Abstract
This study surveys the improvement characteristics in old-aged muscular mitochondria by bio-active materials coated fabric (BMCF). To observe the effects, the fabric (10 and 30%) was worn to old-aged rat then the oxygen consumption efficiency and copy numbers of mitochondria, and mRNA expression of apoptosis- and mitophagy-related genes were verified. By wearing the BMCF, the oxidative respiration significantly increased when using the 30% materials coated fabric. The mitochondrial DNA copy number significantly decreased and subsequently recovered in a dose-dependent manner. The respiratory control ratio to mitochondrial DNA copy number showed a dose-dependent increment. As times passed, Bax, caspase 9, PGC-1α and β-actin increased, and Bcl-2 decreased in a dose-dependent manner. However, the BMCF can be seen to have had no effect on Fas receptor. PINK1 expression did not change considerably and was inclined to decrease in control group, but the expression was down-regulated then subsequently increased with the use of the BMCF in a dose-dependent manner. Caspase 3 increased and subsequently decreased in a dose-dependent manner. These results suggest that the BMCF invigorates mitophagy and improves mitochondrial oxidative respiration in skeletal muscle, and in early stage of apoptosis induced by the BMCF is not related to extrinsic death-receptor mediated but mitochondria-mediated signaling pathway.
Recently, the functionalized bio-active textile products have been increasingly developed [1,2,3]. For examples, the use of special silk clothes may be useful in the management of atopic dermatitis in children [4]. Lee et al. [5] showed that multi-functional fabric wear is effective in inducing deep sleep, increasing growth hormone, and improving the quality of sleep. Also, they suggested that multi-functional fabric may act to stabilize both the autonomic nervous system and psychological state [6]. GERMACOLOR (Germany) and VENTE (Korea) collaborated to develop the fabric radiating bio-active energy. The bio-active materials coated fabric (BMCF) was coated with bio-active materials containing over 30 kinds of minerals. The clothing made of this fabric has layer of bio-active energy which reacts with far infrared rays from human body and it is transferred into human body. Advanced research has shown the improvement characteristics in muscular maximal exercise performance and recovery from muscle fatigue when using this testing product. And, any kind of unusual skin reaction has not been detected in safety evaluation (data not shown). However, the study for intra-cellular mechanisms of signaling pathway induced by this functionalized textile has not been enough.
Aged muscles retain dysfunctional fiber segments which atrophy and break contributing to the loss of muscle mass and function [7]. Recent studies have shown that mitochondria play a major role in the regulation of myogenesis [8,9,10]. The abundance, morphology, and functional properties of mitochondria become altered when the myoblasts differentiate into myotubes [11,12,13]. Mitochondria are vital organelles for the energy production and for the regulation of signaling cascades in skeletal muscle. Mitochondria generate adenosine triphosphate (ATP), which is used as a source of chemical energy, and control the cell cycle and growth by involving in cellular signaling pathway [14]. Thus, a variety of disorders can be caused by oxidative stress, damage and subsequent dysfunction in mitochondria [15].
Apoptosis is mainly divided into two of pathway, extrinsic and intrinsic. The extrinsic pathway is occurred by cell surface death receptors such as Fas receptor (FasR). On the other hand, the intrinsic pathway is occurred by mitochondria-mediated signaling cascade, which release cytochrome c (cyt c) caused by mitochondrial permeability transition pore (mPTP) into cytosol [16,17]. Autophagy has crucial roles in the cellular adaptation to stress as a quality control mechanism [18]. Thus, autophagy has relation to an essential cytoprotective pathway and a potential anti-aging mechanism [19]. Autophagy may occur either as a general phenomenon, for instance when cells lack nutrients and mobilize their energy reserves, or it can specifically target cellular structures such as damaged mitochondria (mitophagy). Because of the danger of having damaged mitochondria in the cell, the timely elimination of damaged and aged mitochondria is essential for maintaining the integrity of the cell. This turnover process consists of the sequestration and hydrolytic degradation by the lysosome, a process also known as mitophagy. Mitochondrial biogenesis is characterized as a vital process in the synthesis and degradation of the organelle [20]. This fundamental process comprehends the synthesis import and incorporation of lipids and proteins to the existing mitochondrial reticulum; the stoichiometric assembly of multi-subunit protein complexes into a functional respiratory chain; replication of the mitochondrial DNA; selective degradation of mitochondria by autophagy [21,22,23].
