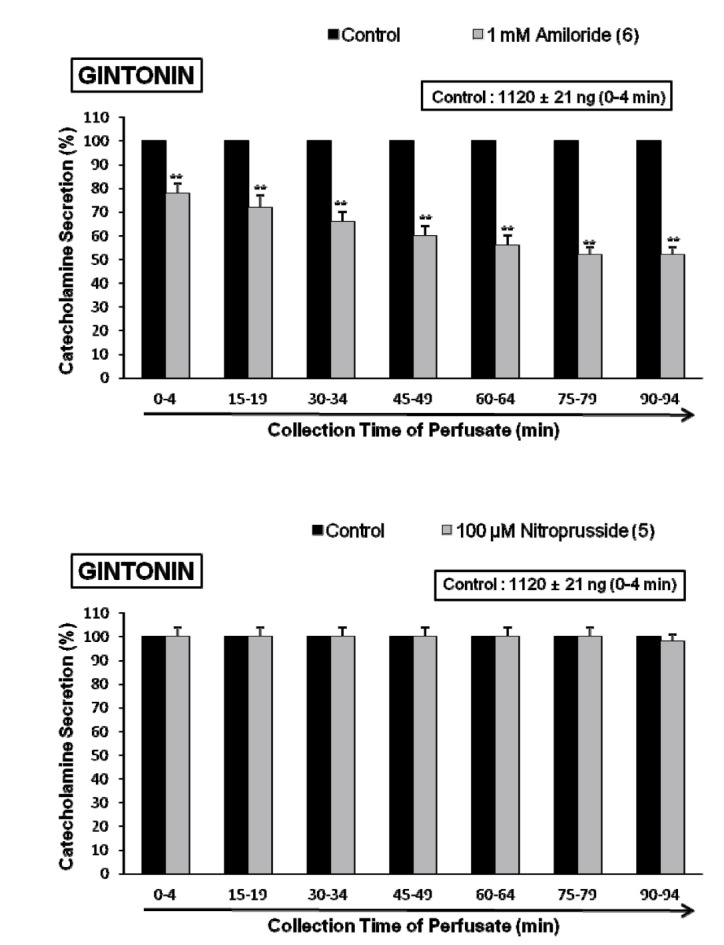
Fig. 4
The effects of chlorisondamine (upper) and pirenzepine (lower) on gintonin-evoked CA secretory responses in the perfused rat adrenal glands.
Gintonin (10 µg/ml)-evoked CA secretion was induced at 15 min intervals during the perfusion with chlorisondamine (1 µM) and pirenzepine (2 µM) for 90 min. Statistical differences were compared between amounts of CA evoked by gintonin before and after treatment with chlorisondamine and pirenzepine. Other legends are the same as in Fig. 1. **p<0.01.
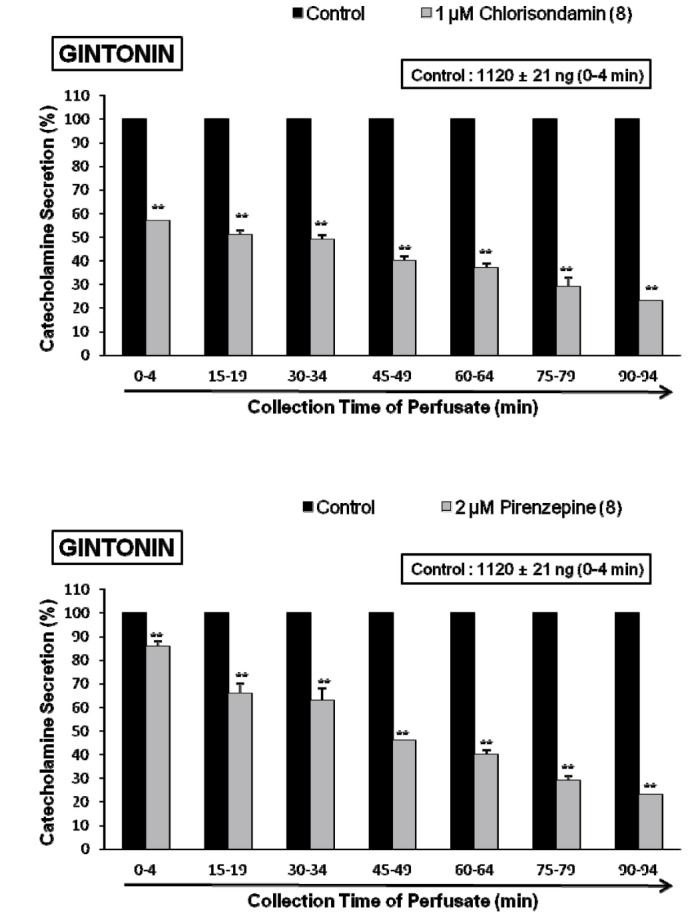
Fig. 5
The effects of Ki16425 on gintonin-evoked CA secretory responses (upper) and concentration-dependent effect of LPA-evoked CA secretion (lower) in the perfused rat adrenal glands.
