Abstract
Paeoniflorin (PAE) is the most abundant compound in Xuebijing injection widely used to treat sepsis. We aimed to investigate effect of PAE on expression of soluble triggering receptor expressed on myeloid cells-1 (sTREM-1) in a rat model of sepsis. Wistar rats were divided into Normal, Model, and PAE groups (n=20 each). Endotoxin was administrated at 5 mg/ml/kg in Model and PAE rats to establish rat sepsis model. 1 h after endotoxin administration, PAE was administrated at 4 ml/kg in PAE group once per day for 3 days. Routine blood tests and biochemical indexes were assessed, including aspartate aminotransferase (AST) and creatine kinase-MB (CK-MB). The plasma sTREM-1 level was measured using quantitative ELISA. At the end of experiment, the small intestine, liver, kidney and lung were subjected to pathological examinations. A rat model of sepsis-induced multiple organ dysfunction syndrome (MODS) was established successfully with endotoxin administration (5 mg/ml/kg), evidenced by histo-pathological examinations, routine blood tests and biochemical indexes: platelet count decreased and white blood cell count increased (p<0.05), CK-MB and AST increased (p<0.05). PAE treatment significantly reduced the plasma levels of AST, CK-MB, and sTREM-1, compared to Model group (p<0.05). Meanwhile, sepsis-induced damages in the liver, lung, stomach and intestinal mucosa were also markedly ameliorated by PAE treatment. PAE demonstrated a significantly protective effect in a rat model of sepsis by decreasing plasma sTREM-1 level, reducing inflammation, preventing MODS and protecting organ functions.
Severe sepsis, an uncontrolled hyper-inflammatory response of the host to invading pathogens, is a leading cause of mortality in the intensive care unit (ICU) [1]. In US, there are more than 750,000 patients with sepsis per year and the mortality rate is nearly 30% in the ICU [2]. Early diagnosis and treatment are critical to promote the prognosis. However, current clinical indexes usually lack specificity and sensitivity, such as blood leukocyte or neutrophil count, therefore sepsis cannot be readily diagnosed at an early stage in routine clinical practice [3]. It is still a challenge to identify a specific and sensitive marker for the diagnosis of sepsis.
Triggering receptor expressed on myeloid cells-1 (TREM-1) is an immunoglobin expressed on the cell membrane of neutrophils, monocytes, and macrophages [45]. It belongs to a family related to the natural killer cell receptors. TREM-1 upregulates the expression levels of pro-inf lammatory chemokines and cytokines and amplifies the inflammatory responses mediated by toll-like receptors [456]. The soluble form, sTREM-1, is readily measured in biological fluids, and may be used as a diagnostic biomarker for evaluating sepsis severity and prognosis. In clinical studies involving patients with severe sepsis, sTREM-1 has exhibited the potential to provide an excellent predictive value for septic shock/death [7]. For instance, sTREM-1 level was found to be significantly associated with acute kidney injury (AKI) development, which may be used as diagnostic and predictive biomarkers for AKI in critically ill patients with sepsis [8]. Moreover in cancer patients with severe sepsis and septic shock, sTREM-1 appeared to be a good predictor of ICU and 28-day mortality [9]. In a recent pilot study sTREM-1 was also found to be feasible as an accurate diagnostic predictor of late-onset neonatal sepsis [10].
Severe sepsis is refractory to treatment in clinic [1]. In addition to the control of primary diseases and intravenous antibiotic therapy, Chinese herbal medicine has gained more and more attention in the management of severe sepsis. However, the underlying mechanisms are yet to be further investigated. Nowadays, Xuebijing (XBJ) injection is widely used in the treatment of sepsis in China [11121314151617]. There are reports demonstrating that XBJ injection antagonizes endotoxin [141819], inhibits the release of inflammatory mediators [1213141520], promotes phagocytic function of the reticuloendothelial system [21], improves microcirculation [1121], and relieves blood coagulation abnormalities caused by disseminated intravascular coagulation [11].
The main components of XBJ are extracts from Carthamus tinctorius (safflower), Paeonia lactiflora (red peony root), Rhizoma Ligustici (Sichuan lovage rhizome), Salvia miltiorrhiza (red sage root) and Radix Angelicae sinensis (angelica root) [1617], but its main effective component is still unknown hence making the clinical use of XBJ unpredictable and cautious. In a recent study paeoniflorin (PAE) was identified to be the most abundant compound present in XBJ injection [22]. PAE has been reported to exhibit various beneficial effects in studies involving several animal models. For instance in mice, PAE exerted analgesic and hypnotic effects via adenosine A receptors [23], and nephroprotective effect on concanavalin A-induced damage through inhibition of macrophage infiltration [24]. In a transgenic mouse model of Alzheimer's disease, PAE attenuated amyloidogenesis and the inflammatory responses [25]. Its neuroprotective effect was also reported in rat cells, where PAE reduced H2O2-induced toxicity by blocking the activation of the neuro-inflammatory factor NF-κB [26]. Moreover in rats, PAE ameliorated ANIT-induced cholestasis by activating Nrf2 through an PI3K/Akt-dependent pathway [27], and improved regional cerebral blood flow and suppressed inflammatory factors in the hippocampus of rats with vascular dementia [28]. However the effect of PAE as a single pharmaceutical agent on severe sepsis remains unexplored.