In this study, we examined the effects of the BMCF on skeletal muscular mitochondria. To observe the effects, the fabric was put on old-aged rat then the oxygen consumption efficiency and copy numbers of mitochondria, and mRNA expression of apoptosis- and mitophagy-related genes were verified as times passed.
Sprague-Dawley rats (12 weeks, male) weighing 350±15 g were obtained from Samtako (Osan, Korea). The animals were allowed to acclimatize for 1 week before the experiments and were housed in a standard, controlled environment with a temperature of 22±3℃, humidity of 50±10%, 12 h light-dark cycle, and a ventilation 10~15 times/h with wind velocity of 10~20 cm/sec. The animals were fed filtered tap water and purified pellet diet (Dong A One Corporation Ltd., Korea). The protocols used conformed to the National Institutes of Health Guide for the Care and Use of Laboratory Animals and were approved by the Ethics Committee for Laboratory Animals of Chung-Ang University (approval number: 14-0023). The animals were divided into one control group (eight animals) and two experimental groups (eight animals each). Cloth made from conventional fabric (V-Con) was used for the control group while 10% (V-10) and 30% (V-30) BMCF were used for each experimental group.
The fabric samples were provided by VENTEX. The bio-active material contained 30 kinds of minerals, such as SiO2, Mg, Al2O3, Na, Ca, and Fe2O3, and was blended with acrylic resin at the desired ratio and coated on one side of the fabric. GERMACOLOR and VENTEX collaborated to develop this fabric, and the clothing made from this fabric has a layer that radiates bio-active energy. The bio-active materials radiate unique energy generated according to the molecular structure and vibration of each element. The energy reacts with far infrared rays emanating from the human body and is transferred into the human body.
The animals were sacrificed through ether inhalation anesthesia and cardiac puncture. After euthanasia was administered, the subtrapezial muscle was rapidly removed and was washed at least three times in PBS. The mitochondria fraction was obtained according to the modified methods described by Frezza et al. [24]. The muscles were homogenized in a homogenizer (WiseTis, Korea) with 3 ml MIB1 (KCl 180 mM, Na2-EDTA 0.5 mM, Tris 10 mM, pH 7.4). The resulting homogenate was centrifuged at 1,000×g for 10 min at 4℃, and the supernatant was collected. The supernatant was centrifuged once again at 1,000×g for 10 min at 4℃, and the supernatant was again collected. Finally, the supernatant was centrifuged at 10,000×g for 10 min at 4℃, and the supernatant was discarded. A mitochondria-enriched pellet was re-suspended in 1 ml MIB2 (KCl 180 mM, Na2EDTA 0.5 mM, Tris 10 mM, BSA 1 g/L, pH 7.4) and was centrifuged at 10,000×g for 10 min at 4℃. The mitochondria-enriched pellet was then ready for the oxygen consumption measurement.
The mitochondrial oxygen consumption was measured according to the modified methods described by Frezza et al. [24]. The measurement of the respiratory control rates was performed with 20 mM ADP, G/M (5 mM Glutamate, 2.5 mM Malate), MIR05 (EGTA 0.5 mM, MgCl2-6H2O 3 mM, K-lactobionate 60 mM, taurine 20 mM, KH2PO4 10 mM, HEPES 20 mM, sucrose 110 mM, BSA 1 g/L, pH 7.1). Oxygen consumption was performed in a NeoFox chamber (Ocean Optics Inc., Dunedin, USA) and was monitored with a NeoFox viewer (version 2.30, Ocean Optics Inc.) program. The respiratory control ratio (RCR) is the ratio of respiration between the presence of ADP (state 3) and the absence of ADP (state 4).
DNA was extracted from rat subtrapezial muscle using the QIAamp DNA Mini Kit (Qiagen, Germany) according to the product instruction manual. The extracted DNA contained two kinds of DNA: genomic DNA (gDNA) and mitochondrial DNA (mtDNA). RNA was extracted from rat subtrapezial muscle tissue using TRIzol reagent (Invitrogen Life Technologies, USA) according to the product instruction manual. The quantity of total RNA was determined using a NanoDrop 1000 (Thermo Scientific, USA). The total RNA was reverse-transcribed to cDNA using the RNA to cDNA Ecodry™ premix (oligo dT) kit (TaKaRa, Japan) with 2,000 ng of RNA according to the product instruction manual.
The sequences for the mitochondrial genome and Glyceraldehyde-3-phosphate dehydrogenase (GAPDH) primers are shown in Table 1. Each real-time PCR reaction mixture included 0.4 µl MgCl2 (final concentration 2 mM), 0.5 µl forward primer (final concentration 0.5 µM), 0.5 µl reverse primer (final concentration 0.5 µM), 1 µl LightCycler DNA Master SYBR Green I 10×(Roche, Germany), DNA template (15 ng) and up to 10 µl of distilled water (DW). Real-time PCR was performed in a LightCycler 2.0 (Roche). The real-time PCR conditions were as follows: denaturation (95℃ for 10 min), a three-segment amplification program repeated 35 times (95℃ for 10 sec; 62℃ for 10 sec; 72℃ for 10 sec), melting curve program (65℃ to 95℃ with a heating rate of 0.1℃/sec) and cooling down (40℃). The results were expressed as the ratio between mtDNA and gDNA. The mtDNA and gDNA Ct ratios were compared according to the modified methods described by Guo et al. [25].
