Abstract
Microglia, the resident macrophages in the central nervous system, can rapidly respond to pathological insults. Toll-like receptor 2 (TLR2) is a pattern recognition receptor that plays a fundamental role in pathogen recognition and activation of innate immunity. Although many previous studies have suggested that TLR2 contributes to microglial activation and subsequent pathogenesis following brain tissue injury, it is still unclear whether TLR2 has a role in microglia dynamics in the resting state or in immediate-early reaction to the injury in vivo. By using in vivo two-photon microscopy imaging and Cx3cr1GFP/+ mouse line, we first monitored the motility of microglial processes (i.e. the rate of extension and retraction) in the somatosensory cortex of living TLR2-KO and WT mice; Microglial processes in TLR2-KO mice show the similar motility to that of WT mice. We further found that microglia rapidly extend their processes to the site of local tissue injury induced by a two-photon laser ablation and that such microglial response to the brain injury was similar between WT and TLR2-KO mice. These results indicate that there are no differences in the behavior of microglial processes between TLR2-KO mice and WT mice when microglia is in the resting state or encounters local injury. Thus, TLR2 might not be essential for immediate-early microglial response to brain tissue injury in vivo.
Microglia, the resident immune cells in the CNS, constitute 5~20% of the total cells in the mammalian brain. Microglia can be activated in response to even minor pathological changes and transform function as well as morphology [12]. These activated microglia phagocytose dead cells and cellular debris [3]. Studying microglia in vitro and ex vivo, however, has a limitation in elucidating the in vivo microglia characteristics because the slicing and culture procedure itself can activate microglia [34]. Whereas, in vivo two-photon imaging studies allowed us to directly monitor the 'resting' microglia in an intact brain, and revealed that resting microglia are also highly motile; their processes continuously extend and retract to survey the microenvironment for surveillance, and once they encounter tissue damage, microglia rapidly respond by extending their processes to the injury site in the CNS [56].
Toll-like receptor 2 (TLR2) is an innate immune receptors recognizing bacterial lipoteichoic acid and lipoprotein, and triggering inflammatory responses in innate immune cells [7]. TLR2 is widely expressed in the central nervous system (CNS), particularly in microglia [8]. Previously, we have reported that TLR2 plays a critical role in microglia activation in various neurological diseases [9]. TLR2 is required for the nerve injury-induced spinal cord microglia activation and subsequent neuropathic pain development [10]. In addition, TLR2 contribute to microglia activation and hippocampal neurodegeneration in kainic acid-induced epilepsy model [11], and also in traumatic brain injury [12]. Therefore, it is likely that TLR2 is utilized by microglia to detect brain tissue damage. However, its role in immediate microglial reaction to brain tissue injury in vivo does not have been resolved. In the present study, we used in vivo two-photon microscopy and Cx3cr1GFP/+ knock-in/TLR2knock-out (KO) mice to investigate whether TLR2 plays a role in the immediate early microglial motility responding to a two-photon laser-induced local injury in the intact cortex.
All procedures were approved by the Institutional Animal Care and Use Committees of Kyung Hee University (KHUASP(SE)-14-016) and Seoul National University (SNU-140408-16). Cx3cr1GFP/+ knock-in mice were obtained from the Jackson Laboratory (Bar Harbor, USA), and TLR2 KO mice were obtained from Dr. Akira (Osaka University, Osaka, Japan).
Adult (2~4 months old) Cx3cr1GFP/+ knock-in mice and Cx3cr1GFP/+ knock-in/TLR2-KO mice were used to visualize microglia. Mice were deeply anesthetized with an intraperitoneal injection of urethane (1.64 g/kg). The animal skull was exposed above the somatosensory cortex (1 mm posterior to bregma and 2 mm lateral) and cleaned. A small circular craniotomy (~2 mm in diameter) was then carefully performed using a high-speed drill, which was covered with a thin glass cover slip [1314]. For thinned-skull cranial window, the skull was thinned with high-speed drill and scraped with a microsurgical blade to a thickness of ~20 µm [15].
Imaging was performed with a two-photon microscope (Zeiss LSM 7 MP, Carl Zeiss). A Ti:sapphire laser (Chameleon, Coherent) was tuned to the excitation wavelength for GFP (900~950 nm) [13]. Subsequent image stacks (512×512 pixels, 0.4 µm/pixel, 26 sections) were recorded every 5 min for 1~2 hour. The imaging depth was 50~150 µm from the pial surface.
Laser ablation was induced by focusing a two-photon laser beam (800 nm, 70 mW) in the somatosensory cortex (target: microglia soma, spot size: 15×15 µm) to create a small injury site as indicated by an autofluorescent sphere [6].
Image processing was done using ImageJ (http://rsbweb.nih.gov/ij). The length of processes was evaluated from maximum-intensity projections and the motility was calculated as absolute Δlength in comparison between two successive imaging sessions.
In laser ablation experiments, we quantified the degree of the invasion of microglial processes to the injured site as described previously [6]. Briefly, the number of microglial processes entering from the outer region Y (70 µm in radius) into the inner region X (35 µm in radius) surrounding the lesion site was measured before and after the laser ablation. We counted the pixels containing GFP signal in the region X over time [Rx(t)], from which Rx(0), the GFP pixels in X immediately after the laser ablation, was subtracted. This subtracted value was then divided by Ry(0), the GFP pixels in the region Y immediately after the ablation. The microglial response, R(t), is thus provided by R(t)=(Rx(t)-Rx(0))/Ry(0).
All data are presented as mean±SEM. Statistical analysis and graphic works were done with Prism 5.0 (Graph Pad Software, USA). Unpaired t test or two-way repeated measures analysis of variance (ANOVA) followed by Dunnett's multiple comparison test were used. In all cases, p<0.05 was considered significant.
