Abstract
Human cardiac fibroblasts (HCFs) have various voltage-dependent K+ channels (VDKCs) that can induce apoptosis. Hydrogen peroxide (H2O2) modulates VDKCs and induces oxidative stress, which is the main contributor to cardiac injury and cardiac remodeling. We investigated whether H2O2 could modulate VDKCs in HCFs and induce cell injury through this process. In whole-cell mode patch-clamp recordings, application of H2O2 stimulated Ca2+-activated K+ (KCa) currents but not delayed rectifier K+ or transient outward K+ currents, all of which are VDKCs. H2O2-stimulated KCa currents were blocked by iberiotoxin (IbTX, a large conductance KCa blocker). The H2O2-stimulating effect on large-conductance KCa (BKCa) currents was also blocked by KT5823 (a protein kinase G inhibitor) and 1 H-[1, 2, 4] oxadiazolo-[4, 3-a] quinoxalin-1-one (ODQ, a soluble guanylate cyclase inhibitor). In addition, 8-bromo-cyclic guanosine 3', 5'-monophosphate (8-Br-cGMP) stimulated BKCa currents. In contrast, KT5720 and H-89 (protein kinase A inhibitors) did not block the H2O2-stimulating effect on BKCa currents. Using RT-PCR and western blot analysis, three subtypes of KCa channels were detected in HCFs: BKCa channels, small-conductance KCa (SKCa) channels, and intermediate-conductance KCa (IKCa) channels. In the annexin V/propidium iodide assay, apoptotic changes in HCFs increased in response to H2O2, but IbTX decreased H2O2-induced apoptosis. These data suggest that among the VDKCs of HCFs, H2O2 only enhances BKCa currents through the protein kinase G pathway but not the protein kinase A pathway, and is involved in cell injury through BKCa channels.
Cardiac fibroblasts (CFs) are the largest population of cells in the heart, and they play a critical role in maintaining its normal function and homeostasis [1]. These cells contribute to the structural, biochemical, mechanical, and electrical characteristics of the heart and are the main source of collagen, which is the most important component of the cardiac extracellular matrix [2]. Excessive extracellular matrix deposition and CFs proliferation lead to heart failure, sudden cardiac death, and other serious complications [3]; therefore, these cells act as mediators of inflammatory and fibrotic myocardial remodeling in injured hearts [45].
It has been reported that extensive networks exist between CFs and cardiomyocytes through numerous anatomical contacts, which suggests potential heterocellular electrical coupling in diseased myocardium in arrhythmogenesis [267].
The ion channels present in cardiomyocytes and their functions have been well studied. CFs also have multiple ion channels, but their distribution and properties are quite distinct from those in cardiomyocytes [8]. It is well known that K+ channel-mediated signals, especially from voltage-dependent K+ channels (VDKCs), play an important role in cell death or apoptosis in many cell types [910111213]. There are two types of VDKCs, Ca2+-activated K+ (KCa) channels and voltage-gated K+ (KV) channels. These channels are found in nearly every cell type, which suggests their physiological importance.
KCa channels can regulate membrane potential and intracellular K+ concentration, and constitute a major link between second messengers and the electrical activity of cells. There are three families of KCa channels that are based on differences in their biophysical and pharmacological properties, large-conductance KCa (BKCa or KCa1.1), intermediate-conductance KCa (IKCa or KCa3.1), and small-conductance KCa (SKCa or KCa2.x) channels. The ubiquitous BKCa channels are composed of pore-forming α subunits and four auxiliary β subunits, and gating is regulated by Ca2+ and membrane voltage [14]. BKCa currents have large conductances of 100~250 and 200~300 pS in physiological K+ gradients and a symmetrical 140 mM K+ solution, respectively [15]. In contrast, IKCa channels are voltage-independent and have intermediate single channel conductance values of 20~80 pS in physiological conditions [16]. Finally, SKCa channels have a small unitary conductance of 4~14 pS [17].
KV channels modulate electrical excitability, regulate the repolarization of action potential, and are divided into two subfamilies, delayed rectifier K+ (KDR) or transient outward K+ (KTO). KDR channels show fast activating kinetics with slow inactivation or no inactivation, whereas KTO channels show fast activation and inactivation kinetics [1819].
Hydrogen peroxide (H2O2), an oxidative stress inducer, is the main contributor to cardiac injury and remodeling [20]. H2O2 has been shown to activate or inhibit ion channels depending on channel type; it inhibits voltage-gated KTO currents and KDR currents in rat hippocampal neurons [21], but enhances BKCa currents in human dermal fibroblasts [9] and human endothelial cells through the cGMP signaling pathway [22]. In cardiomyocytes, H2O2 decreased KDR currents in the ventricular myocytes of adult guinea pigs and increased apoptosis [23]. In contrast, H2O2 decreased KTO currents but increased KDR currents in rabbit atrial myocytes [24]. However, in human CFs (HCFs), whether H2O2 can modulate K+ channels and its mechanism still remain unclear.
In this study, we identified three types of VDKCs in HCFs and investigated the effects of H2O2 on these K+ channels using a whole-cell patch-clamp technique. The key protein kinase pathways involved in the observed effects of H2O2 on the K+ currents and H2O2-induced injury of HCFs as well as the relationship between H2O2-induced injury and K+ currents were also investigated.
Human cardiac fibroblasts (adult ventricle, Catalog #6310) were purchased from ScienCell Research Laboratory (San Diego, CA). Cells were cultured in Dulbecco's modified Eagle's medium (DMEM; Welgene, Korea) supplemented with 10% fetal bovine serum (FBS; Welgene) and penicillin-streptomycin solution (GenDEPOT, Barker, TX) in a humidified atmosphere of 5% CO2 and 95% air at 37℃. Confluent fibroblasts were detached by incubation with 0.25% trypsin (Welgene) and DMEM for a few minutes. The detached cells were pelleted by centrifugation, the supernatant was removed, and the pellet was suspended in 1 mL of bath solution. Cells used in this study were from early passages (3 to 7) to limit possible variation.
