Abstract
The subfornical organ (SFO) is one of circumventricular organs characterized by the lack of a normal blood brain barrier. The SFO neurons are exposed to circulating glutamate (60~100 µM), which may cause excitotoxicity in the central nervous system. However, it remains unclear how SFO neurons are protected from excitotoxicity caused by circulating glutamate. In this study, we compared the glutamate-induced whole cell currents in SFO neurons to those in hippocampal CA1 neurons using the patch clamp technique in brain slice. Glutamate (100 µM) induced an inward current in both SFO and hippocampal CA1 neurons. The density of glutamate-induced current in SFO neurons was significantly smaller than that in hippocampal CA1 neurons (0.55 vs. 2.07 pA/pF, p<0.05). To further identify the subtype of the glutamate receptors involved, the whole cell currents induced by selective agonists were then compared. The current densities induced by AMPA (0.45 pA/pF) and kainate (0.83 pA/pF), non-NMDA glutamate receptor agonists in SFO neurons were also smaller than those in hippocampal CA1 neurons (2.44 pA/pF for AMPA, p<0.05; 2.34 pA/pF for kainate, p< 0.05). However, the current density by NMDA in SFO neurons was not significantly different from that of hippocampal CA1 neurons (1.58 vs. 1.47 pA/pF, p>0.05). These results demonstrate that glutamate-mediated action through non-NMDA glutamate receptors in SFO neurons is smaller than that of hippocampal CA1 neurons, suggesting a possible protection mechanism from excitotoxicity by circulating glutamate in SFO neurons.
The subfornical organ (SFO), located in the anterior dorsal wall of the third ventricle, is one of the sensory circumventricular organs characterized by the presence of neurons and the lack of a normal blood brain barrier [1,2]. The neurons in the SFO may function as a sensory organ for the detection of the blood borne substances such as angiotensin II and leptin, and send the signals to other brain regions [3,4,5].
Neurons in SFO were also directly accessed by blood borne glutamate (60~100 µM) [6,7,8]. Previous studies showed that glutamate has an excitatory effect on SFO neurons [9,10,11,12] and its excitatory effect was mediated by the activation of non-NMDA receptors [13]. Glutamate also modulates excitatory or inhibitory synaptic currents on SFO neurons and the excitability of SFO neurons [11,14]. It is also known that subcutaneous injection of glutamate can induce chemical lesion in the circumventricular organs [15]. However, the density of glutamate receptor subtypes in the SFO is largely unknown.
Glutamate is the key excitatory neurotransmitter that causes excitotoxicity in the various insults including brain ischemia. It causes the hypoxic-ischemic neuronal death [16]. Among various brains regions, the hippocampus, which plays an important role in learning and memory, has the highest level of ionotropic glutamate receptors, such as AMPA, kainate and NMDA receptors [17]. The hippocampus, the CA1 area in particular, is selectively vulnerable to brain ischemia [18,19]. Since the SFO neurons are exposed to a level of glutamate (~100 µM) high enough to induce excitotoxicity in the other brain regions, there should be a protection mechanism for SFO neurons from circulating glutamate. However, little is known about how the SFO neurons are protected from a potential excitotoxicity of circulating glutamate. In this study, to figure out the possible protection mechanism for SFO neurons from excitotoxicity induced by circulating glutamate, we compared the glutamate-induced current density in SFO neurons and hippocampal CA1 neurons in brain slice using the patch clamp technique.
All experiments in the present study were carried out according to the protocol (SNU-100824-4) for care and use of animals approved by the Laboratory Animal Care Advisory Committee of Seoul National University. All effort was made to minimize the number of animals used and their suffering.