The present study investigated the effects of PAE injection in a rat model of sepsis, using plasma sTREM-1 as the biomarker, by performing routine blood tests, biochemical indexes and pathological features, aiming to explore its protective mechanisms against sepsis.
Bacterial endotoxin and paeoniflorin (PAE) were purchased from Sigma, St. Louis, MO, US. A sTREM-1 detection kit, CUSIBIO-CSB-E09681r, was obtained from CusiBio, Wuhan, China.
The rat model of sepsis was established in the study according to the standards as previously described [29]. The standards for a successful animal model of sepsis in laboratory research include: 1) the inducer is similar to that for inducing a multiple organ dysfunction syndrome (MODS); 2) sufficient morbidity and mortality rates; 3) the onset of sepsis is 24 h after injury; 4) exists a systemic inflammatory response syndrome (SIRS); 5) exhibits dysfunction in more than 2 organs and systems.
All animals were treated in compliance with the Guide for the Care and Use of Laboratory Animals (NIH Publication No. 85-23, revised 1996). The protocol was approved by the committee on the Ethics of Animal Experiments of TEDA Hospital. All efforts were made to minimize suffering and numbers of rats used.
Male Wistar rats, weighting 250±35 g, were purchased from the Animal Center at Institute of Radiation Medicine, Chinese Academy of Medical Sciences. 60 rats were randomly divided into 3 groups, with 20 rats each group: Normal group, Model group, PAE group. Rats were acclimated to the surroundings for 1 week before experiments. All rats were housed in on a 12-h light/dark schedule, and allowed ad libitum access to food and water. Endotoxin was administrated through the caudal vein in Model and PAE group rats at 5 mg/ml/kg (stock solution: 3 mg/ml), and a corresponding volume of physiological saline (0.9% NaCl solution) was applied in Normal group rats. 1 h after the administration of endotoxin, PAE injection was administrated through the caudal vein at 4 ml/kg in PAE group rats. PAE was given once a day for 3 consecutive days. As a control, a corresponding volume of physiological saline was given in the other 2 groups.
Blood samples were collected through the inner canthus vein and examined for routine blood tests and blood biochemical indexes (AST, CK-MB) at 4 time points: right before and 1 h after endotoxin administration, 36 and 72 h after PAE administration. At the end of experiment, the small intestine, liver, kidney, and lung were excised out for further histo-pathological examinations by routine H&E staining under a microscope.
In the study, plasma sTREM-1 levels were measured with a quantitative ELISA kit (CusiBio, Wuhan, China), and the concentrations were calculated from the relative O.D. value measured at 450 nm. After centrifugation for 20 min at 1000 g at 4℃ within 30 min of blood sample collection, plasma sTREM-1 levels were measured according to the manufacturer's instruction.
Values were reported as mean±SD (standard deviation) unless otherwise indicated. SPSS19.0 was used for comparing 2 means from different groups, and paired t-test was used for comparing 2 means inside an individual group. Values of p<0.05 were considered statistically significant.
To investigate the effects of PAE injection on sepsis, a rat model of sepsis was developed in the present study. During the development of the rat model of sepsis, routine blood tests were conducted in all the 3 groups of rats at 4 time points: right before and 1 h after endotoxin administration, 36 h and 72 h after PAE administration. Before endotoxin administration, there was no significant difference in the blood platelet count in all the 3 groups. However, the blood platelet count significantly decreased 1 h after endotoxin administration in Model and PAE groups, compared to that in the control of Normal group (Fig. 1A). PAE was administrated 1 h after endotoxin administration in PAE group. At h 36, the blood platelet count significantly recovered in PAE group compared to that in Model group, but still significantly lower than that in Normal group (Fig. 1A). At h 72, the blood platelet count in PAE group reached to a similar level as that in Normal group (Fig. 1A). Of note, the blood platelet count continued to decrease in Model group at h 72 and was significantly higher than that in PAE group. It demonstrated that the administration of PAE promoted the release of platelet into the blood and the blood platelet count recovered to a normal level 72 h after PAE administration in endotoxin-treated rats.