A small aliquot of solution containing HCFs was placed in an open perfusion chamber mounted on the stage of an inverted microscope. Whole-cell currents were recorded with an Axopatch 200B patch clamp amplifier (Axon Instruments, Union City, CA) at room temperature. pCLAMP 9.0 software (Axon Instruments) was used for data acquisition and analysis of whole-cell currents. Activated currents were filtered at 2 kHz and digitized at 10 kHz. Patch pipettes were prepared from filament-containing borosilicate tubes (TW150F-4; World Precision Instruments, Sarasota, FL) using a two-stage microelectrode puller (PC-10; Narishige, Tokyo, Japan), and then fire polished on a microforge (MF-830; Narishige). When filled with pipette solution, the pipettes exhibited a resistance of 2~3 MΩ. The bath solution used for whole-cell recordings contained (in mM): 145 NaCl, 5 KCl, 1 CaCl2, 1 MgCl2, 5 glucose, and 5 HEPES (pH adjusted to 7.35 with NaOH). The pipette solution for patch clamping contained (in mM): 145 KCl, 1.652 CaCl2 (pCa 6.0), 1.013 MgCl2, 10 HEPES, 2 EGTA, and 2 K-ATP (pH adjusted to 7.3 with KOH). H2O2, iberiotoxin (IbTX, a BKCa channel blocker), KT5823 (a protein kinase G inhibitor), 1H-[1,2,4]-oxadiazolo [4,3-a]quinoxalin-1-one (ODQ, a soluble guanylyl cyclase inhibitor), 8-bromo-cyclic guanosine 3', 5'-monophosphate (8-Br-cGMP), KT5720 and H-89 (protein kinase A inhibitors), and all other chemicals were purchased from Sigma-Aldrich (St. Louis, MO).
Total RNA was extracted from HCFs using the Total RNA Isolation PureLink RNA Mini Kit (Ambion, Carlsbad, CA). First-strand cDNA was prepared with the SuperScript III Cells Direct cDNA Synthesis Kit (Invitrogen, Tokyo, Japan). Reverse transcription was performed in a S1000 Thermal Cycler (Bio-Rad, Hercules, CA), according to the manufacturer's instructions. RT-PCR reaction products (cDNA) were resolved by 1.2% agarose gel electrophoresis, and stained with ethidium bromide for visualization under ultraviolet light. The primer sequences are listed in Table 1.
The cells were rinsed twice with ice-cold phosphate buffered saline (PBS) and then lysed in ice-cold lysis buffer. After incubation on ice for 30 min with shaking, the samples were centrifuged at 14,000 rpm for 10 min at 4℃. The protein concentration was measured by the Bradford assay. Extracts containing 20 µg of protein were mixed with 5× sample buffer, boiled for 10 min, and then subjected to continuous electrophoresis using 10~12% SDS-PAGE gels. The separated proteins were transferred to polyvinylidene difluoride (PVDF) membranes (IPVH00010; EMD Millipore, Billerica, MA) by electrophoretic transfer and blocked with 5% skim milk in trisbuffered saline containing 0.1% Tween-20 (TBS-T) for 1 h at room temperature. The membranes were rinsed and incubated with primary antibodies at 4℃ overnight. Removal of primary antibodies was carried out by washing for 4×10 min in TBS-T. The secondary antibodies were incubated with membrane in TBS-T with 5% skim milk for 1 h at room temperature. After a final wash with TBS-T for 4×15 min, the bands were detected using the chemiluminescence system (ECL, Thermo Fisher Scientific, Rockford, IL). The results were analyzed with Bio-Rad molecular analysis software. The primary and secondary antibodies used are listen in Table 2.
Apoptosis of HCFs was determined using flow cytometric analysis. Cellular apoptosis was observed by annexin V-FITC/PI double staining using the Annexin V/Dead Cell Apoptosis Kit (Invitrogen) according to the manufacturer's instructions. Briefly, cells were seeded at a density of 6×105 cells/mL and cultured in DMEM containing 100 µM H2O2 for 6 h, or with IbTX for 24 h, and then 100 µM H2O2 was added and incubated for 6 h. Cells were harvested by treatment with trypsin, and then washed twice with cold PBS and centrifuged to collect the cell pellet. Annexin V-FITC and PI were added, and the cells were incubated in the dark at room temperature. Cells were analyzed with a flow cytometer (BD Biosciences) at 530 nm. Data from 10,000 cells were collected for each data file. Annexin V-FITC-positive and PI-negative cells were defined as apoptotic cells. Finally, the number of cells in each category was expressed as a percentage of the total number of stained cells.
Data are expressed as the mean±standard error of the mean (S.E.M.). Comparison of measurements between groups was performed using Student's t-test or one way ANOVA, depending on the experimental design. Differences were considered significant at p values less than either 0.05 or 0.01.
Macroscopic K+ currents were generated using a voltage protocol that consisted of depolarizing steps (from −80 mV to +50 mV) in 10 mV increments for 400 ms with a −80 mV holding potential in whole-cell mode patch-clamp recordings. Ca2+-activated K+ (KCa) channels and voltage-gated K+ (KV) currents with typical behavior were recorded in HCFs. KCa currents showed strong oscillation in response to strong depolarization, were well maintained throughout the test pulse without marked inactivation during depolarizing voltage increments, and their current-voltage (I~V) relationship showed strong outward rectification (Fig. 1A). These currents were detected in 47% (197 out of 419) of cells. Two types of KV currents could be distinguished based on their activation and inactivation kinetics: fast activating and slow or non-inactivating currents, called delayed rectifier K+ (KDR) currents (Fig. 1B), and fast activating and inactivating currents, called transient outward K+ (KTO) currents (Fig. 1C). KDR and KTO currents were detected in 46.8% and 6.2% of cells, respectively. They did not show the outward rectification in their I~V curves.