For recording the currents from SFO and hippocampal CA1 neurons in brain slice, the slice containing the SFO and hippocampus were prepared by the previously reported methods [14,20]. Spraque-Dawley rats (4~6 weeks old) were deeply anesthetized with diethyl ether and decapitated. The brain was quickly dissected out, immersed in cold artificial cerebrospinal fluid (ACSF, 0~4℃) containing (in mM) 126 NaCl, 26 NaHCO3, 5 KCl, 1.2 NaH2PO4, 2.4 CaCl2, 1.2 MgCl2, and 10 glucose and aerated with O2/CO2 (95%/5%). In each rat, coronal slices (200 µm) containing the SFO and hippocampus were cut from a block of the forebrain and then incubated in aerated ACSF at 32℃ for 60~90 min. For ionic current recording, a slice was transferred to the recording chamber (0.7 ml), which was continuously perfused with aerated ACSF (37℃) at the rate of 2.7 ml/min. Whole cell currents were recorded from neurons in all regions of the SFO and CA1 region of hippocampus in the slice [13,14,21]. Pipettes were filled with a solution containing (in mM) 140 KCl, 20 HEPES, 0.5 CaCl2, 5 EGTA, 5 QX-314, and 5 MgATP (pH=7.2) with 3M KOH (about 1 ml). Pipette tip resistance range was 2~5 MΩ. Individual neuron in the slice was identified using an upright microscope equipped with Nomarski optics (X400, BX50WI, Olympus, Tokyo, Japan) as reported previously [13,14,21]. Whole cell currents were measured by using a patch clamp amplifier (Axopatch 200B, Axon Instruments, USA). Current signals were filtered at 2 kHz and digitized directly into a PC at 1 kHz with the use of pClamp (Ver 7.0) and Digidata 1200A (Axon Instruments, USA) for subsequent analysis and display. The membrane potentials were held at -70 mV. The current density was obtained by dividing the whole cell current by membrane input capacitance, which was calculated from the membrane time constant. The drugs used in this study were purchased from Sigma (MO, USA).
Mean membrane capacitances of hippocampal CA1 neurons (121±13.2 pF, n=6) were larger than SFO neurons (39.1±4.09 pF, n=10, p<0.05), indicating that hippocampal CA1 neurons are larger than SFO neurons in their cell surface area. Bath application of glutamate (100 µM) induced inward currents in both SFO and hippocampal CA1 neurons (Fig. 1A). As shown in Fig. 1B, the amplitude of inward current at its peak in SFO neurons was much lower than that in hippocampal CA1 neurons (20.6±6.39 pA, n=10 vs. 289±146 pA, n=6, p<0.05). When the currents were normalized by the mean membrane capacitance, the mean current density of SFO neurons (0.55±0.15 pA/pF) was much smaller than that in hippocampal CA1 neurons (2.07±0.79 pA/pF, p<0.05, Fig. 1C). These results indicate that the maximal response of glutamate in SFO neurons is less than that in hippocampal CA1 neurons in slice preparation.
To further determine the subtype of glutamate receptors mediating the glutamate-induced inward currents, we compared the whole cell currents induced by non-NMDA receptor agonists (AMPA and kainate) in SFO and hippocampal CA1 neurons. Bath application of AMPA and kainate (10, 30, and 100 µM) induced a reversible and concentration-dependent inward current as reported previously [1]. In this study, we compared the current response at the threshold concentration (10 µM) that induced an inward current in all tested cells. As shown in Fig. 2A, AMPA and kainite induced inward currents both in SFO and hippocampal CA1 neurons. The inward currents induced by AMPA (10 µM) and kainate (10 µM) in SFO neurons were significantly smaller than those in hippocampal CA1 neurons (17.3±3.79 pA, n=13 vs. 208±24.4 pA, n=11, p<0.05 for AMPA; and 24.9±3.44 pA, n=11, vs. 202±44.1 pA, n=7, p<0.05 for kainate), respectively (Fig. 2B). When we normalized the AMPA- and kainite-induced currents by the capacitance of each neuron, we found that the densities of AMPA- and kainite-induced currents in SFO neurons were also significantly smaller than those in hippocampal CA1 neurons (0.45±0.10 vs. 2.44±0.28 pA/pF, p<0.05; 0.83±0.20 vs. 2.34±0.32 pA/pF, p<0.05), respectively (Fig. 2C). The current density ratios of SFO neurons to hippocampal CA1 neurons were 0.18 and 0.35 for AMPA and kainite, respectively.
For comparison of the inward current induced by glutamate through the activation of NMDA receptors, we recorded NMDA (10 µM)-induced currents in the ACSF without Mg2+, which is known to block NMDA current [22] as illustrated in Fig. 3. The mean inward currents by NMDA from SFO and hippocampal CA1 neurons were 56.9±9.02 pA (n=4) and 154±63.2 pA (n=4), respectively (Fig. 3B). As shown in Fig. 3C, the normalized mean current densities were not significantly different (1.58±0.31 [n=4] vs. 1.47±0.48 pA/pF [n=4]).
This study is the first to demonstrate a protection mechanism of SFO neurons from possible excitotoxicity of circulating glutamate. The density of glutamate-induced current in SFO neurons is 4 times lower than that in hippocampal neurons, and this difference is mostly due to the smaller responses mediated by non-NMDA receptor subtypes.