Meanwhile, it was found that the blood white cell count significantly increased in Model and PAE groups 1 h after endotoxin shock, compared to that in the control of Normal group (Fig. 1B). At h 36 after PAE was administrated, the blood white cell count in PAE group significantly decreased, compared to that in Model group (Fig. 1B), but significantly higher than that in Normal group. At h 72, however, the count in PAE group decreased to a similar level as that in Normal group, and was significantly lower than that in Model group.
Taken together the results in the examinations of body temperature, blood platelet count, heart rate, respiration, animal clinical manifestations, and especially pathological results, a rat model of sepsis was developed successfully [29].
At the same time, blood biochemical indexes were examined in all 3 groups of rats during the development of the rat model of sepsis. Clinically, plasma CK-MB is a biomarker of severe heart damage. CK-MB significantly elevated in Model and PAE groups 1 h after rats were administrated with endotoxin, compared to that in Normal group (Fig. 2A). PAE was administrated 1 h after endotoxin injection. At h 36, the CK-MB level in PAE group significantly decreased, compared to that in Model group (Fig. 2A), but still significantly higher than that in Normal group. At h 72, the CK-MB level in PAE group reduced further, and the level was significantly lower than that in Model group (Fig. 2A).
Aspartate aminotransferase (AST) is commonly measured clinically as a part of diagnostic liver function tests. PAE exhibited a similar effect on the AST level: PAE significantly inhibited the AST level induced by endotoxin at h 36, and the inhibitory effect was more pronounced at h 72 (Fig. 2B). The endotoxin-induced AST expression was restored to a similar level as that in Normal group 72 h after PAE administration.
In short, the treatment of PAE injection significantly ameliorated sepsis-induced damages, such as in the heart and liver as evidenced by the restoration of plasma CK-MB and AST levels.
Furthermore, the dynamic change in relative plasma sTREM-1 levels was examined in all 3 groups of rats during the development of the rat model of sepsis. 1 h after administration of endotoxin, the plasma sTREM-1 level significantly increased in Model and PAE groups, compared to that in Normal group (Fig. 3), as previously reported in septic rats [4630]. PAE was administrated 1 h after endotoxin administration. At h 36, the plasma sTREM-1 level in PAE group significantly decreased, compared to that in Model group (Fig. 3), but significantly higher than that in Normal group. At h 72, the sTREM-1 level in PAE group decreased further to a normal level as that in Normal group, and the level was significantly lower than that in Model group (Fig. 3). The results demonstrated that the plasma sTREM-1 level was decreased by PAE treatment, and PAE downregulated the sTREM-1 expression.
Manifestations in the rat model of sepsis were examined and compared among all the 3 groups of rats, including appearance, activity, diet, urination and defecation. Before endotoxin administration, there was no significant difference in the 3 group rats.
1 h after endotoxin administration, various symptoms were observed in Model and PAE group rats, such as crouching with less or no activity, shortness of breath or breath accompanied by shivering, less diet, dry and coarse hair, attenuated or disappeared bowel sound, dark brown urine, and thin stool. In the dying rats, it was also observed, such as slow and shallow breathing, decreased heart rates, weak heart sound, reduced muscle tension, and decreased response to stimulus. Moreover, some rats died 6 h later after endotoxin administration. Noteworthy, the dead rats were not included in the statistical analyses in Fig. 1, 2, and 3. On day 3, Model group rats worsened: low spirits, reduced activity, and decreased response to stimulus.
However, the rats in PAE group exhibited markedly ameliorated symptoms on day 3 even they showed similar manifestations at the early stage after endotoxin was administrated. 72 h after PAE administration, the symptoms in the rats of PAE group were alleviated or disappeared: gradually recovered activity, gradually eased vertical hair, gradually restored heart rate and sound, ameliorated diarrhea, and restored diet.
Symptoms in Model group rats: dark red lung surface, visible bloody fluid exudates from lung sections; enlarged liver, obvious congestion and hemorrhage on the liver surface, dark red liver surface; significantly enlarged kidney, dark red medulla cortex section, pale cortex; flatulence, visible hemorrhage, necrotic foci, bloody exudates in the abdominal cavity; partially intestinal mucosal erosion, hemorrhage. However, rats in PAE group: congested lung; visible blood stasis in the liver; a small amount of bloody exudates in the abdominal cavity; no erosion in the intestinal wall.