By using in vivo two-photon microscopy imaging through a cranial window, we first observed the resting state dynamics of microglial processes for an hour in the intact somatosensory cortex of TLR2-KO or WT mice. As shown in Fig. 1A and 1B, the resting microglia in the both groups of mice had a small rod-shaped soma and ramified processes. Their processes continually extended and retracted, but microglial soma remained fixed (Fig. 1A and 1B). To quantify the motility (i.e. extension and retraction) of resting microglial processes, we measured the length changes of individual processes every five minutes. Fig. 1c shows that there was no significant difference in the motility of microglial processes between the TLR2-KO and WT mice (p>0.05). Imaging through a thinned-skull cranial window showed a similar shape and motility of microglia in WT mice to that obtained through an open-skull window (Fig. 2), indicating that our craniotomy procedure does not alter a physiological condition of microglia.
By using a two-photon laser ablation method, we next examined an immediate response of microglia to a local brain injury in TLR2-KO and WT mice. As shown in Fig. 3A, this local injury was developed after a focal two-photon laser stimulus was applied to a microglia soma, and then nearby microglia rapidly sent out their processes to the lesion site in both groups of mice. More specifically, within a few minutes after a laser ablation, the microglial processes close to the damaged site appeared slightly enlarged and bulbous. After the next few minutes, microglial processes reached to the damaged site (Supplementary Videos 1 and 2). To quantitatively compare the microglial response to laser-induced injury in TLR2-KO and WT mice, we counted the number of pixels entering from the outer area Y into the inner area X with time (Fig. 3B and see also Methods). Fig. 3C shows that there was no significant difference in the microglial response to the injury between the TLR2-KO and WT mice (p>0.05).
In the present study, we first investigated whether the 'resting' state microglia behave differently between the TLR2-KO and WT mice. Irrespective of the presence of TLR2 signaling, their processes continually extended and retracted, in consistent with a previous report [5], with no significant difference in the motility between the two groups of mice (Fig. 1). These results indicate that the microglial behavior in the resting state is not dependent on TLR2 signaling. Although an open-skull preparation for long-term in vivo two-photon imaging is reported to gradually cause glial activation [16], our experimental conditions using acute and short-term two-photon imaging of microglia that located 50~150 µm from the pial surface showed no difference in the morphology and motility of resting microglia between an thinned-skull preparation and an open-skull window (Fig. 2).
Our previous studies have reported that TLR2 has a critical role in microglial activation and pathogenesis in the CNS [101112]. Other studies also demonstrated that TLR2-mediated signaling promotes the production of multiple inflammatory mediators such as interleukin (IL)-1, IL-6, tumor necrosis factor (TNF)-α and nitric oxide (NO) [171819]. In addition, axonal injury in the mouse brain induced early cytokine/chemokine expressions (e.g. TNF-α, CXCL10), T cell infiltration and microglial expansion, all of which were significantly reduced in TLR2-KO mice [20]. These results indicate that microglia activated through TLR2 signaling induces neuroinflammation and neurite degeneration. In contrast, a recent ex vivo study [21] showed that a TLR2 agonist, Pam2CSK4, increased the microglial response to laser-induced spinal cord injury, reduced proximal axonal dieback and secondary axonal degeneration, and induced an alternative microglial activation profile, suggesting that this TLR2-mediated microglial activation might be protective. However, other TLR2 agonists had little effect on such microglial responses and axon degeneration, and the contrary effect of TLR2-KO seemed to be very slight [21]. Thus, the role of TLR2-mediated microglial activation in the pathological states is still controversial. Furthermore, it remains to be unclear whether TLR2 has a role in immediate microglial reaction to a local brain injury in vivo.
In the second set of the experiments, we took advantage of the focal properties of the two-photon microscopy laser [6] to identify how microglia immediately react to local brain injury in TLR2-KO and WT mice. Unexpectedly, we found that TLR2-KO mice exhibited a similar microglial response to the injury when compared to that of WT mice (Fig. 3). These results also indicate that the immediate microglial reaction to a local brain injury is not dependent on TLR2 signaling.
In conclusion, this study demonstrates that the microglial process of TLR2-KO mice showed similar level of motility to that of WT mice in the 'resting' state. Following a two-photon laser ablation-induced focal injury, the microglial response motility to the injury is also similar between WT and TLR2-KO mice. Therefore, our findings suggest that TLR2 signaling does not have an essential role in the resting state microglial behavior as well as in the immediate early microglial response to a brain tissue injury in vivo.
ACKNOWLEDGEMENTS
This work was supported by National Research Foundation of Korea grants funded by the Korea government (NRF-2013R1A1A1012403 to S.K.Kim, and 2013R1A1A20-74231 and 2013R1A2A2A01067248 to S.J.Lee, and NRF-2011-0030737 to S.J.Kim). Funders had no involvement in study design, and in the collection, analysis and interpretation of data, and in the writing of the manuscript, and in the decision to submit the manuscript for publication.
Notes
AUTHORS' CONTRIBUTIONS: SJL conceived the study. SKK, SJK and SJL designed the experiments. HY performed the main experimental work and the analysis of the results. SKK and SJK supervised the in vivo two-photon imaging experiments and the analysis of the results. YHJ and SJL maintained and provided TLR2-KO and Cx3cr1GFP/+ mice. HY, SKK, SJK and SJL interpreted the data and wrote the manuscript. All authors read and approved the final manuscript.
References
1. Hanisch UK, Kettenmann H. Microglia: active sensor and versatile effector cells in the normal and pathologic brain. Nat Neurosci. 2007; 10:1387–1394. PMID: 17965659.