It is well known that glutamate-induced neurotoxicity is caused by an intracellular accumulation of Ca2+ via mainly AMPA and NMDA receptors [16,17,23]. Kainate can also induce neurotoxicity in various brain sites [24,25]. AMPA and kainate receptors trigger a rapid excitatory neurotransmission by promoting the influx of Na+ into neurons. NMDA receptors are associated to a high conductance with calcium channel which is blocked by Mg2+ in non-depolarizing condition in a voltage dependent manner. Their activation is secondary to AMPA or kainate receptor activation, which depolarizes the neuron, allowing for the release of the Mg2+ blockade. Therefore, AMPA and kainite receptors play a major role in the initiation of the excitotoxicity process in neurons [26]. In this study, we also found that non-NMDA receptor responses are selectively lower in the SFO neurons than in the hippocampal neurons. Since the SFO neuronal cell bodies are located out of blood brain barrier, circulating glutamate can freely access the SFO neurons and its concentration at the SFO is likely to be sufficiently high to induce excitotoxicity reported in general central neurons, such as hippocampal neurons. However, SFO neurons in adult rat normally do not suffer from excitotoxicity and are sensitive to exogenously applied glutamate [27,28,29]. Therefore, it is likely that circulating glutamate causes much smaller inward current in SFO neurons and the threshold of the excitotoxicity of glutamate is much higher in SFO neurons than in the other central neurons.
In the experiments comparing the densities of ionotropic glutamate receptor agonist-induced currents, the current density ratios of SFO neurons to hippocampal CA1 neurons were 0.18 for AMPA and 0.35 for kainate, respectively. However, there was no significant difference in current density by NMDA between SFO neurons and hippocampal CA1 neurons. These data indicate that the lower density of glutamate-induced current in SFO neurons compared to hippocampal CA1 neurons is mostly due to a lower glutamate current induced by non-NMDA glutamate receptors. Our observation is also in agreement with the previous report of Xu et al. [13] showing that the non-NMDA receptor agonist, kainic acid is more potent than NMDA in depolarizing SFO neurons. Here, one can infer that the lower density of non-NMDA receptors in the SFO neurons results in less depolarization, as well as a failure or delay in releasing Mg2+ block, whereas the higher density of non-NMDA receptors in the hippocampal CA1 neurons causes more depolarization, and an effective removal of Mg2+ block of NMDA receptors. Therefore, the protection mechanism in SFO neurons is, at least in part, due to the higher threshold for the initiation of excitotoxicity by glutamate.
In SFO, there may be other protection mechanisms such as the effective removal of glutamate in the vicinity of SFO neurons by highly efficient glutamate transporters in the SFO [6,30]. The relative significance among different protection mechanisms remains to be studied further. Since the density of glutamate subtypes was functionally assessed in this study, it would be necessary to perform a direct comparison of the densities of glutamate receptor subtypes between hippocampal CA1 and SFO neurons. In addition, the current densities of NMDA receptors recorded in ACSF without Mg2+ were not significantly different between the two neuron groups (Fig. 3). Direct measurement of NMDA receptors expressed on two neuron groups is also needed.
Taken together, this study provides a new insight that the current density of non-NMDA glutamate receptors on SFO neurons is lower than other central neurons, such as CA1 neurons, that are selectively vulnerable to ischemic excitotoxicity, and suggest that lower current density of non-NMDA glutamate receptors could be an effective protection mechanism for the SFO neurons from excitotoxicity of circulating glutamate.
ACKNOWLEDGEMENTS
Authors thank Chae Jeong Lim for his technical assistance. This work was supported by a grant (2010-0021656) in the General Researcher Program of NRF funded by the Korean Government (MEST).
References
1. Dellmann HD. Structure of the subfornical organ: a review. Microsc Res Tech. 1998; 41:85–97. PMID: 9579597.