The mRNA expression of apoptosis-related genes was verified via RT-PCR experiment. The sequences for B-cell lymphoma 2 gene (Bcl-2), Bcl-2-associated X protein (Bax), PTEN-induced putative kinase 1 (PINK1), beta-actin (β-actin), Peroxisome proliferator-activated receptor gamma coactivator 1 alpha (PGC-1α) and 18S ribosomal RNA (18S) primers are described in Table 1. The RT-PCR conditions were as follows: denaturation (95℃ for 10 min), a three-segment amplification program repeated 30 times (95℃ for 1 min; each annealing temperature for 1 min; 72℃ for 1 min) and final extension (72℃ for 5 min). Electrophoresis was carried out for the PCR products (Mupid-exU, ADVANCE, Japan) in 1.2% agarose gel. Real-time PCR was performed with the cDNA templates. The sequences for caspase 9 (CASP9), caspase 3 (CASP3), Bax, Bcl-2, β-actin and FasR for real-time PCR primers are described in Table 1. Each reaction mixture contained 0.8 µl MgCl2 (final concentration 3 mM), 0.2 µl forward primer (final concentration 0.2 µM), 0.2 µl reverse primer (final concentration 0.2 µM), 1 µl LightCycler DNA Master SYBR Green I 10 X, 1 µl cDNA template and up to 10 µl DW.
The oxygen consumption significantly increased in the 1st (p<0.001) and 4th (p<0.01) weeks when using the 30% BMCF (Figure 1). The mtDNA copy number significantly decreased with the 30% BMCF in the 1st week (p<0.01) and increased between the 2nd and 4th weeks (Figure 2). The 10% BMCF showed a decrease in the mtDNA copy number between the 2nd and 3rd weeks and a subsequent recovery in the 4th week. However, in the control group, no changes were shown in the mitochondrial oxygen consumption, and the mtDNA copy number slightly decreased as time passed throughout all 4 weeks. The RCR to mtDNA copy number during the 4 weeks showed a dose-dependent increment with the use of the BMCF (p<0.001) (Figure 3). These results suggested that the BMCF invigorate mitophagy and improve mitochondrial oxidative respiration efficiently in skeletal muscle.
The changes in mRNA expression of Bcl-2, Bax, PINK1 and PGC-1α were confirmed via RT-PCR experiment in a time-course experiment with duration of 4 weeks (Figure 4). In addition, Bcl-2, Bax, CASP3, CASP9 and FasR were confirmed via real-time PCR experiment (Figure 5). In Figure 4, as times passed, Bax expression increased in a dose-dependent manner, although Bcl-2 decreased. PINK1 expression did not change considerably and was inclined to decrease in control group. However, with the use of the BMCF, mRNA expression of PINK1 was down-regulated and subsequently increased in a dose-dependent manner. PGC-1α expression increased alike in 10% and 30% BMCF group compare to control group. β-actin was used as a control marker as 18S, but it also increased with the use of the BMCF. In the results of real-time PCR experiment (Figure 5), the BMCF can be seen to have had no effect on FasR even though Bax and CASP9 expression increased, and also Bcl-2 decreased as former results. CASP3 increased in the 1st week then decreased between the 2nd and 4th weeks in V-30 group, and increased till the 3rd week then decreased in the 4th week in V-10 group, which showing dose-dependent manner. The increment in β-actin due to the use of the BMCF was re-confirmed via real-time PCR with different primer sets. The results of mRNA expression analysis suggest that altered mtDNA copy number is closely related to mitochondrial autophagy and the apoptosis induced by the BMCF is not related to extrinsic death-receptor mediated but mitochondria-mediated signaling pathway.
The results of current investigation demonstrated that BMCF provided by VENTEX leads to the stimulation of mitochondrial biogenesis in a dose-dependent manner as evidenced by: increased oxidative respiration of mitochondria, decrement followed by a recovery of mtDNA copy number, improved RCR to mtDNA copy number, increased Bax, CASP9, and PGC-1α, and decreased Bcl-2 expression.
Including mitochondrial mass/volume, mtDNA copy number and mitochondrial respiration are markedly increased at the beginning of myogenic differentiation [26,27,28]. In this study, by wearing the BMCF, the oxidative respiration significantly increased and the mtDNA copy number markedly decreased then recovered as times passed (Figure 2), and RCR to mtDNA copy number during the 4 weeks showed a dose-dependent increment (Figure 3). These results suggest that the BMCF invigorates mitochondrial biogenesis and leads to improvement of mitochondrial oxidative respiration in skeletal muscle.