In rats from Normal group, the intestinal villi structure was intact and the intervillous space was evenly distributed. In Model group, the damages in the rat organs were significantly severer than those found in PAE group 72 h after PAE administration (Fig. 4): 1) in the lung: pulmonary congestion and edema, pulmonary hemorrhage, alveolar atrophy, thickened alveolar wall, foam cell foci; 2) in the liver: partial hepatic cell focal necrosis, portal infiltration of lymphocytes; 3) in the intestine: severe intestinal mucosal villus necrosis, inflammatory infiltration in the intestinal villus. In PAE group, 72 h after treatment with PAE, there existed (Fig. 4): mild focal necrosis in liver cells, mild alveolar edema and atrophy; mild interstitial pneumonia; mild focal necrosis in liver cells, visible hepatic fibrosis, mild intestinal mucosal villus injury. Of note, pathological changes in the kidney were similar in all the 3 groups (Fig. 4).
Sepsis is a systemic inflammatory response syndrome caused by an infection, and it is a major cause of death in the ICU [1]. The injury caused by sepsis is a result of direct action of pathogenic microorganisms, bowel bacterial translocation, ischemic reperfusion induced injury and immune imbalance [31]. The immune system plays a pivotal role in fighting against an infection. However, overly exuberant immune response may be lethal as happens in sepsis. For the diagnosis of an infection, C-reactive protein, procalcitonin (PCT), and sTREM-1 are widely used. Expression of TREM-1 on the cell membrane was found to be greatly upregualted in septic patients [45]. Besides this membrane-bound form, the soluble counterpart sTREM-1 is also an accurate diagnostic biomarker in various disease involving severe sepsis [7]. It has been demonstrated that the sTREM-1 level has an important value for evaluating prognosis in septic patients with positive blood cultures [432]. Its level can reflect the status and severity of an infection accurately [533]. Comparing patients with a systemic inflammatory response syndrome (SIRS) and those with sepsis, the expression level of sTREM-1 is significantly higher in patients with sepsis, suggesting that it can be used as an index of sepsis [34].
Despite advances in modern medicine, treatment of sepsis remains a challenge. Chinese herbal medicine, especially XBJ injection, has gained more and more attention in clinical trials for the treatment of sepsis in the past decade. Sepsis leads to production of free oxygen radicals, ischemic reperfusion induced injury, while XBJ is an efficient antioxidant [35] which was shown to reduce injury caused by ischemic reperfusion, protect organ function and limit organ injury [3637]. Endotoxin causes different types of tissue and organ damages, and XBJ injection exhibited antagonistic effects against endotoxin toxicity in different animal models [1819]. Of particular interest to our current study, XBJ inhibited the expression of TREM-1 by antagonizing endotoxin [38], through which it decreased the level of Toll-like receptor 4, hence reducing the production of inflammatory cytokines to protect organ functions [39].
PAE was found to inhibit systemic inflammation and improve survival by suppressing the release of pro-inflammatory factors, such as TNF-α and IL-1β, in both in vitro cell culture and in vivo animal models [4041]. PAE was also able to inhibit the activation of the neuro-inflammatory factor NF-κB thereby alleviating H2O2-induced oxidative stress [26]. In the present study, we found that PAE, the most abundant component in XBJ injection [22], significantly inhibited the plasma sTREM-1 level in rats of endotoxin-induced sepsis. Blood indexes, animal manifestations and histo-pathology demonstrated that sepsis-induced damages were markedly ameliorated by the treatment of PAE. Our results supports the use of PAE as a novel therapeutic reagent in treating severe sepsis, but the exact molecular mechanisms as well as potential side effects remain elusive. However the advantage of PAE over XBJ lies in that, compared with XBJ which is a mixture of herbal extracts, PAE is a single pharmaceutical agent, whose pharmacokinetics could be easily measured. In this context, our study provided evidences on significant beneficial roles of PAE in alleviating endotoxin-induced sepsis, which certainly warrants additional studies to elucidate the molecular target accounting for these organ protecting effects, as well as pharmacokinetics studies to direct its prescribed use in human patients.
In conclusion, our current study demonstrated a significantly protective effect of PAE injection in the rat model of sepsis, where it decreased the plasma sTREM-1 level, reduced inflammation, prevented MODS and protected organ functions. Our current study has identified PAE as the main functional component of XBJ against endotoxin-induced sepsis, thereby providing a novel and more precise therapeutic approach in the clinical treatment of severe septic symptoms.
ACKNOWLEDGEMENTS
This study was funded by Integrative Medicine Topic of Tianjin Administration of Traditional Chinese Medicine (11111, 13145).
Notes
References
1. Angus DC, van der Poll T. Severe sepsis and septic shock. N Engl J Med. 2013; 369:840–851. PMID: 23984731.