2. Johnson AK, Gross PM. Sensory circumventricular organs and brain homeostatic pathways. FASEB J. 1993; 7:678–686. PMID: 8500693.


3. Ferguson AV, Bains JS. Actions of angiotensin in the subfornical organ and area postrema: implications for long term control of autonomic output. Clin Exp Pharmacol Physiol. 1997; 24:96–101. PMID: 9043813.


4. McKinley MJ, Allen AM, Burns P, Colvill LM, Oldfield BJ. Interaction of circulating hormones with the brain: the roles of the subfornical organ and the organum vasculosum of the lamina terminalis. Clin Exp Pharmacol Physiol Suppl. 1998; 25:S61–S67. PMID: 9809195.


5. Smith PM, Chambers AP, Price CJ, Ho W, Hopf C, Sharkey KA, Ferguson AV. The subfornical organ: a central nervous system site for actions of circulating leptin. Am J Physiol Regul Integr Comp Physiol. 2009; 296:R512–R520. PMID: 19020290.


6. Price MT, Olney JW, Lowry OH, Buchsbaum S. Uptake of exogenous glutamate and aspartate by circumventricular organs but not other regions of brain. J Neurochem. 1981; 36:1774–1780. PMID: 6113269.


7. Fontana L, Moreira E, Torres MI, Fernández MI, Ríos A, Sánchez de Medina F, Gil A. Serum amino acid changes in rats with thioacetamide-induced liver cirrhosis. Toxicology. 1996; 106:197–206. PMID: 8571392.


8. Hawkins RA, DeJoseph MR, Hawkins PA. Regional brain glutamate transport in rats at normal and raised concentrations of circulating glutamate. Cell Tissue Res. 1995; 281:207–214. PMID: 7648616.


9. Gutman MB, Ciriello J, Mogenson GJ. Effect of paraventricular nucleus lesions on cardiovascular responses elicited by stimulation of the subfornical organ in the rat. Can J Physiol Pharmacol. 1985; 63:816–824. PMID: 2864128.


10. Osaka T, Yamashita H, Koizumi K. Inhibitory inputs to the subfornical organ from the AV3V: involvement of GABA. Brain Res Bull. 1992; 29:581–587. PMID: 1422855.


11. Schmid HA. Effect of glutamate and angiotensin II on whole cell currents and release of nitric oxide in the rat subfornical organ. Amino Acids. 1998; 14:113–119. PMID: 9871450.


12. Weindl A, Bufler J, Winkler B, Arzberger T, Hatt H. Neurotransmitters and receptors in the subfornical organ. Immunohistochemical and electrophysiological evidence. Prog Brain Res. 1992; 91:261–269. PMID: 1329146.
13. Xu SH, Inenaga K, Honda E, Yamashita H. Glutamatergic synaptic inputs activate neurons in the subfornical organ through non-NMDA receptors. J Auton Nerv Syst. 2000; 78:177–180. PMID: 10789698.


14. Lee HS, Chong W, Han SK, Lee MH, Ryu PD. Activation of metabotropic glutamate receptors inhibits GABAergic transmission in the rat subfornical organ. Neuroscience. 2001; 102:401–411. PMID: 11166126.


15. Corrêa FM, Saavedra JM. Chemical lesion of the circumventricular organs with monosodium glutamate reduces the blood pressure of spontaneously hypertensive but not of one kidney-one clip hypertensive rats. Braz J Med Biol Res. 1992; 25:515–519. PMID: 1342228.
16. Choi DW, Rothman SM. The role of glutamate neurotoxicity in hypoxic-ischemic neuronal death. Annu Rev Neurosci. 1990; 13:171–182. PMID: 1970230.


17. Ozawa S, Kamiya H, Tsuzuki K. Glutamate receptors in the mammalian central nervous system. Prog Neurobiol. 1998; 54:581–618. PMID: 9550192.


18. Harry GJ, Lefebvre d'Hellencourt C. Dentate gyrus: alterations that occur with hippocampal injury. Neurotoxicology. 2003; 24:343–356. PMID: 12782100.


19. Schmidt-Kastner R, Freund TF. Selective vulnerability of the hippocampus in brain ischemia. Neuroscience. 1991; 40:599–636. PMID: 1676492.


20. Inenaga K, Nagatomo T, Honda E, Ueta Y, Yamashita H. GABAergic inhibitory inputs to subfornical organ neurons in rat slice preparations. Brain Res. 1995; 705:85–90. PMID: 8821737.


21. Ferguson AV, Li Z. Whole cell patch recordings from forebrain slices demonstrate angiotensin II inhibits potassium currents in subfornical organ neurons. Regul Pept. 1996; 66:55–58. PMID: 8899894.