Bcl-2 and Bax are inversely related to fluctuation of apoptosis and adjust release of cyt c which is known as critical trigger to induce apoptosis signaling cascade [29,30]. Activated Bax induces intrinsic apoptosis signaling pathway using mitochondria intermembrane space generates apoptosis by releasing cyt c to outside, although Bcl-2 blocks the release of cyt c from mitochondria. Also, Bcl-2 plays crucial roles in mitochondria membrane permeability transition with Bax. Decrease in Bcl-2 function and increase in Bax protein levels may be responsible for the release of cyt c from mitochondria [31]. In this study, the mRNA expression of Bax increased in a dose-dependent manner although Bcl-2 decreased, which suggested the BMCF has the facilitating role in mitochondria-mediated intrinsic apoptosis signaling pathway (Figure 4, 5C&D).
PGC-1α co-activates the transcription factors that control expression of genes encoding mitochondrial proteins and, thus, stimulates mitochondrial biogenesis [32,33,34]. In this study, the BMCF upregulated the mRNA expression level of PGC-1α (Figure 4). There are several ways to induce mitophagy in mammalian cells. The PINK1 and Parkin pathway is, so far, the best characterized. This pathway starts in by distinguishing the difference between healthy mitochondria and damaged one. PINK1 has been implicated to detect mitochondria quality [35]. In this study, PINK1 expression did not change considerably and was inclined to decrease in control group, but the expression was down-regulated then subsequently increased with the use of the BMCF in a dose-dependent manner (Figure 4). The result suggests that PINK1-mediated mitochondrial quality control pathway has been triggered by using the BMCF, because the alteration of PINK1 expression and mtDNA copy number showed similar tendency. The mRNA expression level of CASP3 increased in the 1st week then decreased between the 2nd and 4th weeks in V-30 group, and increased till the 3rd week then decreased in the 4th week in V-10 group, which showing dose-dependent manner, which showed diametrically opposite result from PINK1 (Figure 5B). Taken together, the BMCF activated PINK1-mediated mitochondrial quality control pathway and this damaged mitochondrial-targeted PINK1 inhibited apoptosis and triggered mitophagy such as previously reported [36].
Activation of CASP3 is induced by the initiator CASPs, CASP8 and CASP9, as part of the extrinsic and intrinsic apoptotic signaling pathways. In the extrinsic pathway, death ligands, including Fas ligand, bind and activate their cognate receptors which eventually activate CASP8 [37]. In the results of real-time PCR experiment, the BMCF can be seen to have had no effect on FasR even though Bax and CASP9 increased, and also Bcl-2 decreased (Figure 5). These results suggested that the apoptotic effects of BMCF is not relevant to the extrinsic but intrinsic apoptotic signaling pathway, although the verification of CASP8 activity should be left in a subject for further study.
Actin is one of the major components of the cellular scaffold that is necessary for organizing and maintaining cell shape. β-actin dynamics support a many of processes ranging from cell motility, cell division and cell morphogenesis to intracellular progress [38]. In this study, mtDNA copy number has been related to β-actin with similar expression patterns (Figure 4, Figure 5F), which suggested that alteration in the process of mitochondrial biogenesis and oxidative respiration, which is induced by the BMCF, demand sufficient β-actin.
Mitophagy is the selective engulfment of mitochondria by autophagosomes and their subsequent catabolism by lysosomes [35]. It was initially found that mitochondria are selectively engulfed by autophagosomes following a loss in membrane potential [39], suggesting that mitophagy mediates selective removal of damaged mitochondria. In this study, not a few experimental evidences suggested the mitophagy phenomenon. However, the verification of mitochondrial morphology and membrane potential affected by the BMCF is remained.
In summary, the results suggest that the BMCF provided by VENTEX activates PINK1-mediated mitochondrial quality control pathway and mitochondrial biogenesis, which consequently increases the efficiency of oxidative respiration in skeletal muscle. On the other hand, in early stage of apoptosis induced by the BMCF is not related to extrinsic death-receptor mediated but mitochondria-mediated signaling pathway.
ACKNOWLEDGEMENTS
This research was supported by the Department of Convergence Medicine and Pharmaceutical Biosciences Research Scholarship Grants, Chung-Ang University in 2013.
References
1. Ibrahim NA, Eid BM, Khalil HM. Cellulosic/wool pigment prints with remarkable antibacterial functionalities. Carbohydr Polym. 2015; 115:559–567. PMID: 25439932.