To determine the effect of H2O2 on outward K+ currents in HCFs, H2O2 (100 µM) was added to the bath solution. In the presence of H2O2, KCa currents were significantly increased (Fig. 1A). This increase was 274.6±29.7% of that in the absence of H2O2 at +50 mV (n=13, *p<0.05 vs. control). The current densities of KCa currents at +50 mV in the absence and presence of H2O2 were 3.43±0.77 pA/pF and 9.42±2.27 pA/pF, respectively (n=13, p<0.05, Fig. 1D). In contrast, H2O2 had no significant effects on KDR currents (106.7±10.3% of the control, n=12, Fig. 1B) or KTO currents (101.2±10.1% of the control, n=8; Fig. 1C). At +50 mV, the current densities did not differ (control KDR, 3.77±0.96 pA/pF vs. with H2O2, 4.03±0.99 pA/pF; control KTO, 3.30±0.91 pA/pF vs. with H2O2, 3.34±0.92 pA/pF, Fig. 1D)
To determine which of the KCa channels subtypes are present in HCFs, KCa channel gene expression was examined by RT-PCR using specific primers for the KCa channel families (shown in Table 1). We detected clear amplification of the mRNA encoding the pore-forming α subunit of the KCa1.1(BKCa) channel along with the auxiliary 1.1β1, 1.1β3, and 1.1β4 subunits (Fig. 2A). The mRNA expression of the KCa2.2 and KCa2.3 subunits of the SKCa channel and the KCa3.1 subunit of the IKCa channel was also detected by RT-PCR. Expression of the KCa1.1β2 subunit of BKCa and the KCa2.1 subunit of SKCa was not detected. To examine whether the ion channels that were detected by RT-PCR were expressed in protein form, a western blot analysis was performed. As shown in Fig. 2B, the expected sizes of immunoreactive protein bands for the BKCa channel subunits (KCa1.1α and KCa1.1β) were detected (molecular weights, 125 kDa and 22 kDa, respectively). Expression of the subunits of the SKCa channels KCa2.2 and KCa2.3 and the IKCa channel KCa3.1 was also detected as prominent bands of molecular weights 64 kDa, 82 kDa, and 47 kDa, respectively. β-Actin (42 kDa) was used as a control for normalization.
Previous studies have suggested that BKCa channels are the predominant KCa channels in HCFs [8925]. Here, we employed IbTX, a specific BKCa channel blocker, to examine whether the K+ currents modulated by H2O2 were BKCa currents. IbTX (100 nM) significantly inhibited the strong oscillation of KCa currents (44.2±7.4% of the control at 50 mV, n=6, *p<0.05 vs. control; Fig. 3A). The current densities of KCa currents at +50 mV in the absence and presence of IbTX were 3.38±0.94 pA/pF and 1.49±0.70 pA/pF, respectively (n=6, p<0.05, Fig. 3C).
The H2O2-stimulated KCa currents were also decreased by IbTX (from 208.1±10.3% to 116.2±5.7%, n=5, *p<0.05 vs. control; Fig. 3B). The current densities of KCa currents at +50 mV in the control, H2O2, and IbTX were 3.11±0.98 pA/pF, 6.48±1.0 pA/pF, and 3.62±0.55 pA/pF, respectively (n=5, *p<0.05 vs. control, #p<0.05 vs. H2O2 ; Fig. 3C).
We next examined whether the H2O2-induced increase in BKCa currents was associated with H2O2-induced cell injury and found that IbTX attenuated the H2O2-induced apoptosis of HCFs. The apoptosis patterns of HCFs induced by H2O2 were analyzed by flow cytometry. The resulting cytogram shows the percentage of cells with an apoptosis-specific staining pattern (Fig. 4). In total, 28.02% of the cells treated with H2O2 showed late apoptotic changes, whereas 7.18% of control cells were late apoptotic. When we pretreated the cells with IbTX for 24 h before adding H2O2, only 12.38% of the cells showed the late apoptotic changes induced by H2O2. These data demonstrated that the inhibition of K+ efflux through BKCa channels was able to inhibit late apoptosis and that H2O2-induced apoptotic cell death of HCFs is mediated by BKCa channels.
We studied whether the effects of H2O2 on BKCa currents was mediated by the cGMP signaling pathway. We added KT5823 (a PKG inhibitor, 1 µM) to the bath solution for 20 min, and then treated the cells with 100 µM H2O2. Under these conditions, H2O2 failed to increase BKCa currents (95.1±8.3%, n=7; Fig. 5A). When we pretreated the cells with ODQ (a soluble guanylate cyclase inhibitor, 10 µM) for 20 min, H2O2 also failed to increase BKCa currents (89.4±9.9%, n=5; Fig. 5B). We also assessed the effects of cGMP, which is generated by NO binding to soluble guanylate cyclase. Treatment with 8-Br-cGMP (a membranepermeable cGMP analogue, 300 µM) increased the BKCa currents to 169.2±14.6% of the control at +50 mV (n=5, p<0.05 vs. control; Fig. 5C). Fig. 5D shows the effect of H2O2 on the current density at +50 mV after KT5823 pretreatment (control, 3.20±0.89 pA/pF; +KT5823, 3.02±1.63 pA/pF; +H2O2, 3.04±1.57 pA/pF) and ODQ pretreatment (control, 3.07±1.22 pA/pF; +ODQ, 2.70±1.34 pA/pF; +H2O2, 2.74±1.21 pA/pF). The effect of 8-Br-cGMP on BKCa currents is also shown (from 3.78±0.84 pA/pF to 6.39±1.22 pA/pF, p<0.05).
To determine whether PKA is also involved in the effect of H2O2 on BKCa currents, we pretreated fibroblasts with KT5720 and H-89, well-known PKA inhibitors. KT5720 (1 µM) was added to the bath solution for 20 min, and then H2O2 (100 µM) was added. KT5720 increased BKCa currents (116.9±15.6% of the control at +50 mV, n=6, Fig. 6A); however, this difference was not significant. BKCa currents were still increased by 100 µM H2O2 in the presence of KT5720 (224.1±22.2%, n=6, p<0.01 vs. control; Fig. 6A). H-89 (1 µM) also increased BKCa currents, and the difference was significant (131.2±12.9%, n=5, p<0.05 vs. control; Fig. 6B). However, pretreatment with H-89 did not inhibit the stimulatory effect of H2O2 on BKCa currents because 100 µM H2O2 increased the BKCa currents (174.7±12.6% of the control at +50 mV). Fig. 6C shows the effects of H2O2 on the current density at +50 mV after KT5720 pretreatment (control, 3.21±0.31 pA/pF; +KT5720, 3.69±0.48 pA/pF; +H2O2, 7.21±0.66 pA/pF) and H-89 pretreatment (control, 3.35±0.41 pA/pF; +H-89, 4.56±0.41 pA/pF; +H2O2, 5.85±0.50 pA/pF).
