Abstract
Spontaneous myometrial contraction (SMC) in pregnant uterus is greatly related with gestational age and growing in frequency and amplitude toward the end of gestation to initiate labor. But, an accurate mechanism has not been elucidated. In human and rat uterus, all TRPCs except TRPC2 are expressed in pregnant myometrium and among them, TRPC4 are predominant throughout gestation, suggesting a possible role in regulation of SMC. Therefore, we investigated whether the TRP channel may be involved SMC evoked by mechanical stretch in pregnant myometrial strips of rat using isometric tension measurement and patch-clamp technique. In the present results, hypoosmotic cell swelling activated a potent outward rectifying current in G protein-dependent manner in rat pregnant myocyte. The current was significantly potentiated by 1µM lanthanides (a potent TRPC4/5 stimulator) and suppressed by 10µM 2-APB (TRPC4-7 inhibitor). In addition, in isometric tension experiment, SMC which was evoked by passive stretch was greatly potentiated by lanthanide (1µM) and suppressed by 2-APB (10µM), suggesting a possible involvement of TRPC4/5 channel in regulation of SMC in pregnant myometrium. These results provide a possible cellular mechanism for regulation of SMC during pregnancy and provide basic information for developing a new agent for treatment of premature labor.
The frequency and amplitude of spontaneous myometrial contraction (SMC) in pregnant uterus are greatly related with gestational age [1]. Whereas SMC are hardly observed in the early and mid-gestational stage, the SMC increase in frequency and amplitude toward the end of gestation to augment myometrial contractility to initiate labor [2]. Although an accurate mechanisms which trigger labor are not yet clear, stretch of the myometrium mediated by the growth of the fetus, has been reported to play an important role in inducing labor [3,4,5]. In fact, preterm labor more frequently occurs in plural pregnancy or polyhydroamnios [6,7] in which the myometrium is excessively stretched compared to normal pregnancy, indicating a crucial role of myometrial stretch in controlling spontaneous uterine contractility during pregnancy. A detailed mechanism of the stretch-induced SMC during pregnancy has not yet been elucidated yet.
Generally contractions in uterine smooth muscle are usually initiated by membrane depolarization. The depolarization opens voltage-gated Ca2+ channels to trigger action potential (AP) discharges, which causes increase intracellular Ca2+ concentration by Ca2+ influx into uterine myometrial cell through opened voltage-gated Ca2+ channels [8]. So, any stimuli which cause membrane depolarization enough to trigger AP discharges can readily contract myometrium. Therefore, it is possible that SMC occurring during late-term gestation may be caused by depolarization of membrane potential induced by mechanical stretch. However, to date, a mechanism which can explain stretch-induced membrane depolarization in pregnant uterine myometrium has not been clearly established yet.
Recently, transient receptor potential (TRP) channels have come into the spotlight as a candidate for processing these mechanical stimuli. For instance, TRPV2, M3 and M4 are activated by mechanical stimuli evoked by osmotic cell swelling in various tissues [9,10,11,12,13,14,15] and activation of the TRP channels may depolarize the membrane potential through opening of nonselective cationic channel to trigger AP. In human and rat uterus, all TRPCs except TRPC2 are expressed in non-pregnant and pregnant myometrium and among them, TRPC4 are predominant throughout gestation [16,17,18]. These results suggest that TRP channels may be involved in development of SMC by initiating membrane depolarization in pregnant rat uterus. To date, however, a physiological role of these TRP channels in pregnant myometrium has not been clearly determined yet.
Taken all these into consideration, it is possible that TRP channels may play a key role in regulating SMC during late-term gestation. To investigate this hypothesis, we tested 1) whether the TRP channel may be involved SMC evoked by mechanical stretch in pregnant myometrial strips of rat using isometric tension measurement technique. We also confirmed the existence of ion channel activated by hypoosmotic stretch and analyzed the electrophysiological characteristics to elucidate a correlation with TRP channels. We demonstrate here that mechanical stretch induced by hypoosmotic stimuli may activate TRPC4/5 like channel in rat pregnant myometrium, which may contribute to membrane depolarization to trigger SMC in pregnant myometrium.