3. Petersen MA, Dailey ME. Diverse microglial motility behaviors during clearance of dead cells in hippocampal slices. Glia. 2004; 46:195–206. PMID: 15042586.


4. Stence N, Waite M, Dailey ME. Dynamics of microglial activation: a confocal time-lapse analysis in hippocampal slices. Glia. 2001; 33:256–266. PMID: 11241743.


5. Nimmerjahn A, Kirchhoff F, Helmchen F. Resting microglial cells are highly dynamic surveillants of brain parenchyma in vivo. Science. 2005; 308:1314–1318. PMID: 15831717.


6. Davalos D, Grutzendler J, Yang G, Kim JV, Zuo Y, Jung S, Littman DR, Dustin ML, Gan WB. ATP mediates rapid microglial response to local brain injury in vivo. Nat Neurosci. 2005; 8:752–758. PMID: 15895084.
7. Kawai T, Akira S. The role of pattern-recognition receptors in innate immunity: update on Toll-like receptors. Nat Immunol. 2010; 11:373–384. PMID: 20404851.


8. Lehnardt S, Henneke P, Lien E, Kasper DL, Volpe JJ, Bechmann I, Nitsch R, Weber JR, Golenbock DT, Vartanian T. A mechanism for neurodegeneration induced by group B streptococci through activation of the TLR2/MyD88 pathway in microglia. J Immunol. 2006; 177:583–592. PMID: 16785556.


9. Hayward JH, Lee SJ. A Decade of research on TLR2 discovering its pivotal role in glial activation and neuroinflammation in neurodegenerative diseases. Exp Neurobiol. 2014; 23:138–147. PMID: 24963278.


10. Kim D, Kim MA, Cho IH, Kim MS, Lee S, Jo EK, Choi SY, Park K, Kim JS, Akira S, Na HS, Oh SB, Lee SJ. A critical role of toll-like receptor 2 in nerve injury-induced spinal cord glial cell activation and pain hypersensitivity. J Biol Chem. 2007; 282:14975–14983. PMID: 17355971.


11. Hong J, Cho IH, Kwak KI, Suh EC, Seo J, Min HJ, Choi SY, Kim CH, Park SH, Jo EK, Lee S, Lee KE, Lee SJ. Microglial Toll-like receptor 2 contributes to kainic acid-induced glial activation and hippocampal neuronal cell death. J Biol Chem. 2010; 285:39447–39457. PMID: 20923777.