2. Fluhr JW, Breternitz M, Kowatzki D, Bauer A, Bossert J, Elsner P, Hipler UC. Silver-loaded seaweed-based cellulosic fiber improves epidermal skin physiology in atopic dermatitis: safety assessment, mode of action and controlled, randomized single-blinded exploratory in vivo study. Exp Dermatol. 2010; 19:e9–e15. PMID: 19645851.


3. Koller DY, Halmerbauer G, Böck A, Engstler G. Action of a silk fabric treated with AEGIS in children with atopic dermatitis: a 3-month trial. Pediatr Allergy Immunol. 2007; 18:335–338. PMID: 17346297.
4. Ricci G, Patrizi A, Bendandi B, Menna G, Varotti E, Masi M. Clinical effectiveness of a silk fabric in the treatment of atopic dermatitis. Br J Dermatol. 2004; 150:127–131. PMID: 14746626.


5. Lee MS, Song J, Kim HJ, Park KW, Moon SR. Effect of multi-functional fabric on sleep stages and growth hormone levels during sleep. Int J Neurosci. 2004; 114:795–804. PMID: 15204045.


6. Lee MS, Kim HJ, Song J, Park KW, Moon SR. Effects of multifunctional fabrics on cardiac autonomic tone and psychological state. Int J Neurosci. 2004; 114:923–931. PMID: 15527199.
7. Herbst A, Johnson CJ, Hynes K, McKenzie D, Aiken JM. Mitochondrial biogenesis drives a vicious cycle of metabolic insufficiency and mitochondrial DNA deletion mutation accumulation in aged rat skeletal muscle fibers. PLoS One. 2013; 8:e59006. PMID: 23516592.


8. Wagatsuma A, Kotake N, Yamada S. Muscle regeneration occurs to coincide with mitochondrial biogenesis. Mol Cell Biochem. 2011; 349:139–147. PMID: 21110070.


9. Remels AH, Langen RC, Schrauwen P, Schaart G, Schols AM, Gosker HR. Regulation of mitochondrial biogenesis during myogenesis. Mol Cell Endocrinol. 2010; 315:113–120. PMID: 19804813.


10. Duguez S, Féasson L, Denis C, Freyssenet D. Mitochondrial biogenesis during skeletal muscle regeneration. Am J Physiol Endocrinol Metab. 2002; 282:E802–E809. PMID: 11882500.
11. Rochard P, Rodier A, Casas F, Cassar-Malek I, Marchal-Victorion S, Daury L, Wrutniak C, Cabello G. Mitochondrial activity is involved in the regulation of myoblast differentiation through myogenin expression and activity of myogenic factors. J Biol Chem. 2000; 275:2733–2744. PMID: 10644737.


12. Barbieri E, Battistelli M, Casadei L, Vallorani L, Piccoli G, Guescini M, Gioacchini AM, Polidori E, Zeppa S, Ceccaroli P, Stocchi L, Stocchi V, Falcieri E. Morphofunctional and biochemical approaches for studying mitochondrial changes during myoblasts differentiation. J Aging Res. 2011; 2011:845379. PMID: 21629710.


13. Seyer P, Grandemange S, Rochard P, Busson M, Pessemesse L, Casas F, Cabello G, Wrutniak-Cabello C. P43-dependent mitochondrial activity regulates myoblast differentiation and slow myosin isoform expression by control of Calcineurin expression. Exp Cell Res. 2011; 317:2059–2071. PMID: 21664352.


14. McBride HM, Neuspiel M, Wasiak S. Mitochondria: more than just a powerhouse. Curr Biol. 2006; 16:R551–R560. PMID: 16860735.


15. McFarland R, Taylor RW, Turnbull DM. Mitochondrial disease--its impact, etiology, and pathology. Curr Top Dev Biol. 2007; 77:113–155. PMID: 17222702.


16. Giorgi C, Wieckowski MR, Pandolfi PP, Pinton P. Mitochondria associated membranes (MAMs) as critical hubs for apoptosis. Commun Integr Biol. 2011; 4:334–335. PMID: 21980573.


17. Crompton M. The mitochondrial permeability transition pore and its role in cell death. Biochem J. 1999; 341:233–249. PMID: 10393078.


18. Kroemer G, Mariño G, Levine B. Autophagy and the integrated stress response. Mol Cell. 2010; 40:280–293. PMID: 20965422.


19. Madeo F, Tavernarakis N, Kroemer G. Can autophagy promote longevity? Nat Cell Biol. 2010; 12:842–846. PMID: 20811357.


20. Terman A, Kurz T, Navratil M, Arriaga EA, Brunk UT. Mitochondrial turnover and aging of long-lived postmitotic cells: the mitochondrial-lysosomal axis theory of aging. Antioxid Redox Signal. 2010; 12:503–535. PMID: 19650712.


21. Hood DA, Irrcher I, Ljubicic V, Joseph AM. Coordination of metabolic plasticity in skeletal muscle. J Exp Biol. 2006; 209:2265–2275. PMID: 16731803.


22. Hock MB, Kralli A. Transcriptional control of mitochondrial biogenesis and function. Annu Rev Physiol. 2009; 71:177–203. PMID: 19575678.


23. Kim I, Rodriguez-Enriquez S, Lemasters JJ. Selective degradation of mitochondria by mitophagy. Arch Biochem Biophys. 2007; 462:245–253. PMID: 17475204.


24. Frezza C, Cipolat S, Scorrano L. Organelle isolation: functional mitochondria from mouse liver, muscle and cultured fibroblasts. Nat Protoc. 2007; 2:287–295. PMID: 17406588.
25. Guo W, Jiang L, Bhasin S, Khan SM, Swerdlow RH. DNA extraction procedures meaningfully influence qPCR-based mtDNA copy number determination. Mitochondrion. 2009; 9:261–265. PMID: 19324101.