The main finding of this study is that, among the VDKCs in HCFs, H2O2 stimulates BKCa currents but not KDR or KTO currents and that this H2O2-stimulating effect on BKCa currents is mediated by activation of protein kinase G (PKG) pathways but not the protein kinase A (PKA) pathway. In addition, H2O2-induced apoptosis of HCFs is mediated by BKCa channels.
The presence of VDKCs has been previously reported. These currents are thought regulate resting membrane potential, proliferation of ventricular fibroblasts [26], the functional expression of BKCa channels, and electrical coupling between cardiomyocytes and fibroblasts [25]. Li et al. [8] also reported the presence of three types of VDKCs in HCFs. However, we found some discrepancies between their results and ours. In their report, BKCa currents were present in most HCFs (88%), whereas KDR and KTO currents were present in a small population of cells (15 and 14%, respectively). In contrast, our results showed 47% of cells with KCa currents, 46.8% of cells with KDR currents, and 6.2% of cells with KTO currents. This difference may be due to some confusion in their report. We think that they may have miscounted KDR currents as KCa currents. Their I-V curve of KCa currents did not show the strong outward rectification that is characteristic of KCa currents, even for currents inhibited by paxilline, this may explain why the KDR current is co-present with the BKCa current in these cells. We also detected the coexistence of these three VDKCs in HCFs. To prevent confusion in identifying the types of K+ current, we defined only strongly oscillating outward K+ currents that had strong outward rectification in the I-V curve as KCa currents.
Furthermore, this earlier study [8] also showed significant mRNA expression of the KCa1.1 subunit of BKCa channels, but no mRNA expression of the 2.1 subunit of SKCa channels or the KCa3.1 subunit of IKCa was detected. In contrast, we detected mRNA expression of channel genes corresponding to the three subtypes of KCa, including clear mRNA amplification of the poreforming α subunit of KCa1.1 (BKCa) channels and the auxiliary 1.1β1, 1.1β3, and 1.1β4 subunits, the KCa2.2 and KCa2.3 subunits of SKCa channels, and the KCa3.1 subunit of IKCa channels by RT-PCR. The protein expression of BKCa, SKCa, and IKCa channels (KCa1.1, KCa2.2, KCa2.3, and KCa3.1) was also detected as prominent bands in the western blot analysis. Even though these ion channels contribute to the electrical coupling between cardiomyocytes and fibroblasts, BKCa currents have not been detected in human cardiomyocytes [825].
In our experiment, the H2O2-increased, strongly oscillating, outward rectifying KCa currents were considered BKCa currents since they were inhibited by IbTX, a BKCa- specific channel blocker. In addition, these currents were activated by high Ca2+ levels introduced using an intra-pipette solution and a high-stimulation voltage, which is the cause of strong outward rectification.
Our results showing H2O2-enhanced BKCa currents in whole-cell recordings are consistent with previous reports on vascular smooth muscle cells, human endothelial cells, and human dermal fibroblasts [92227]. In contrast, in this study, H2O2 did not stimulate voltage-gated K+ currents (KDR and KTO currents) in HCFs. These results are not consistent with previous studies in cardiomyocytes [2324] and coronary vascular smooth muscle cells [28]. These differences in the distribution of K+ currents and H2O2 effects imply variations in the functions of these channels in these two types of heart cells.
To the best of our knowledge, this is the first report showing that H2O2 enhances the BKCa currents of HCFs through PKG pathways but not through the PKA pathway. Several studies have reported that H2O2 stimulates BKCa channels through the PKG pathway in cultured human endothelial cells [22] and human coronary arterioles [29]. However, the effect of H2O2 on BKCa channels in HCFs and the underlying mechanism were not completely clear. In addition, inhibition of PKG by KT5823 and inhibition of soluble guanylate cyclase by ODQ attenuated the increase in BKCa currents following H2O2 exposure. 8-Br-cGMP, a membrane permeable analogue of cGMP, also increased BKCa currents. These results suggest that the PKG pathway is involved in the modulation of BKCa currents by H2O2 in HCFs.
Activation of BKCa channels likely elevates the intracellular Ca2+ level, which further enhances the release of NO in endothelial cells [30]. It has also been reported that NO increases BKCa currents in human dermal fibroblasts via the cGMP/PKG pathway [31] and cross-activation of the PKA pathway that stimulates currents through cGMP [32]. However, in this study, the H2O2-stimulating effects on BKCa currents were not decreased by pretreatment with KT5720 or H-89, suggesting that the PKA pathway is not involved in the modulation of BKCa currents by H2O2 in human cardiac fibroblasts. The stimulating effect of H-89 on BKCa currents was reported previously [33] and could explain the direct modulation of the currents observed here.
It is well known that K+ channel-mediated signals play an important role in cell death or apoptosis and that increased K+ efflux is one of the earliest indicators of apoptosis [1012]. The KCa currents that are associated with apoptosis have been reported in a wide variety of cells [3435]. When we pretreated cells with IbTX for 24 h before the addition of H2O2, the H2O2-induced early apoptotic changes decreased, which means that BKCa channels mediate early apoptotic cell death induced by H2O2 in HCFs. Similar results were also reported in human dermal fibroblasts [9]. We have also reported that activation of BKCa channels by its agonist, NS1619, mediates early apoptotic cell death in human dermal fibroblasts [36].
One limitation of the present study is that we cannot rule out the existence of other mechanisms for H2O2-induced cell injury in HCFs, especially the involvement of IKCa channels, since their expression was detected by RT-PCR and western blot analysis, and they also play important roles in cell proliferation [3738].
ACKNOWLEDGEMENT
This work was supported by National Research Foundation of Korea Grant funded by the Korean Government (2010-0023712).
References
1. Dixon IM, Cunnington RH. Mast cells and cardiac fibroblasts: accomplices in elevation of collagen synthesis in modulation of fibroblast phenotype. Hypertension. 2011; 58:142–144. PMID: 21730295.
2. Camelliti P, Borg TK, Kohl P. Structural and functional characterisation of cardiac fibroblasts. Cardiovasc Res. 2005; 65:40–51. PMID: 15621032.