2. Angus DC, Linde-Zwirble WT, Lidicker J, Clermont G, Carcillo J, Pinsky MR. Epidemiology of severe sepsis in the United States: analysis of incidence, outcome, and associated costs of care. Crit Care Med. 2001; 29:1303–1310. PMID: 11445675.


3. Prucha M, Bellingan G, Zazula R. Sepsis biomarkers. Clin Chim Acta. 2015; 440:97–103. PMID: 25447700.


4. Gibot S, Cravoisy A, Levy B, Bene MC, Faure G, Bollaert PE. Soluble triggering receptor expressed on myeloid cells and the diagnosis of pneumonia. N Engl J Med. 2004; 350:451–458. PMID: 14749453.


5. Gibot S. Clinical review: role of triggering receptor expressed on myeloid cells-1 during sepsis. Crit Care. 2005; 9:485–489. PMID: 16277737.
6. Knapp S, Gibot S, de Vos A, Versteeg HH, Colonna M, van der Poll T. Cutting edge: expression patterns of surface and soluble triggering receptor expressed on myeloid cells-1 in human endotoxemia. J Immunol. 2004; 173:7131–7134. PMID: 15585833.


7. Lemarié J, Barraud D, Gibot S. Host response biomarkers in sepsis: overview on sTREM-1 detection. Methods Mol Biol. 2015; 1237:225–239. PMID: 25319790.