26. Korohoda W, Pietrzkowski Z, Reiss K. Chloramphenicol, an inhibitor of mitochondrial protein synthesis, inhibits myoblast fusion and myotube differentiation. Folia Histochem Cytobiol. 1993; 31:9–13. PMID: 8500631.
27. Herzberg NH, Middelkoop E, Adorf M, Dekker HL, Van Galen MJ, Van den Berg M, Bolhuis PA, Van den Bogert C. Mitochondria in cultured human muscle cells depleted of mitochondrial DNA. Eur J Cell Biol. 1993; 61:400–408. PMID: 8223726.
28. Hamai N, Nakamura M, Asano A. Inhibition of mitochondrial protein synthesis impaired C2C12 myoblast differentiation. Cell Struct Funct. 1997; 22:421–431. PMID: 9368716.


29. Reed JC. Bcl-2 and the regulation of programmed cell death. J Cell Biol. 1994; 124:1–6. PMID: 8294493.


30. Parker JE, Mufti GJ, Rasool F, Mijovic A, Devereux S, Pagliuca A. The role of apoptosis, proliferation, and the Bcl-2-related proteins in the myelodysplastic syndromes and acute myeloid leukemia secondary to MDS. Blood. 2000; 96:3932–3938. PMID: 11090080.


31. Zhang XD, Wang Y, Wu JC, Lin F, Han R, Han F, Fukunaga K, Qin ZH. Down-regulation of Bcl-2 enhances autophagy activation and cell death induced by mitochondrial dysfunction in rat striatum. J Neurosci Res. 2009; 87:3600–3610. PMID: 19565656.


32. Handschin C, Spiegelman BM. Peroxisome proliferator-activated receptor gamma coactivator 1 coactivators, energy homeostasis, and metabolism. Endocr Rev. 2006; 27:728–735. PMID: 17018837.
33. Kelly DP, Scarpulla RC. Transcriptional regulatory circuits controlling mitochondrial biogenesis and function. Genes Dev. 2004; 18:357–368. PMID: 15004004.


34. Wu Z, Puigserver P, Andersson U, Zhang C, Adelmant G, Mootha V, Troy A, Cinti S, Lowell B, Scarpulla RC, Spiegelman BM. Mechanisms controlling mitochondrial biogenesis and respiration through the thermogenic coactivator PGC-1. Cell. 1999; 98:115–124. PMID: 10412986.


35. Jin SM, Youle RJ. PINK1- and Parkin-mediated mitophagy at a glance. J Cell Sci. 2012; 125:795–799. PMID: 22448035.


36. Matsuda N, Sato S, Shiba K, Okatsu K, Saisho K, Gautier CA, Sou YS, Saiki S, Kawajiri S, Sato F, Kimura M, Komatsu M, Hattori N, Tanaka K. PINK1 stabilized by mitochondrial depolarization recruits Parkin to damaged mitochondria and activates latent Parkin for mitophagy. J Cell Biol. 2010; 189:211–221. PMID: 20404107.


37. Clarke P, Tyler KL. Apoptosis in animal models of virus-induced disease. Nat Rev Microbiol. 2009; 7:144–155. PMID: 19148180.


38. Cingolani LA, Goda Y. Actin in action: the interplay between the actin cytoskeleton and synaptic efficacy. Nat Rev Neurosci. 2008; 9:344–356. PMID: 18425089.


39. Lemasters JJ, Nieminen AL, Qian T, Trost LC, Elmore SP, Nishimura Y, Crowe RA, Cascio WE, Bradham CA, Brenner DA, Herman B. The mitochondrial permeability transition in cell death: a common mechanism in necrosis, apoptosis and autophagy. Biochim Biophys Acta. 1998; 1366:177–196. PMID: 9714796.