This study was conducted in accordance with the National Institute of Health (NIH) Guide for the Care and Use of Laboratory Animals. All procedures were performed in accordance with protocols approved by the Institutional Animal Care and Use Committee.
Myometrial strips were prepared from Sprague-Dawley rats in late pregnancy (18~20 days gestation), as described previously. Briefly, we removed the blood from a blood vessel by perfusing the rats transcardially with cold Dulbecco's phosphate buffer saline (D-PBS) for 15 min under enflurane anesthesia (3%, 2~3 min). Longitudinal myometrial strips, approximately 1.5~3 mm from each horn, were dissected out with fine scissors under a binocular microscope. Both ends of the tissue strip were tied with thin threads. One end was connected to a force-displacement transducer to monitor muscle tension and the other end was attached to a tissue holder. The Krebs-Henseleit (KH) solution was maintained at 37℃ and was continuously aerated with 95% O2, 5% CO2. Muscle strips were stretched passively to an optimal length by imposing a stretch of 800 mg and equilibrated for 60 min.
The longitudinal muscle layer of myometrium was cut into small pieces and placed in Ca2+-free PSS. The Ca2+-free PSS was then replaced with PSS containing 30µM Ca2+ (low Ca2+ PSS), and 1 h incubations at 37℃ were carried out in fresh, low-Ca2+ PSS containing collagenase (0.3 mg/ml), papain (0.6 mg/ml), and bovine serum albumin (1 mg/ml). After enzyme digestion, tissue fragments were suspended in a fresh 120µM Ca2+ containing PSS and gently agitated. The resulting suspension was centrifuged at 800 g for 2 min, and the cells were resuspended in a 0.5 mM Ca2+ containing PSS, aliquoted into 12 mm poly-L-lysine-coated cover glasses, and stored in a humidified atmosphere at 4℃. Experiments were carried out within 12 h of harvesting at (22~24℃).
Osmotic stress-induced current was recorded using conventional whole-cell techniques. Electrode resistance varied from 2 to 4 MΩ when cells were filled with internal solution. We performed measurements using an Axopatch 1D patch-clamp amplifier (Molecular Devices, Sunnyvale, CA, USA). Voltage and current commands and digitization of membrane voltages and currents were controlled using a Digidata 1440 A interfaced with Clampex 10.2 (Molecular Devices, Sunnyvale, CA, USA) on an IBM-compatible computer. We analyzed data using Clampfit 10.2 (Molecular Devices, Sunnyvale, CA, USA) and Prism 5.0 (GraphPad, San Diego, CA, USA). Currents were low-pass filtered at 2 kHz using a four-pole Bessel filter in the amplifier. Capacitance (Cm) values were taken from automatically calculated recordings by the pClamp 10.2 software. Multiple independently controlled syringes served as reservoirs for a gravity-driven fast drug perfusion system. Switching between solutions was accomplished by manually controlled valves. All experiments were conducted at room temperature.
The Krebs-Henseleit solution (KH solution) of the following composition as modified by Asano and Nomura [19] (in mM): 119 NaCl, 4.6 KCl, 2.5 CaCl2, 1.2 KH2PO4 1.2, 1.5 MgSO4, 25 NaHCO3, 11 glucose. The KH solution was continuously aerated with 95% O2 and 5% CO2. The Ca2+-free PSS contained the following (in mM): 140 NaCl, 5.4 KCl; 1.2 MgCl2, 10 HEPES, 12 Glucose; the solution was adjusted to pH 7.4 with NaOH. Low Ca2+ PSS was made by adding Ca2+ (30 or 120µM) to Ca2+-free PSS. The internal (pipette) solution contained the following (in mM): 120 CsCl2, 1 MgCl2, 4 MgATP, 0.4 Na2GTP, 10 phosphocreatine, 10 HEPES, and 1 EGTA, 0.363 CaCl2, 80 sucrose; the solution was adjusted to pH 7.2 with CsOH. The external (bath) solution contained (300mOsm) (in mM) 120 CsCl, 0.363 CaCl2, 1 MgCl2, 80 sucrose, 10 HEPES and 1 EGTA; the solution was adjusted to pH 7.4 with CsOH. The hypoosmotic solutions (260 mosm and 220 mosm solutions) were made by removing sucrose from the abovementioned composition and the osmolality of the solution was tested using an osmometer. Through this test, the effects of changes in the concentration of Cl ions in the solution on the junction potential were minimized. The D-PBS solution with glucose contained the following (in mM): 2.67 KCl, 1.47 KH2PO4, 137.93 NaCl, 8.06 Na2HP4~7H2O, 5.56 D-glucose; the solution was adjusted to pH 7.4 with HCl. The phosphate-buffered saline (PBS) contained the following (in mM): 137 NaCl, 2.7 KCl, 10.14 Na2HPO4, 1.76 KH2PO4. All other drugs were purchased from Sigma-Aldrich Chemicals. All other drugs were dissolved in distilled water as stock solutions (1~100 mM).