8. Dai X, Zeng Z, Fu C, Zhang S, Cai Y, Chen Z. Diagnostic value of neutrophil gelatinase-associated lipocalin, cystatin C, and soluble triggering receptor expressed on myeloid cells-1 in critically ill patients with sepsis-associated acute kidney injury. Crit Care. 2015; 19:223. PMID: 25944130.


9. Ravetti CG, Moura AD, Vieira ÉL, Pedroso ÊR, Teixeira AL. sTREM-1 predicts intensive care unit and 28-day mortality in cancer patients with severe sepsis and septic shock. J Crit Care. 2015; 30:440.e7–440.e13.


10. Arízaga-Ballesteros V, Alcorta-García MR, Lázaro-Martínez LC, Amézquita-Gómez JM, Alanís-Cajero JM, Villela L, Castorena-Torres F, Lara-Díaz VJ. Can sTREM-1 predict septic shock & death in late-onset neonatal sepsis? A pilot study. Int J Infect Dis. 2015; 30:27–32. PMID: 25461656.
11. Hou SY, Feng XH, Lin CL, Tan YF. Efficacy of Xuebijing for coagulopathy in patients with sepsis. Saudi Med J. 2015; 36:164–169. PMID: 25719579.


12. Jiang M, Zhou M, Han Y, Xing L, Zhao H, Dong L, Bai G, Luo G. Identification of NF-κB Inhibitors in Xuebijing injection for sepsis treatment based on bioactivity-integrated UPLC-Q/TOF. J Ethnopharmacol. 2013; 147:426–433. PMID: 23524166.


13. He XD, Wang Y, Wu Q, Wang HX, Chen ZD, Zheng RS, Wang ZS, Wang JB, Yang Y. Xuebijing protects rats from sepsis challenged with acinetobacter baumannii by promoting annexin A1 expression and inhibiting proinflammatory cytokines secretion. Evid Based Complement Alternat Med. 2013; 2013:804940. PMID: 24369483.
14. Gao YL, Chai YF, Yao YM. Advancement in the research of mechanism of immune dysfunction in sepsis and the regulatory effects of Xuebijing injection. Zhonghua Shao Shang Za Zhi. 2013; 29:162–165. PMID: 23985206.
15. Shao M, Liu B, Wang JQ, Tao XG, Zhou SS, Jin K, Zhang CP. Effect of Xuebijing injection on T helper 17 and CD4+ CD25+ regulatory T cells in patients with sepsis. Zhongguo Wei Zhong Bing Ji Jiu Yi Xue. 2011; 23:430–434. PMID: 21787474.
16. Zhu XQ, Wang L, Liu QQ, Yao YM. Protective effects of Xuebijing injection on kidney in rats with sepsis. Zhongguo Wei Zhong Bing Ji Jiu Yi Xue. 2006; 18:680–683. PMID: 17092422.
17. Zhang SW, Sun CD, Wen Y, Yin CH. Effect of treatment with Xuebijing injection on serum inflammatory mediators and Th1/2 of spleen in rats with sepsis. Zhongguo Wei Zhong Bing Ji Jiu Yi Xue. 2006; 18:673–676. PMID: 17092419.
18. Guo SS, Gao YJ, Tian XC, Jin YH, Liu FZ, Cui XL. Effect of xuebijing oral effervescent tablet on endotoxin induced fever and disseminated intravascular coagulation rabbit model. Yao Xue Xue Bao. 2013; 48:1241–1246. PMID: 24187830.
19. Yao XQ, Zhang YH, Sun CH. Protective effects of Xuebijing effervescent tablet on vital organs in rats with toxic injury induced by endotoxin. Zhongguo Wei Zhong Bing Ji Jiu Yi Xue. 2012; 24:357–359. PMID: 23019734.
20. Liu YK, He JB, Chen HE, Chen D, Miao YF, Ying L, You X, Wang WT. Effect of Xuebijing Injection on TLR4-NF-κB-TNF-α pathway of rats' myocardial anoxia/reoxygenation. Zhongguo Zhong Xi Yi Jie He Za Zhi. 2014; 34:1463–1468. PMID: 25632747.
21. He J, Tan Z, Zhang M, Guo L. Effect of Xuebijing injection on hemodynamics and endothelial function in patients with severe sepsis: a prospective study. Zhonghua Wei Zhong Bing Ji Jiu Yi Xue. 2015; 27:127–132. PMID: 25665612.
22. Ji L, Huang H, Jiang M, Bai G, Luo G. Simultaneous HPLC determination of 11 essential compounds in Xuebijing injection. Zhongguo Zhong Yao Za Zhi. 2010; 35:2395–2398. PMID: 21141485.
23. Yin D, Liu YY, Wang TX, Hu ZZ, Qu WM, Chen JF, Cheng NN, Huang ZL. Paeoniflorin exerts analgesic and hypnotic effects via adenosine A1 receptors in a mouse neuropathic pain model. Psychopharmacology (Berl). 2016; 233:281–293. [Epub ahead of print]. PMID: 26514553.