22. Bekkers JM, Stevens CF. NMDA receptors at excitatory synapses in the hippocampus: test of a theory of magnesium block. Neurosci Lett. 1993; 156:73–77. PMID: 8414193.


23. Takasaki Y. Studies on brain lesions after administration of monosodium L-glutamate to mice. II. Absence of brain damage following administration of monosodium L-glutamate in the diet. Toxicology. 1978; 9:307–318. PMID: 663940.


24. Simonian NA, Getz RL, Leveque JC, Konradi C, Coyle JT. Kainic acid induces apoptosis in neurons. Neuroscience. 1996; 75:1047–1055. PMID: 8938740.


25. Portera-Cailliau C, Price DL, Martin LJ. Non-NMDA and NMDA receptor-mediated excitotoxic neuronal deaths in adult brain are morphologically distinct: further evidence for an apoptosis-necrosis continuum. J Comp Neurol. 1997; 378:88–104. PMID: 9120056.


26. Michaelis EK. Molecular biology of glutamate receptors in the central nervous system and their role in excitotoxicity, oxidative stress and aging. Prog Neurobiol. 1998; 54:369–415. PMID: 9522394.


27. Osaka T, Yamashita H, Koizumi K. Inhibition of paraventricular neurons by subfornical organ and AV3V in cats. Am J Physiol. 1988; 255:R961–R967. PMID: 3202228.


28. Osaka T, Yamashita H, Koizumi K. Inhibitory inputs to the subfornical organ from the AV3V: involvement of GABA. Brain Res Bull. 1992; 29:581–587. PMID: 1422855.


29. Wright JW, Roberts KA, Stubley LA, Hanesworth JM, Harding JW. Hypothalamic angiotensin release in response to AII or glutamic acid stimulation of the SFO in rats. Brain Res Bull. 1993; 31:649–654. PMID: 8100178.
30. Berger UV, Hediger MA. Distribution of the glutamate transporters GLAST and GLT-1 in rat circumventricular organs, meninges, and dorsal root ganglia. J Comp Neurol. 2000; 421:385–399. PMID: 10813794.