Data are presented as mean±SEM, with the number of experiments indicated in parentheses. The frequency of SMC was presented as the number of spontaneous contractions per 5 min. The amplitude of SMC was presented in grams (g). We used a paired t-test to compare the effects of lanthanide and 2-APB before and after application. We used unpaired Student's t-tests to compare data from two groups. Differences were considered statistically significant at p<0.05.
To investigate the existence of stretch-induced ion channels in pregnant rat myocyte, we measured whole-cell currents evoked in response to application of hypoosmotic solutions using a voltage clamp technique. As shown in Fig. 1A, application of hypoosmotic solution (260 mOsm, 220 mOsm) evoked a large, reversible current in proportion to the degree of hypoosmolarity. Fig. 1B shows for the same cell, the I~V relation of hypoosmotic swelling-induced current (IHypo) with a characteristic outward rectifying shape and reversal potentials close to 0 mV. IHypos were recorded in rat pregnant myocyte with a mean amplitude (2.9±0.4 pA/pF for 300 mOSm, 8.9±1.7 pA/pF for 260 mOsm, 15.8±2.6 pA/pF for 220 mOsm at -60 mV; 4.9±0.9 pA/pF for 300 mOsm, 12.9±2.5 pA/pF for 260 mOsm, 22.3±2.9 pA/pF for 220 mOsm at +60 mV, n=6) (Fig. 1C).
To rule out the possibility that IHypo is carried by flux of anions like chloride ion, we replaced Cs+ in external solution with NMDG+ to remove monovalent cation. As shown in Supplemental Fig. 2, NMDG+ in the external solution nearly completely prevented current development evoked by application of hypoosmotic NMDG+ solution (0.7±0.7 pA/pF for 300 mOsm, 0.7±0.7 pA/pF for 220 mOsm at -60 mV; 4.9±0.9 pA/pF for 300 mOsm, 1.1±0.8 pA/pF for 260 mOsm, 1.2±0.9 pA/pF for 220 mOsm at +60 mV, n=5) (Supplemental Fig. 2). In addition, replacing Cl- in the pipet solution did not significantly shift reversal potential of IHypo (120 CsCl: 3.6±1.4 mV, 50 CsCl: 3.4±1.3 mV, n=7; p>0.05) (Supplemental Fig. 3). These data suggested the existence of nonselective cation channel evoked by hypoosmotic membrane stimulus in rat pregnant myocyte.
We determined the involvement of G proteins in activation of IHypo using GDPβS, a hydrolysis-resistant GDP analogue known to prevent G protein activation [20]. As shown in Fig. 2B, intracellular dialysis of GDPβS (2 mM) prevented induction of IHypo (5.3±1.0 pA/pF for 300 mOsm, 6.7±1.9 pA/pF for 220 mOsm at -60 mV; 5.3±1.0 pA/pF for 300 mOsm, 5.9±0.9 pA/pF for 220 mOsm, n=5, p>0.05). These results suggest that G-protein may be involved in activation of IHypo in rat pregnant myometrium.
To identify the channel activated by hypoosmotic stimuli in pregnant rat myocyte, we examine the effects of 1µM Lanthanide (Gd3+ or La3+) which is a potent TRPC4/5 activator [21], on IHypo. Surprisingly, IHypo was significantly augmented by 1µM Gd3+ (Fig. 3A) (10.4±2.5 pA/pF for 220 mOsm, Gd3+; 17.8±3.7 pF at -60 mV and 220 OSm; 18.3±2.9 pF, Gd3+; 34.7±5.1 pF at 60 mV n=5). In addition, the augmented whole-cell current by micromolar Gd3+ was nearly completely abolished by application of 10µM 2-APB, a potent TRPC blocker (Gd3+; 17.8±3.7 pF, 2-APB; 3.1±1.1 at -60 mV and Gd3+; 34.7±5.1 pF, 2-APB; 2.7±0.7 at 60 mV, n=5). Similarly, La3+ (1µM) also significantly increased hypoosmotic-evoked whole-cell current in pregnant rat myocyte (220 mOSm; 11.8±1.6 pF, 220 mOSm+La3+; 25.6±3.9 pA/pF at -60 mV and 220 mOSm; 13.6±1.8 pF, La3+; 29.5±4.0 pF at 60 mV, n=5) (Fig. 3B). Moreover, the augmented hypooamotic swelling induced-current by La3+ were almost completely suppressed by 10µM 2-APB (La3+; 25.6±3.9 pA/pF, La3++2-APB; 2.8±1.1 at -60 mV and La3+; 29.5±4.0 pA/pF, La3++2-APB; 2.2±0.6 at 60 mV) (Fig. 3B). These results suggest that TRPC4/5 like channels may be involved in regulating hypoosmotic-evoked whole-cell current in pregnant rat myocyte.