Fig. 1
Effect of BMCF on the expression of oxygen consumption. V-Con, 0% BMCF group; V-10, 10% BMCF group; V-30, 30% BMCF group. **p<0.01, ***p<0.001.
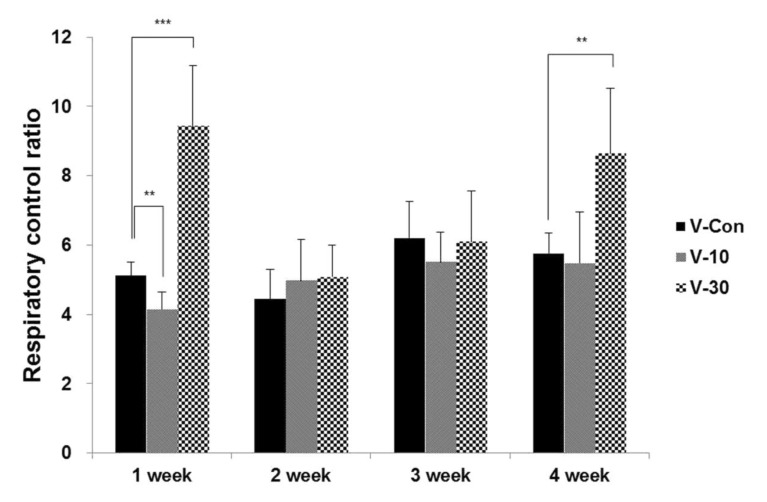
Fig. 2
Effect of BMCF on the expression of mitochondrial DNA copy number. V-Con, 0% BMCF group; V-10, 10% BMCF group; V-30, 30% BMCF group. *p<0.05, **p<0.01.
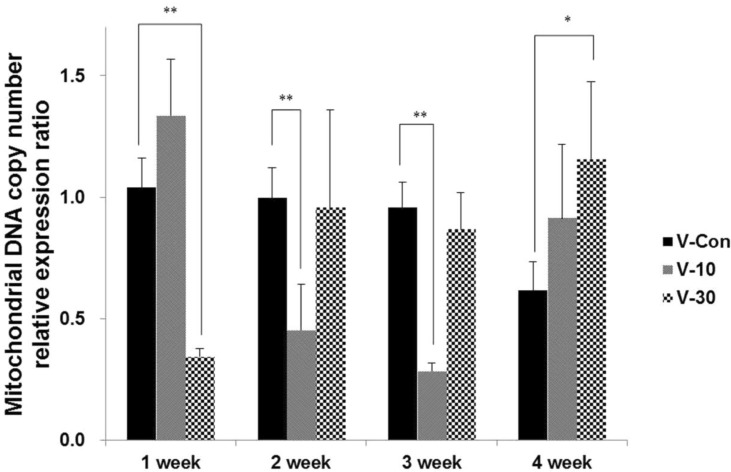
Fig. 3
Effect of BMCF on the expression of oxygen consumption to mitochondrial DNA copy number through 4 weeks. V-Con, 0% BMCF group (n=26); V-10, 10% BMCF (n=19); V-30, 30% BMCF group (n=27). ***p<0.001.
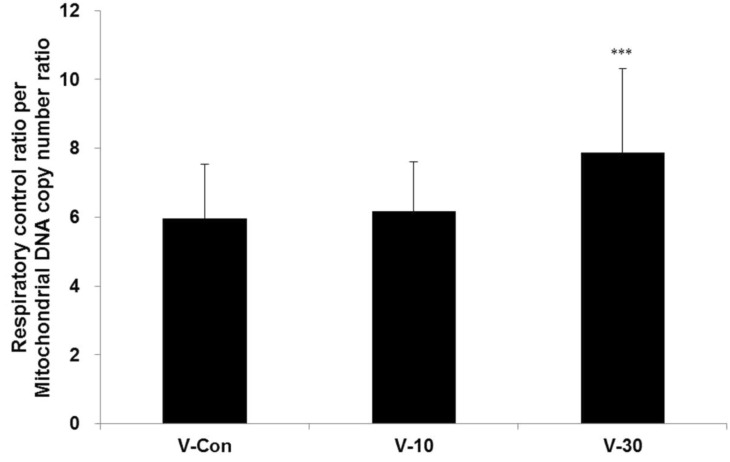
Fig. 4
PCR amplification of the cDNA with B-cell lymphoma 2 gene (Bcl-2), Bcl-2-associated X protein (Bax), PTEN-induced putative kinase 1 (PINK1), Peroxisome proliferator-activated receptor gamma coactivator 1 alpha (PGC-1α), beta-actin (β-actin) and 18S ribosomal RNA (18S) from rat subtrapezial muscle.