12. Park C, Cho IH, Kim D, Jo EK, Choi SY, Oh SB, Park K, Kim JS, Lee SJ. Toll-like receptor 2 contributes to glial cell activation and heme oxygenase-1 expression in traumatic brain injury. Neurosci Lett. 2008; 431:123–128. PMID: 18164130.


13. Wake H, Moorhouse AJ, Jinno S, Kohsaka S, Nabekura J. Resting microglia directly monitor the functional state of synapses in vivo and determine the fate of ischemic terminals. J Neurosci. 2009; 29:3974–3980. PMID: 19339593.
14. Holtmaat A, Bonhoeffer T, Chow DK, Chuckowree J, De Paola V, Hofer SB, Hübener M, Keck T, Knott G, Lee WC, Mostany R, Mrsic-Flogel TD, Nedivi E, Portera-Cailliau C, Svoboda K, Trachtenberg JT, Wilbrecht L. Long-term, high-resolution imaging in the mouse neocortex through a chronic cranial window. Nat Protoc. 2009; 4:1128–1144. PMID: 19617885.


15. Yang G, Pan F, Parkhurst CN, Grutzendler J, Gan WB. Thinned-skull cranial window technique for long-term imaging of the cortex in live mice. Nat Protoc. 2010; 5:201–208. PMID: 20134419.


16. Xu HT, Pan F, Yang G, Gan WB. Choice of cranial window type for in vivo imaging affects dendritic spine turnover in the cortex. Nat Neurosci. 2007; 10:549–551. PMID: 17417634.
17. Chao CC, Hu S, Molitor TW, Shaskan EG, Peterson PK. Activated microglia mediate neuronal cell injury via a nitric oxide mechanism. J Immunol. 1992; 149:2736–2741. PMID: 1383325.
18. Giulian D, Vaca K, Corpuz M. Brain glia release factors with opposing actions upon neuronal survival. J Neurosci. 1993; 13:29–37. PMID: 8423475.


19. Hur J, Lee P, Kim MJ, Cho YW. Regulatory effect of 25-hydroxyvitamin D3 on nitric oxide production in activated microglia. Korean J Physiol Pharmacol. 2014; 18:397–402. PMID: 25352759.
20. Babcock AA, Wirenfeldt M, Holm T, Nielsen HH, Dissing-Olesen L, Toft-Hansen H, Millward JM, Landmann R, Rivest S, Finsen B, Owens T. Toll-like receptor 2 signaling in response to brain injury: an innate bridge to neuroinflammation. J Neurosci. 2006; 26:12826–12837. PMID: 17151286.


21. Stirling DP, Cummins K, Mishra M, Teo W, Yong VW, Stys P. Toll-like receptor 2-mediated alternative activation of microglia is protective after spinal cord injury. Brain. 2014; 137:707–723. PMID: 24369381.