Fig. 1
Comparison of glutamate-induced inward currents recorded by the slice patch clamp technique in SFO and hippocampal CA1 neurons. (A) Representative traces showing glutamate-induced inward currents on a SFO (left) and a hippocampal CA1 neuron (right), respectively. (B) Comparison of the mean inward currents by glutamate (100 µM) on SFO (n=10) and hippocampal CA1 neurons (n=6). (C) Comparison of the mean current densities by glutamate (100 µM) on SFO and hippocampal CA1 neurons. *represents p<0.05. SFO, Subfornical organ neuron; HP, Hippocampal CA1 neuron.
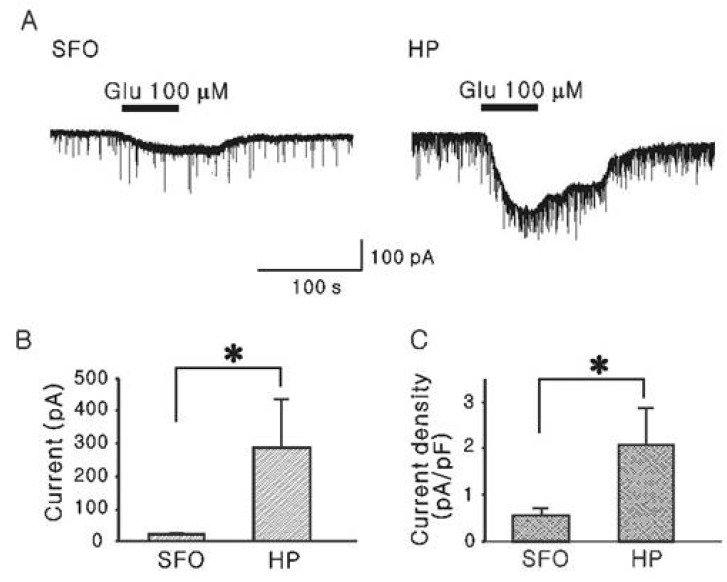
Fig. 2
Current responses elicited by non-NMDA receptor agonists (AMPA and kainate) in SFO and hippocampal CA1 neurons in the slice preparation. (A) Representative traces showing currents induced by AMPA (10 µM) and kainate (10 µM) in SFO (left) and hippocampal CA1 neuron (right), respectively. (B, C) Mean amplitude (B) and mean density (C) of inward currents induced by AMPA and kainate in SFO (n=13 and 11) and hippocampal neurons (n=11 and 7) at the peak *p<0.05. SFO, Subfornical organ neuron; HP, Hippocampal CA1 neuron.
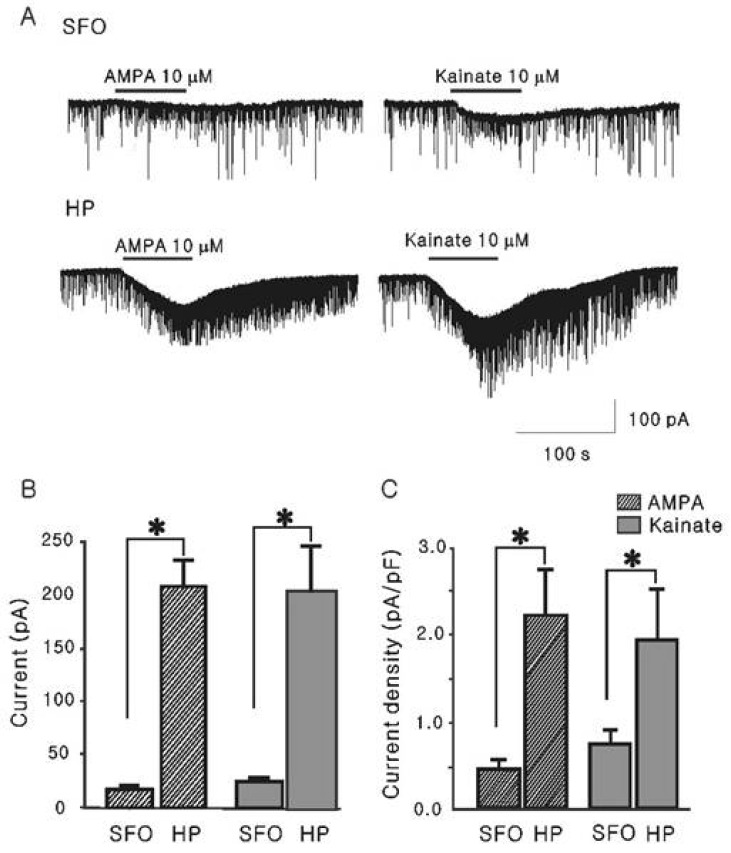
Fig. 3
Current responses elicited by NMDA in SFO and hippocampal neurons. (A) Left and right traces show NMDA (10 µM) induced inward current in SFO and hippocampal CA1 neurons. Mg2+-free ACSF was applied for recording of NMDA induced current. (B, C) Mean inward current induced by NMDA (B) and its mean current density (C) in SFO (n=4) and hippocampalCA1 neurons (n=4). SFO, Subfornical organ neuron; HP, Hippocampal CA1 neuron.
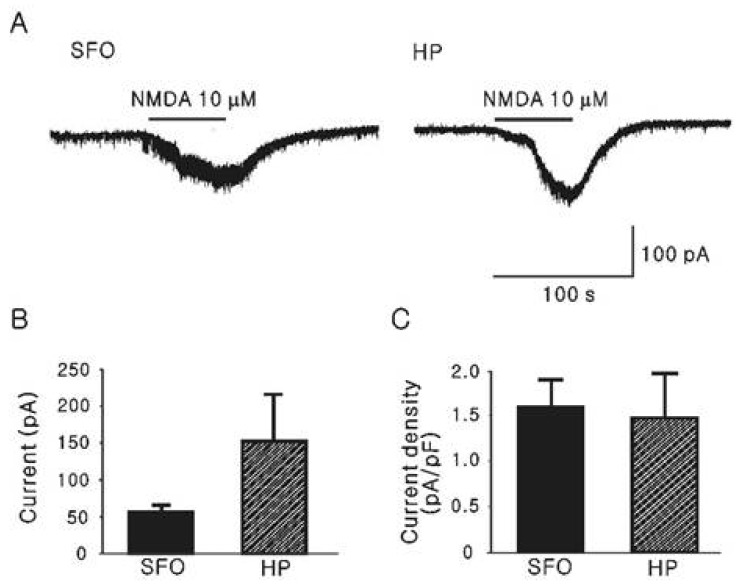