3. Krenning G, Zeisberg EM, Kalluri R. The origin of fibroblasts and mechanism of cardiac fibrosis. J Cell Physiol. 2010; 225:631–637. PMID: 20635395.


4. Brown RD, Ambler SK, Mitchell MD, Long CS. The cardiac fibroblast: therapeutic target in myocardial remodeling and failure. Annu Rev Pharmacol Toxicol. 2005; 45:657–687. PMID: 15822192.


5. Flack EC, Lindsey ML, Squires CE, Kaplan BS, Stroud RE, Clark LL, Escobar PG, Yarbrough WM, Spinale FG. Alterations in cultured myocardial fibroblast function following the development of left ventricular failure. J Mol Cell Cardiol. 2006; 40:474–483. PMID: 16516916.
6. Kohl P, Camelliti P. Cardiac myocyte-nonmyocyte electrotonic coupling: implications for ventricular arrhythmogenesis. Heart Rhythm. 2007; 4:233–235. PMID: 17275764.


7. Kohl P, Camelliti P, Burton FL, Smith GL. Electrical coupling of fibroblasts and myocytes: relevance for cardiac propagation. J Electrocardiol. 2005; 38(4 Suppl):45–50. PMID: 16226073.


8. Li GR, Sun HY, Chen JB, Zhou Y, Tse HF, Lau CP. Characterization of multiple ion channels in cultured human cardiac fibroblasts. PLoS One. 2009; 4:e7307. PMID: 19806193.


9. Feng B, Ye WL, Ma LJ, Fang Y, Mei YA, Wei SM. Hydrogen peroxide enhanced Ca2+-activated BK currents and promoted cell injury in human dermal fibroblasts. Life Sci. 2012; 90:424–431. PMID: 22273755.
10. Jiao S, Wu MM, Hu CL, Zhang ZH, Mei YA. Melatonin receptor agonist 2-iodomelatonin prevents apoptosis of cerebellar granule neurons via K+ current inhibition. J Pineal Res. 2004; 36:109–116. PMID: 14962062.
11. Wu CT, Qi XY, Huang H, Naud P, Dawson K, Yeh YH, Harada M, Kuo CT, Nattel S. Disease and region-related cardiac fibroblast potassium current variations and potential functional significance. Cardiovasc Res. 2014; 102:487–496. PMID: 24596399.


12. Yu SP, Yeh CH, Sensi SL, Gwag BJ, Canzoniero LM, Farhangrazi ZS, Ying HS, Tian M, Dugan LL, Choi DW. Mediation of neuronal apoptosis by enhancement of outward potassium current. Science. 1997; 278:114–117. PMID: 9311914.


13. Yue L, Xie J, Nattel S. Molecular determinants of cardiac fibroblast electrical function and therapeutic implications for atrial fibrillation. Cardiovasc Res. 2011; 89:744–753. PMID: 20962103.


14. Toro L, Wallner M, Meera P, Tanaka Y. Maxi-KCa, a unique member of the voltage-gated K channel superfamily. News Physiol Sci. 1998; 13:112–117. PMID: 11390773.
15. Hoffman JF, Joiner W, Nehrke K, Potapova O, Foye K, Wickrema A. The hSK4 (KCNN4) isoform is the Ca2+-activated K+ channel (Gardos channel) in human red blood cells. Proc Natl Acad Sci U S A. 2003; 100:7366–7371. PMID: 12773623.
16. Ouadid-Ahidouch H, Roudbaraki M, Delcourt P, Ahidouch A, Joury N, Prevarskaya N. Functional and molecular identification of intermediate-conductance Ca2+-activated K+ channels in breast cancer cells: association with cell cycle progression. Am J Physiol Cell Physiol. 2004; 287:C125–C134. PMID: 14985237.
17. Sheng JZ, Braun AP. Small- and intermediate-conductance Ca2+-activated K+ channels directly control agonist-evoked nitric oxide synthesis in human vascular endothelial cells. Am J Physiol Cell Physiol. 2007; 293:C458–C467. PMID: 17459950.
18. Mathie A, Wooltorton JR, Watkins CS. Voltage-activated potassium channels in mammalian neurons and their block by novel pharmacological agents. Gen Pharmacol. 1998; 30:13–24. PMID: 9457476.


19. Park WS, Firth AL, Han J, Ko EA. Patho-, physiological roles of voltage-dependent K+ channels in pulmonary arterial smooth muscle cells. J Smooth Muscle Res. 2010; 46:89–105. PMID: 20551590.
20. Sun Y. Myocardial repair/remodelling following infarction: roles of local factors. Cardiovasc Res. 2009; 81:482–490. PMID: 19050008.


21. Angelova P, Muller W. Oxidative modulation of the transient potassium current IA by intracellular arachidonic acid in rat CA1 pyramidal neurons. Eur J Neurosci. 2006; 23:2375–2384. PMID: 16706845.
22. Dong DL, Yue P, Yang BF, Wang WH. Hydrogen peroxide stimulates the Ca2+-activated big-conductance K channels (BK) through cGMP signaling pathway in cultured human endothelial cells. Cell Physiol Biochem. 2008; 22:119–126. PMID: 18769038.


23. Dong DL, Liu Y, Zhou YH, Song WH, Wang H, Yang BF. Decreases of voltage-dependent K+ currents densities in ventricular myocytes of guinea pigs by chronic oxidant stress. Acta Pharmacol Sin. 2004; 25:751–755. PMID: 15169627.
24. Huang SY, Lu YY, Chen YC, Chen WT, Lin YK, Chen SA, Chen YJ. Hydrogen peroxide modulates electrophysiological characteristics of left atrial myocytes. Acta Cardiol Sin. 2014; 30:38–45. PMID: 27122766.
25. Wang YJ, Sung RJ, Lin MW, Wu SN. Contribution of BKCa-channel activity in human cardiac fibroblasts to electrical coupling of cardiomyocytes-fibroblasts. J Membr Biol. 2006; 213:175–185. PMID: 17483867.