24. Liu C, Cheng Z, Wang Y, Dai X, Zhang J, Xue D. Paeoniflorin exerts a nephroprotective effect on concanavalin A-induced damage through inhibition of macrophage infiltration. Diagn Pathol. 2015; 10:120. PMID: 26204936.


25. Zhang HR, Peng JH, Cheng XB, Shi BZ, Zhang MY, Xu RX. Paeoniflorin atttenuates amyloidogenesis and the inflammatory responses in a transgenic mouse model of alzheimer's disease. Neurochem Res. 2015; 40:1583–1592. PMID: 26068144.


26. Li P, Li Z. Neuroprotective effect of paeoniflorin on H2O2-induced apoptosis in PC12 cells by modulation of reactive oxygen species and the inflammatory response. Exp Ther Med. 2015; 9:1768–1772. PMID: 26136891.


27. Chen Z, Ma X, Zhu Y, Zhao Y, Wang J, Li R, Chen C, Wei S, Jiao W, Zhang Y, Li J, Wang L, Wang R, Liu H, Shen H, Xiao X. Paeoniflorin ameliorates ANIT-induced cholestasis by activating Nrf2 through an PI3K/Akt-dependent pathway in rats. Phytother Res. 2015; 29:1768–1775. PMID: 26269092.


28. Zhang LG, Wang LJ, Shen QQ, Wang HF, Zhang Y, Shi CG, Zhang SC, Zhang MY. Paeoniflorin improves regional cerebral blood flow and suppresses inflammatory factors in the hippocampus of rats with vascular dementia. Chin J Integr Med. 2015; [Epub ahead of print].


29. HU S, Sheng ZY, Zhou BT. Studies on the animal models of two-phase late onset of multiple organ dystrophy syndrome. Chin J Traumatol. 1996; 12:102–106.
30. Determann RM, Millo JL, Gibot S, Korevaar JC, Vroom MB, van der Poll T, Garrard CS, Schultz MJ. Serial changes in soluble triggering receptor expressed on myeloid cells in the lung during development of ventilator-associated pneumonia. Intensive Care Med. 2005; 31:1495–1500. PMID: 16195904.


31. Yao YM, Luan YY, Zhang QH, Sheng ZY. Pathophysiological aspects of sepsis: an overview. Methods Mol Biol. 2015; 1237:5–15. PMID: 25319775.


32. Barati M, Bashar FR, Shahrami R, Zadeh MH, Taher MT, Nojomi M. Soluble triggering receptor expressed on myeloid cells 1 and the diagnosis of sepsis. J Crit Care. 2010; 25:362.e1–362.e6.


33. Su L, Han B, Liu C, Liang L, Jiang Z, Deng J, Yan P, Jia Y, Feng D, Xie L. Value of soluble TREM-1, procalcitonin, and C-reactive protein serum levels as biomarkers for detecting bacteremia among sepsis patients with new fever in intensive care units: a prospective cohort study. BMC Infect Dis. 2012; 12:157. PMID: 22809118.


34. Wang HX, Chen B. Diagnostic role of soluble triggering receptor expressed on myeloid cell-1 in patients with sepsis. World J Emerg Med. 2011; 2:190–194. PMID: 25215008.