To determine whether TRPC channels are involved in spontaneous myometrial contractility in pregnant rat uterus, we examine the effects of micromolar lanthanide on SMC in pregnant myometrial strips. As shown in Fig. 4A, stretch of rat myometrial strip, evoking tension to about 1 g, readily evoked SMC with frequency of 2.9±0.4 Hz and amplitude of 1.6±0.4 g (n=5). Theses SMC were significantly augmented by Gd3+ (1µM) (Gd3+ application: 4.2±0.6 for frequency, 1.7±0.4 g for amplitude, n=5; p<0.05). In addition, the Gd3+-induced augmentation of SMC was nearly completely abolished by application of 10µM 2-APB (0.8±0.4 for frequency, 0.8±0.5 g for amplitude, n=6). Similarly, La3+ (1µM) also significantly increased stretch-evoked SMC in pregnant myometrial strips (Control: 2.4±0.5 for frequency, 1.0±0.5 for amplitude, n=5; La3+ application: 3.6±0.7 for frequency, 1.1±0.5 g for amplitude, n=5; p<0.05) (Fig. 4). The La3+-induced augmentation of SMC were almost completely suppressed by 10µM 2-APB (0.2±0.2 for frequency, 0.3±0.1 g for amplitude, n=5) (Fig. 4). To exclude a possible effect of 2-APB on voltage-dependent Ca2+ current which also play a crucial role in regulating SMC in pregnant myometrium, we examined the effect of 2-APB (10µM) on myometrial contraction evoked by 70 mM high-K solution in rat pregnant myometrial strips and voltage-dependent Ca2+ current measured using voltage-clamp technique. As shown in Figure S1A, 2-APB had little effect on high-K solution-evoked myometrial contraction. In addition, 2-APB also did not affect voltage-dependent Ca2+ current in rat pregnant myocyte (Supplemental Fig. 1B), indicating absence of 2-APB effect on voltage-dependent Ca2+ channel in rat pregnant myometrium.
These results suggest that TRPC4/5 like channels may be involved in regulating SMC evoked by stretch in pregnant myometrium.
According to the previous results, membrane potential of pregnant uterine myocyte is spontaneously depolarized by membrane stretch induced by fetus growth in late gestational age and when this depolarization reaches the threshold, action potential (AP) starts to fire to induce contraction [22,23,24], Although several mechanism for the spontaneous depolarization which results in initiation of AP firing have been proposed, an accurate explanation has not been elucidated yet. Recently, since the existence of stretch-activated (SA) channels were first reported in cultured skeletal muscle cells, expression of SA channels has been identified in diverse cells including smooth muscle cells [25,26,27]. The SA channels are usually activated by membrane-stretch stimuli and cation influx through the SA channels depolarizes the membrane potential, which, in turn, activates voltage-dependent Ca2+ channels that can induce changes in cell functions [27]. To date, to identify this SA channel, various TRP channel which is sensitive to various mechanical stresses including the increase in surface tension evoked by osmotic swelling [28,29,30] was proposed for a candidate for the SA channel. For example, TRPV4 was reported for the first time to be activated by osmotic cell swelling [31,32]. TRPV2 is also reported to be activated by mechanical stretch induced by osmotic cell swelling [9,10]. In addition, TRPM4 [12,13,14,15] and a long splice variant of TRPM3 [11] are also activated by hypoosmotic membrane stretch. As for TRPC family, TRPC1 and TRPC6 were reported to be activated by mechanical and hypoosmotic stretch [33,34], which is still in controversy [35,36,37]. As for uterine myometrium, there also exists TRPC family in human and rat pregnant myometrium [17,18,38]. Among them, TRPC4 is expressed in the greatest abundance without alteration in expression during gestation, suggesting that TRPC4 may play a major role in regulation of Ca2+ entry in uterine myocyte to evoke SMC during pregnancy [17,18]. But, a direct evidence for involvement of TRPC family in SMC during pregnancy has not yet been investigated due to absence of specific pharmacological inhibitors to discriminate TRPC subtypes. Traditionally, the lanthanides (La3+ and Gd3+) have been used as blockers of