26. Chilton L, Ohya S, Freed D, George E, Drobic V, Shibukawa Y, Maccannell KA, Imaizumi Y, Clark RB, Dixon IM, Giles WR. K+ currents regulate the resting membrane potential, proliferation, and contractile responses in ventricular fibroblasts and myofibroblasts. Am J Physiol Heart Circ Physiol. 2005; 288:H2931–H2939. PMID: 15653752.
27. Brzezinska AK, Gebremedhin D, Chilian WM, Kalyanaraman B, Elliott SJ. Peroxynitrite reversibly inhibits Ca2+-activated K+ channels in rat cerebral artery smooth muscle cells. Am J Physiol Heart Circ Physiol. 2000; 278:H1883–H1890. PMID: 10843885.
28. Rogers PA, Chilian WM, Bratz IN, Bryan RM Jr, Dick GM. H2O2 activates redox- and 4-aminopyridine-sensitive KV channels in coronary vascular smooth muscle. Am J Physiol Heart Circ Physiol. 2007; 292:H1404–H1411. PMID: 17071731.
29. Zhang DX, Borbouse L, Gebremedhin D, Mendoza SA, Zinkevich NS, Li R, Gutterman DD. H2O2-induced dilation in human coronary arterioles: role of protein kinase G dimerization and large-conductance Ca2+-activated K+ channel activation. Circ Res. 2012; 110:471–480. PMID: 22158710.
30. Matoba T, Shimokawa H, Nakashima M, Hirakawa Y, Mukai Y, Hirano K, Kanaide H, Takeshita A. Hydrogen peroxide is an endothelium-derived hyperpolarizing factor in mice. J Clin Invest. 2000; 106:1521–1530. PMID: 11120759.


31. Lim I, Yun J, Kim S, Lee C, Seo S, Kim T, Bang H. Nitric oxide stimulates a large-conductance Ca-activated K+ channel in human skin fibroblasts through protein kinase G pathway. Skin Pharmacol Physiol. 2005; 18:279–287. PMID: 16145282.
32. Roh S, Choi S, Lim I. Involvement of protein kinase A in nitric oxide stimulating effect on a BKCa channel of human dermal fibroblasts. J Invest Dermatol. 2007; 127:2533–2538. PMID: 17554366.
33. Park WS, Son YK, Kim N, Youm JB, Warda M, Ko JH, Ko EA, Kang SH, Kim E, Earm YE, Han J. Direct modulation of Ca2+-activated K+ current by H-89 in rabbit coronary arterial smooth muscle cells. Vascul Pharmacol. 2007; 46:105–113. PMID: 17052962.
34. Krick S, Platoshyn O, Sweeney M, McDaniel SS, Zhang S, Rubin LJ, Yuan JX. Nitric oxide induces apoptosis by activating K+ channels in pulmonary vascular smooth muscle cells. Am J Physiol Heart Circ Physiol. 2002; 282:H184–H193. PMID: 11748062.
35. Ma YG, Dong L, Ye XL, Deng CL, Cheng JH, Liu WC, Ma J, Chang YM, Xie MJ. Activation of cloned BKCa channels in nitric oxide-induced apoptosis of HEK293 cells. Apoptosis. 2010; 15:426–438. PMID: 20012488.
36. Yun J, Park H, Ko JH, Lee W, Kim K, Kim T, Shin J, Kim K, Kim K, Song JH, Noh YH, Bang H, Lim I. Expression of Ca2+ -activated K+ channels in human dermal fibroblasts and their roles in apoptosis. Skin Pharmacol Physiol. 2010; 23:91–104. PMID: 20016251.
37. Choi S, Lee W, Yun J, Seo J, Lim I. Expression of Ca-activated K channels and their role in proliferation of rat cardiac fibroblasts. Korean J Physiol Pharmacol. 2008; 12:51–58. PMID: 20157394.
38. Wang LP, Wang Y, Zhao LM, Li GR, Deng XL. Angiotensin II upregulates KCa3.1 channels and stimulates cell proliferation in rat cardiac fibroblasts. Biochem Pharmacol. 2013; 85:1486–1494. PMID: 23500546.
Fig. 1
Identification of outward K+ currents in human cardiac fibroblasts and effect of H2O2.
Representative K+ current traces were obtained by voltage step pulses from −80 mV to +50 mV for 400 ms in whole-cell mode patch clamp recordings (holding potential, −80 mV). (A) Strongly oscillating, noninactivating KCa currents are stimulated by H2O2. (B) Fast activation with slow or non-inactivation KDR currents are not stimulated by H2O2. (C) Fast activating with rapid inactivation KTO currents are not stimulated by H2O2. (D) Current densities of KCa currents, KDR currents, and KTO currents, at +50 mV from a holding potential of −80 mV, in control cells and after the addition of H2O2. *p<0.05, versus the control.
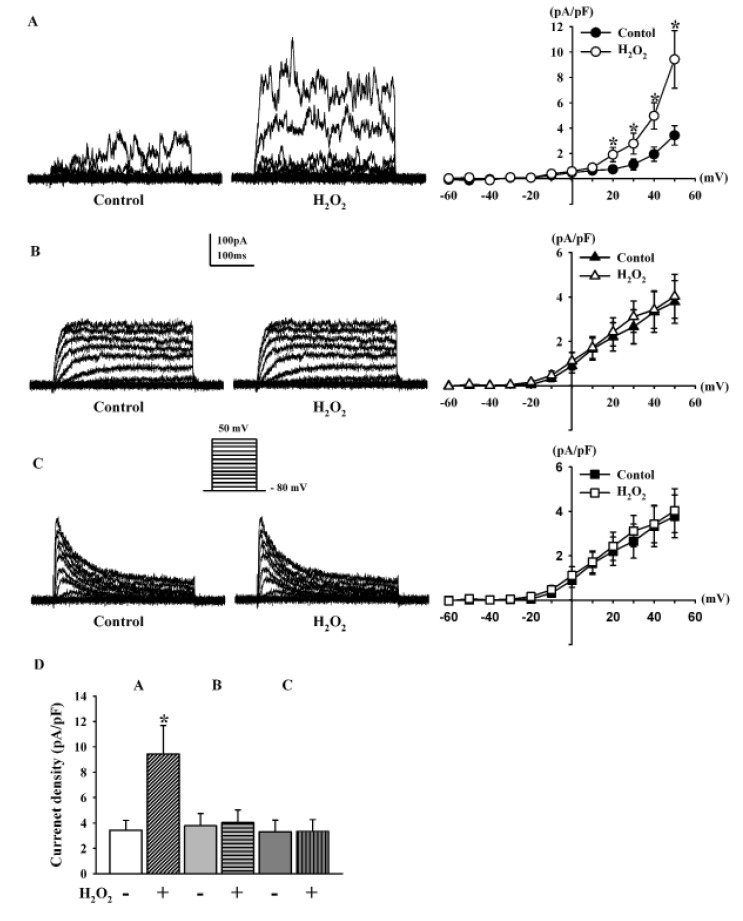
Fig. 2
Expression of various KCa channel subtypes in human cardiac fibroblasts.