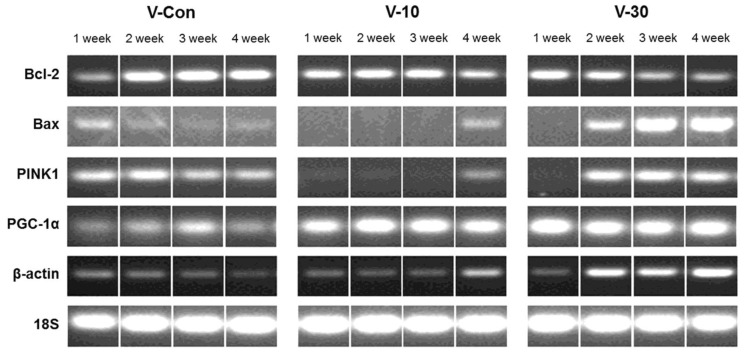
Fig. 5
Effect of BMCF on the mRNA expressions of caspase 9 (CASP9), caspase 3 (CASP3), Bcl-2-associated X protein (Bax), B-cell lymphoma 2 gene (Bcl-2), Fas receptor (FasR) and beta-actin (β-actin). (A) CASP9, (B) CASP3, (C) Bax, (D) Bcl-2, (E) FasR, (F) β-actin. *p<0.05, **p<0.01, ***p<0.001.
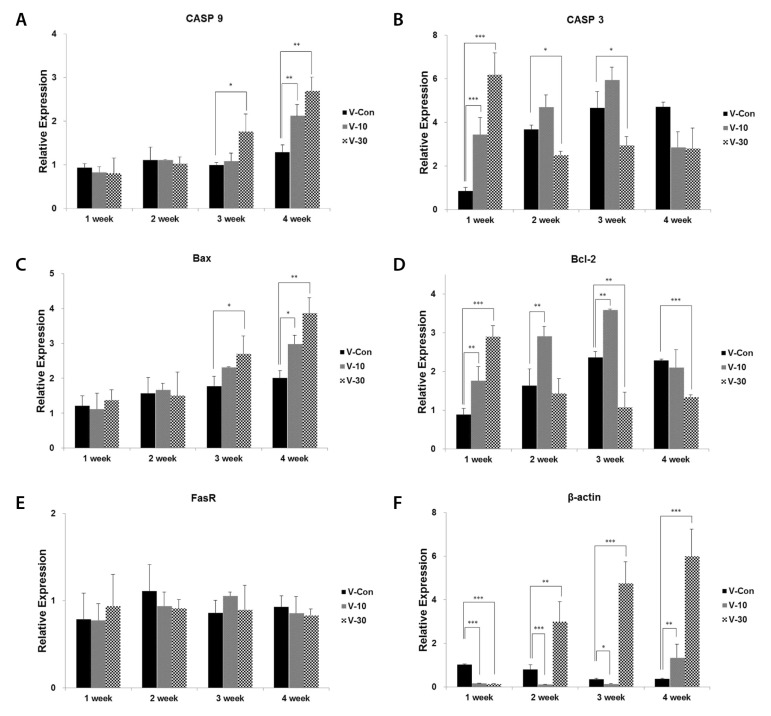
Table 1
Primers used for amplification
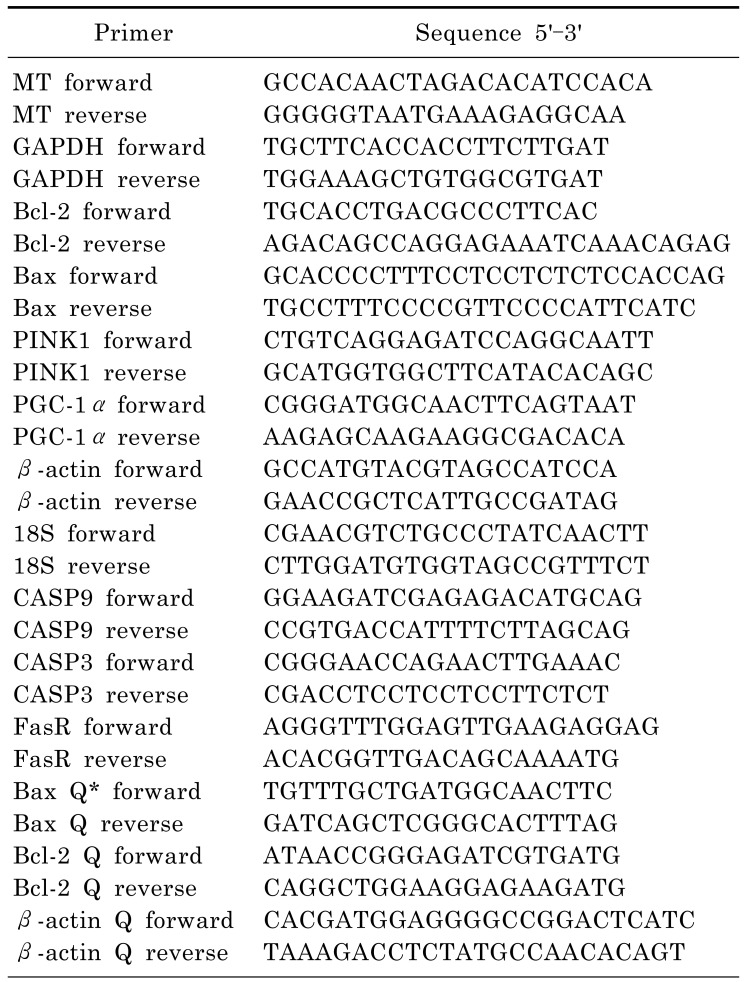
MT, mitochondrial genome; GAPDH, Glyceraldehyde-3-phosphate dehydrogenase; Bcl-2, B-cell lymphoma 2 gene; Bax, Bcl-2-associated X protein; PINK 1, PTEN-induced putative kinase 1; PGC-1α, Peroxisome proliferator-activated receptor gamma coactivator 1 alpha; β-actin, beta-actin; 18S, 18S ribosomal RNA; CASP3, caspase 3; CASP9, caspase 9; FasR, Fas receptor.
*Q, mean primer for real-time PCR.