Gintonin (10 µg/ml)-evoked CA secretion was induced at 15 min intervals during the perfusion with Ki16425 (10 µM) for 90 min. LPA (0.3~3 µM) was infused into an adrenal vein for 4min at 15 min intervals during 90 min. Statistical differences were compared between amounts of CA evoked by gintonin before and after treatment with Ki16425. There was statistical significance between concentrations of LPA (p<0.01). Other legends are the same as in Fig. 1. **p<0.01.
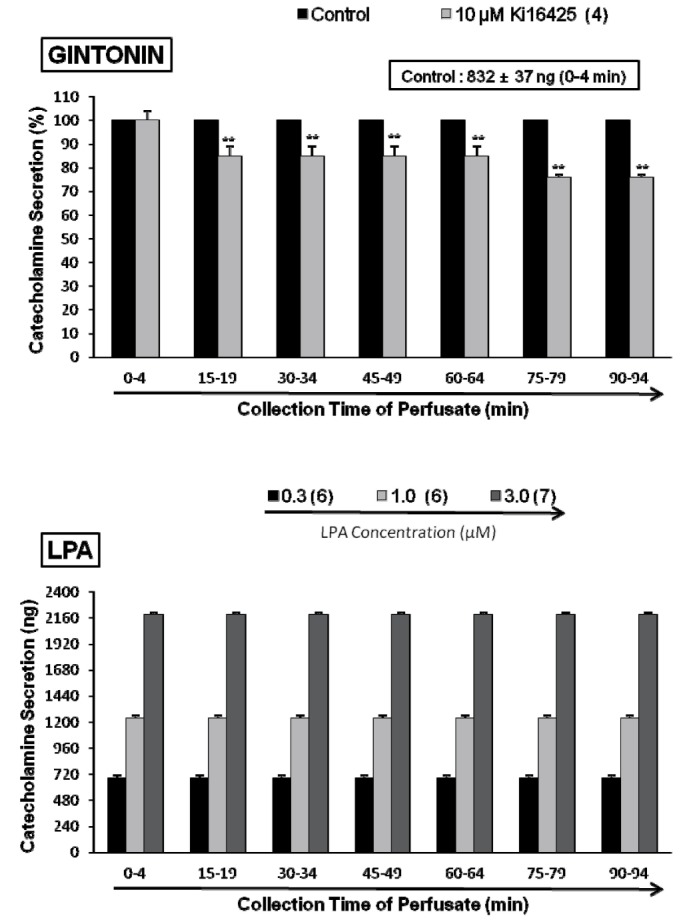
Fig. 6
The effects of Ki16425 on LPA-evoked CA secretory responses (upper) and potentiative effect of gintonin on ACh-evoked CA secretion (lower) in the perfused rat adrenal glands.
LPA (1 µM)-evoked CA secretion was induced at 15 min intervals during the perfusion with Ki16425 (10 µM). ACh (5.32 mM)-evoked CA secretion was induced at 15 min intervals during the perfusion with gintonin (10 µg/ml). Statistical differences were compared between amounts of CA evoked by LPA before and after treatment with Ki16425, and between amounts of CA evoked by ACh before and after treatment with gintonin. Other legends are the same as in Fig. 1. **p<0.01.
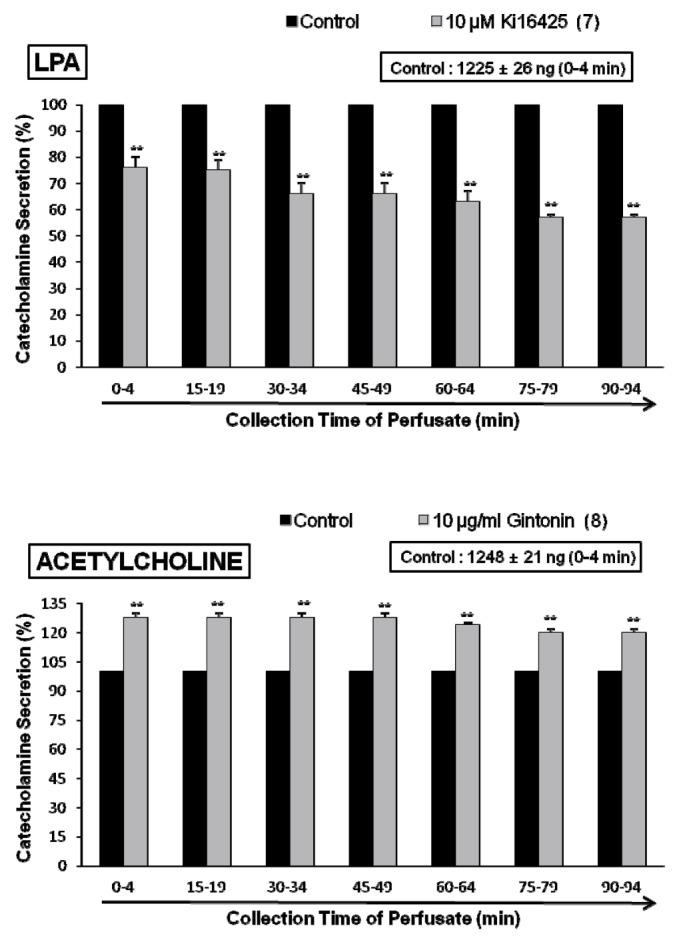