(A) RT-PCR showing strong mRNA expression of KCa1.1α, KCa1.1β1, KCa1.1β3, KCa1.1β4, and KCa2.2 and weak expression of KCa2.3 and KCa3.1. GAPDH was used as control. (B) Western blot analysis showing protein expression of KCa1.1α, KCa1.1β, KCa2.2, KCa2.3, and KCa3.1. β-actin was used as a loading control.
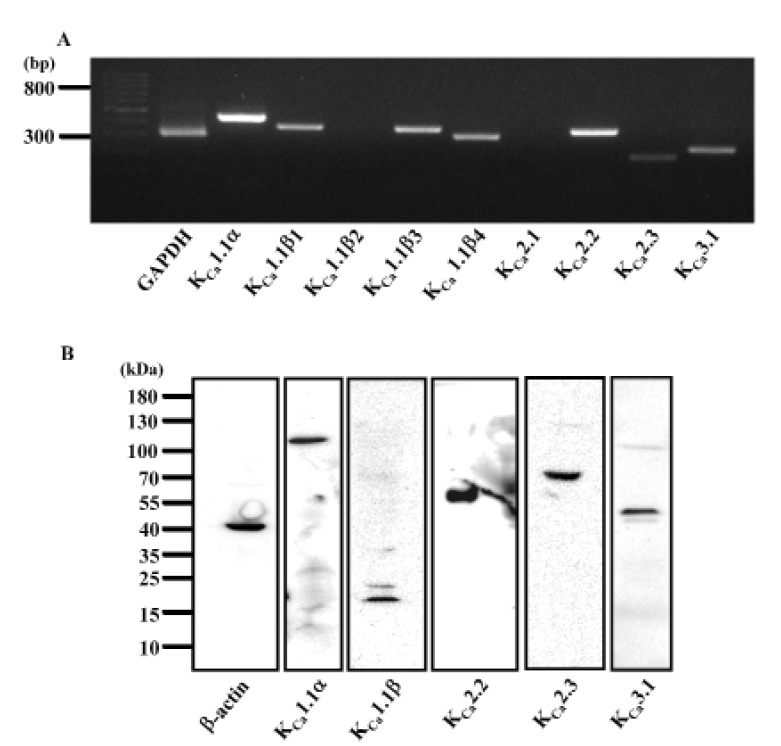
Fig. 3
Effect of iberiotoxin on outward K+ currents and H2O2–activated K+ currents in human cardiac fibroblasts.
(A) Strongly oscillating KCa currents inhibited by100 nM IbTX. (B) Addition of 100 nM IbTX blocked the H2O2-activated KCa currents (C) Bar graph summarizing the effects of IbTX, and the effect of IbTX on KCa currents after pre-incubation with H2O2. *p<0.05, versus the control; #p<0.05, versus the H2O2.
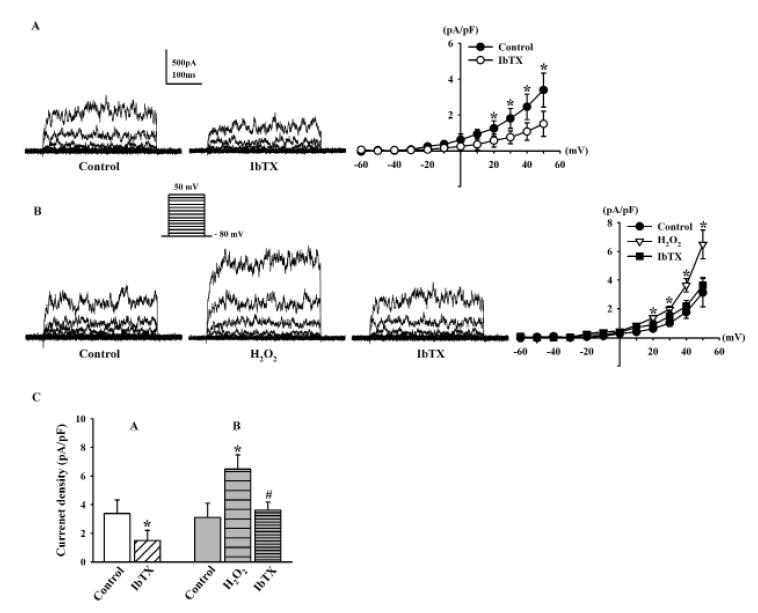
Fig. 4
Role of BKCa channels in H2O2-induced apoptosis.
Monolayer of human cardiac fibroblasts was treated by 100 µM H2O2 for 24 h. The H2O2-induced apoptosis was inhibited in the presence of 100 nM IbTX. The cytogram of the flow cytometry analysis showing the percentages of cells with specific staining patterns; In the lower left-hand quadrant (annexin V negative, PI negative) are viable cells, in the lower right-hand quadrant (annexin V positive, PI negative) are early apoptotic cells, in the upper left-hand quadrant (annexin V negative, PI positive) are dead cells, and in the upper right-hand quadrant (annexin V positive, PI positive) are late apoptotic/necrotic cells.