SUPPLEMENTARY MATERIALS
Supplementary data including one video can be found with this article online at http://dx.doi.org/10.4196/kjpp.2015.19.5.461.
Fig. 1
The motility of microglial processes in TLR2-KO and WT mice. (A, B) Representative images for the maximum-intensity projections of an individual microglia from 0 min to 60 min after the starting of two-photon time series imaging in WT (A) and TLR2-KO (B) mice. Arrowheads indicate the extension (red) and retraction (white) of microglial processe (open: previous process; filled: extended or retracted one). (C) Length changes of microglial processes (extension and retraction) in TLR2-KO (n=87 processes/7 cells/3 mice) and WT (n=105 processes/9 cells/4 mice) mice. ns, no significant difference between the two groups. Data are presented as mean±SEM.
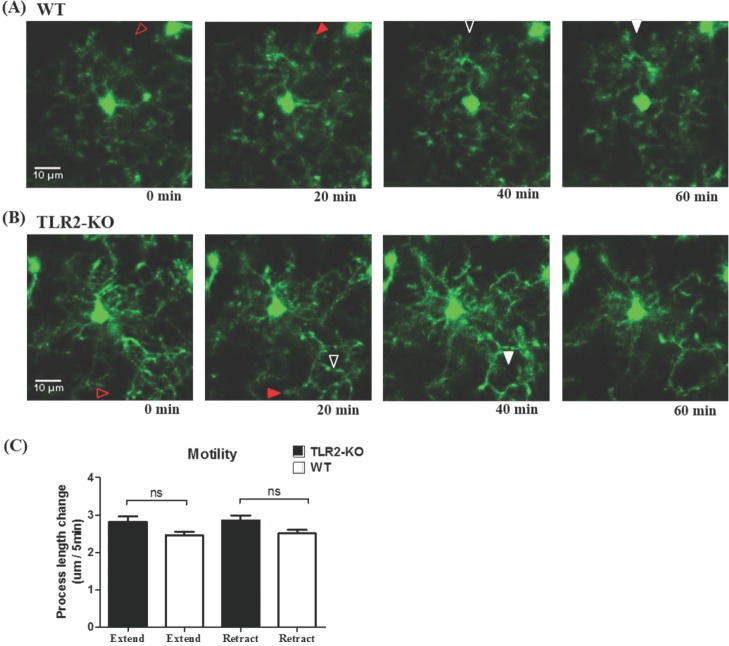
Fig. 2
The movement of microglial processes through open-skull or thinned-skull cranial window in WT mice. (A) Representative image for the maximum-intensity projections of an individual microglia from 0 min to 60 min after the starting of two-photon time series imaging through openskull or thinned-skull cranial window in WT mice. A thinned-skull prep; thinned with a high-speed drill and then scraped with a microsurgical blade. (B) The motility of microglial processes using thinned-skull prep (n=78 processes/7 cells/4 mice) and opened-skull prep (n=105 processes/9 cells/4 mice) in WT mice. No significant difference was observed between the two groups. Data are presented as mean±SEM.
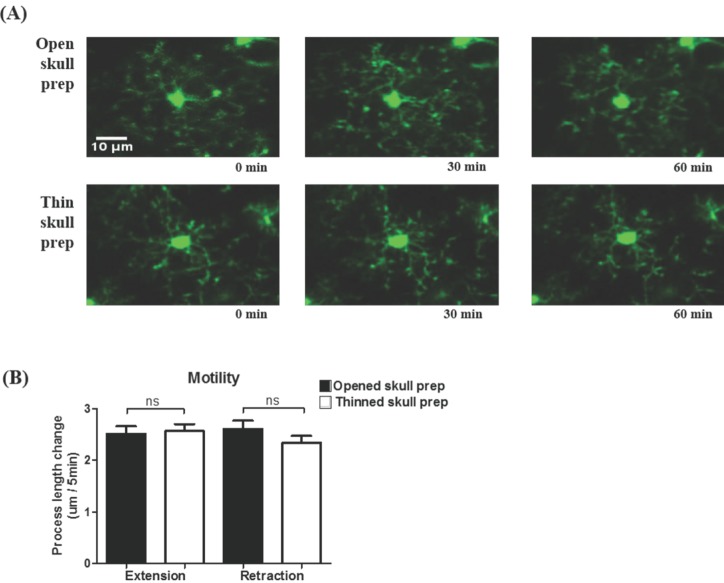
Fig. 3
The microglial response to brain injury in TLR2-KO and WT mice. (A) Representative images for the microglial response to brain tissue injury with time (-30, 0, 60, 120 min post-injury) in WT and TLR2-KO mice. (B) To quantify the microglial response toward laser-induced injury, we measured the number of pixels entering from the outer area Y (70 µm in radius) into the inner area X (35 µm in radius). The number of GFP pixels in area X or Y were measured at each time point (Rx(t) or Ry(t)), and the microglial response was defined as R(t)=(Rx(t)-Rx(0))/Ry(0). (C) Quantification of microglial response to laser ablation in TLR2KO (n=3) and WT (n=3) mice. Data are presented as mean±SEM.
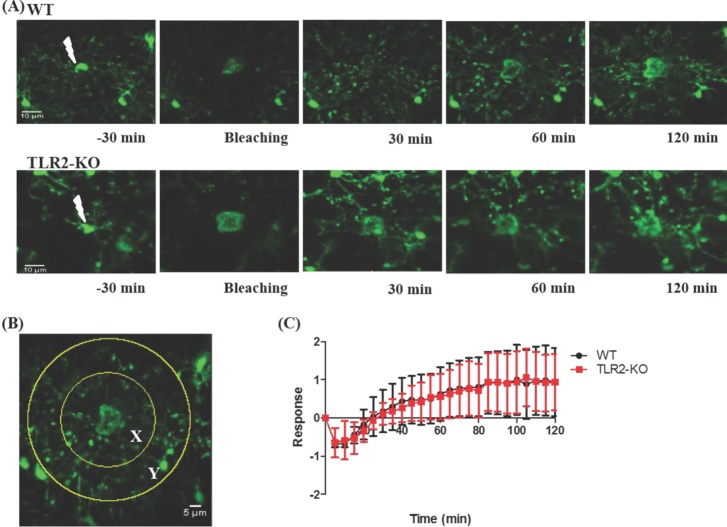