35. Cui Y, Zhang YX, Li CX, Li Y, Shen HL. The effects of nitrogen monoxide/inducible nitric oxide synthase on renal injury in septic rats and the renal protective effects of Xuebijing injection. Zhongguo Wei Zhong Bing Ji Jiu Yi Xue. 2009; 21:497–498. PMID: 19695177.
36. Shen J, Lin XJ, Cui BK, Chi PD, Zeng QY, Zhao QY. The protective effect of Xuebijing injection pretreatment on hepatic ischemia reperfusion injury and coagulopathy after excision of liver cancer. Zhonghua Wei Zhong Bing Ji Jiu Yi Xue. 2013; 25:743–748. PMID: 24620386.
37. Ma JF, Xuan LZ, Wu W, Zhu DM. Effect of Xuebijing injection on rabbits ischemia/reperfusion injury induced by femoral arterial disease. Zhongguo Wei Zhong Bing Ji Jiu Yi Xue. 2012; 24:233–236. PMID: 22464578.
38. Lu Y, Sheng HL, Wang LH. Effects of Xuebijing injection on the expression of sTREM-1 in severe spetic patients. Chin J TCM WM Crit Care. 2013; 20:337–340.
39. Liu MW, Wang YH, Qian CY, Li H. Xuebijing exerts protective effects on lung permeability leakage and lung injury by upregulating Toll-interacting protein expression in rats with sepsis. Int J Mol Med. 2014; 34:1492–1504. PMID: 25269519.


40. Jiang WL, Chen XG, Zhu HB, Gao YB, Tian JW, Fu FH. Paeoniflorin inhibits systemic inflammation and improves survival in experimental sepsis. Basic Clin Pharmacol Toxicol. 2009; 105:64–71. PMID: 19371254.


41. Cao W, Zhang W, Liu J, Wang Y, Peng X, Lu D, Qi R, Wang Y, Wang H. Paeoniflorin improves survival in LPS-challenged mice through the suppression of TNF-α and IL-1β release and augmentation of IL-10 production. Int Immunopharmacol. 2011; 11:172–178. PMID: 21094290.


Fig. 1
Platelet (A) and white blood cell (B) count of rats in PAE, Model and Normal experimental groups (see Materials and Methods section) at indicated time points.
Data are shown as mean±SEM (n=20). *p<0.05 PAE and Model vs Normal; **p<0.05 PAE vs either Model or Normal; #p<0.01 PAE vs Model.
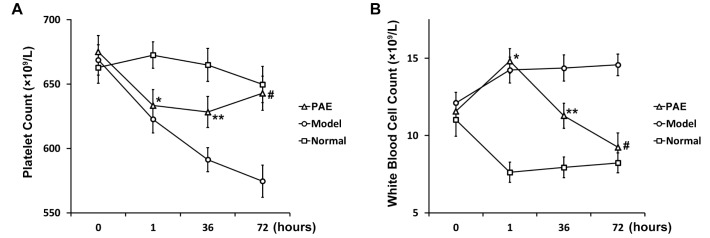
Fig. 2
CK-MB (A) and AST (B) test of rats in PAE, Model and Normal experimental groups (see Materials and Methods section) at indicated time points.
Data are shown as mean±SEM (n=20). *p<0.05 PAE and Model vs Normal; **p<0.05 PAE vs either Model or Normal; #p<0.05 PAE vs Model.
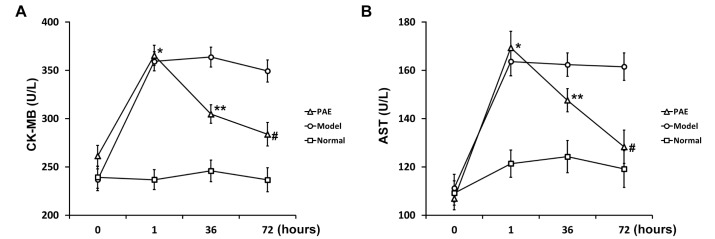