nonselective cationic channels and Ca2+-permeable channels. But, recently the lanthanides at micromolar range have been reported to potentiate mouse, rat and human TRPC4 and mouse TRPC5, which is in contrast with the effect of lanthanides on other TRPCs. In the present study, hypoosmotic cell swelling readily activated outward going rectifier currents (Fig. 1) and the currents were significantly potentiated by 1µM lanthanides (Gd3+, La3+), a potent TRPC4/5 stimulator , and nearly completely suppressed by 2-APB which is a potent TRPC3-6 blocker (Fig. 3). These results strongly suggest the existence of TRPC4/5 like channel which can be activated by mechanical stretch in rat pregnant myometrium. In addition, in isometric tension measurement experiment, 1µM lanthanides significantly augmented SMC in frequency and amplitude and the augmented SMC is nearly completed abolished by 2-APB application, consistent with the patch-clamp data. These results therefore strongly suggest that TRPC4/5 like channel may be involved in regulation of SMC in pregnant myometrium. Meanwhile, the present results could not discriminate a major contribution to SMC between TRPC4 and 5 in pregnant myometrium. According to recent report, TRPC5 is activated by hypoosmotic- and pressure-induced membrane stretch [39,40], suggesting a possibility for involvement of TRPC5 in stretch-induced SMC. But, TRPC5 mRNA is expressed at extremely lower level compared to that of TRPC4 and is even decreased during gestation [17,18] and reduction in TRPC4 expression specifically attenuates G-protein coupled receptor-stimulated increases in intracellular Ca2+ which contribute to SMC in human myocyte. These results suggest TRPC4 may significantly contribute to regulating SMC in pregnancy compared to TRPC5. But, further studies are needed to determine a functional role of TRPC4/5 in regulating SMC in pregnant myometrium.
As for the mechanistic link between membrane stretch (or cell swelling) and G-protein activation, G protein-coupled receptors (GPCRs) have emerged as a candidate for potential mechanosensors which covert mechanical stimuli to biochemical signals. In fact, various GPCRs such as histamine 1, angiotensin II, acetylcholine and vasopressin receptors are reported to be activated by mechanical stress without the involvement of their ligands [37,41]. For example, it has been reported that in cardinal component of the renin-angiotensin regulatory system, mechanical stress can active the AT1R(angiotensin-II type-1 receptor) independently of angiotensin-II [42]. AT1Rs were also identified as mechanosensors participating in the physiological process of myogenic vasoconstriction. This ligand-independent mechanical activation of GPCRs induces conformational change in transmembrane domain of the receptors, which leads to activation of G proteins [43]. Once GPCRs are activated by mechanical stimuli, TRPC channel activation occurs downstream of mechanically induced GPCR activation, playing a role as a mechanosensor [41,44,45]. In the present study, hypoosmotic stress directly activated TRPC4/5 like channels without involvement of any ligand for GPCR and the channel activation was nearly completely prevented in the presence of GDPβS in the pipette solution. These findings indicate that hypoosmotic stimuli-induced GPCR activation plays a major role in activating TRPC4/5 channels in pregnant myometrium.
In conclusion, we have demonstrated the existence of TRPC4/5 like channels which can be activated by mechanical stimuli evoked by hypoosmotic cell swelling and this channel may play a major role in regulating SMC in pregnant myometrium. These results provide a possible mechanism for regulation of SMC during pregnancy and provide important information for developing a new agent for treatment of obstetric disorder like premature labor.
ACKNOWLEDGEMENTS
This research was supported by the Pioneer Research Center Program through the National Research Foundation of Korea funded by the Ministry of Science, ICT & Future Planning (2012-0009525) and a faculty research grant of Yonsei University College of Medicine for 2006 (6-2011-0164).
References
1. Bengtsson B. Factors of importance for regulation of uterine contractile activity. Acta Obstet Gynecol Scand Suppl. 1982; 108:13–16. PMID: 6957124.