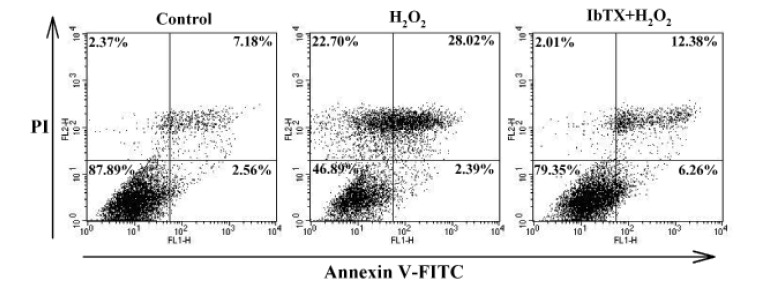
Fig. 5
Effects of PKG pathway modulation on H2O2-induced BKCa current changes.
(A, B) Representative current traces showing the effect of 100 µM H2O2 on BKCa currents in the presence of 1 µM KT5823 and 1 µM ODQ. (C) Effect of 300 µM 8-Br-cGMP on BKCa currents. (D) Bar graph summarizing the effects of H2O2 on BKCa currents after pre-incubation with KT5823 or ODQ, and the effect of 8-Br-cGMP on BKCa currents. *p<0.05, versus the control.
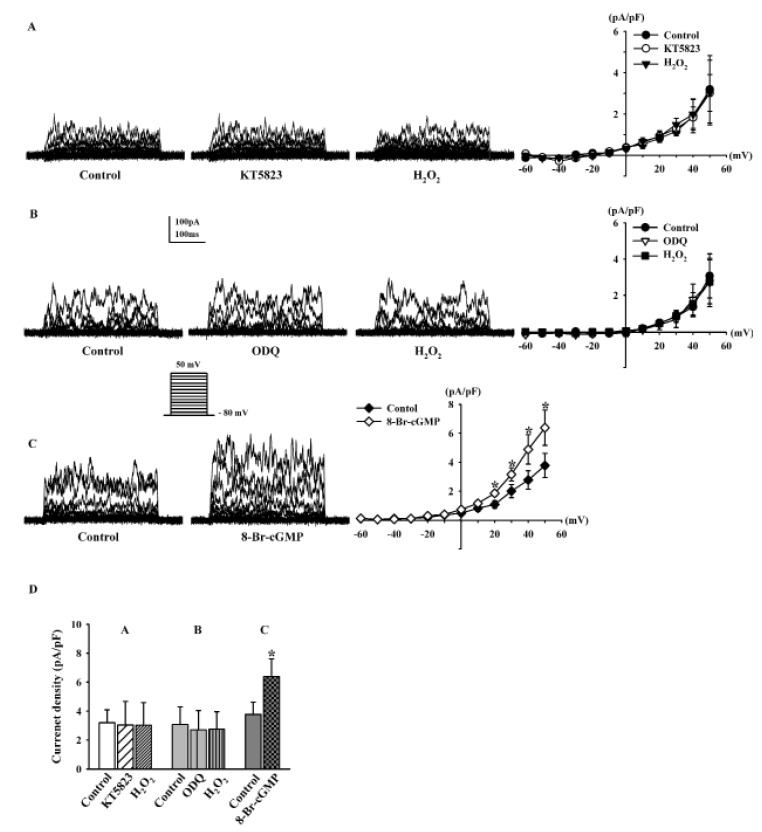
Fig. 6
Effects of PKA pathway modulation on H2O2-induced BKCa current changes.
(A, B) Representative current traces showing the effect of 100 µM H2O2 on BKCa currents after pre-incubation with 1 µM KT5720 and 1 µM H-89. (C) Bar graph summarizing the effects of H2O2 on BKCa currents in the presence of KT5720 or H-89. *p<0.05, **p<0.01 versus the control.
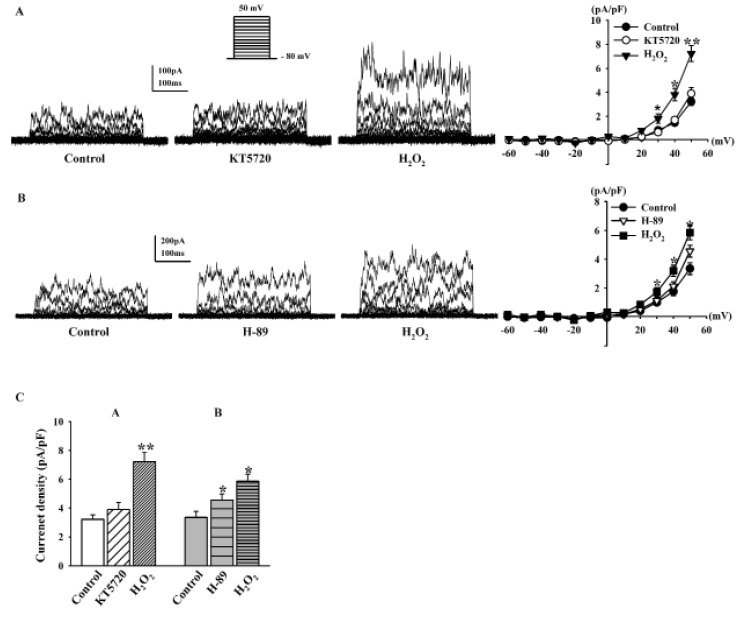
Table 2
List of primary and secondary antibodies
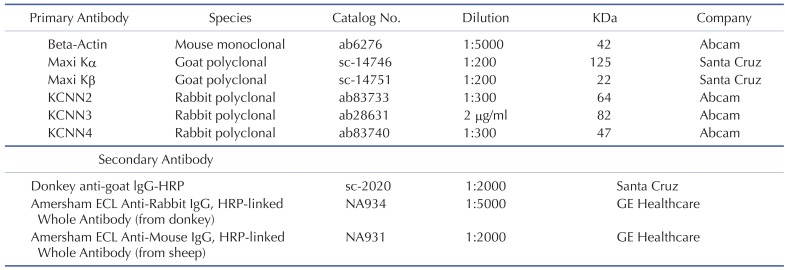
Maxi K, large conductance calcium-activated potassium channels (KCa1.1, BKCa); KCNN2, small conductance calcium-activated potassium channels (KCa2.2, SKCa2); KCNN3, small conductance calcium-activated potassium channels (KCa2.3, SKCa3); KCNN4, intermediate conductance calcium-activated potassium channels (KCa3.1, IKCa).