2. Olson DM, Mijovic JE, Sadowsky DW. Control of human parturition. Semin Perinatol. 1995; 19:52–63. PMID: 7754411.


3. Wray S. The role of mechanical and hormonal stimuli on uterine involution in the rat. J Physiol. 1982; 328:1–9. PMID: 7131308.


4. Wray S. Uterine contraction and physiological mechanisms of modulation. Am J Physiol. 1993; 264:C1–C18. PMID: 8430759.


5. Kasai Y, Tsutsumi O, Taketani Y, Endo M, Iino M. Stretch-induced enhancement of contractions in uterine smooth muscle of rats. J Physiol. 1995; 486:373–384. PMID: 7473204.


6. Moutquin JM. Classification and heterogeneity of preterm birth. BJOG. 2003; 110(Suppl 20):30–33. PMID: 12763108.


8. Kleinhaus AL, Kao CY. Electrophysiological actions of oxytocin on the rabbit myometrium. J Gen Physiol. 1969; 53:758–780. PMID: 5783010.


9. Muraki K, Iwata Y, Katanosaka Y, Ito T, Ohya S, Shigekawa M, Imaizumi Y. TRPV2 is a component of osmotically sensitive cation channels in murine aortic myocytes. Circ Res. 2003; 93:829–838. PMID: 14512441.


10. Beech DJ, Muraki K, Flemming R. Non-selective cationic channels of smooth muscle and the mammalian homologues of Drosophila TRP. J Physiol. 2004; 559:685–706. PMID: 15272031.
11. Grimm C, Kraft R, Sauerbruch S, Schultz G, Harteneck C. Molecular and functional characterization of the melastatinrelated cation channel TRPM3. J Biol Chem. 2003; 278:21493–21501. PMID: 12672799.


12. Earley S, Waldron BJ, Brayden JE. Critical role for transient receptor potential channel TRPM4 in myogenic constriction of cerebral arteries. Circ Res. 2004; 95:922–929. PMID: 15472118.


13. Kraft R, Harteneck C. The mammalian melastatin-related transient receptor potential cation channels: an overview. Pflugers Arch. 2005; 451:204–211. PMID: 15895246.


14. Dietrich A, Chubanov V, Kalwa H, Rost BR, Gudermann T. Cation channels of the transient receptor potential superfamily: their role in physiological and pathophysiological processes of smooth muscle cells. Pharmacol Ther. 2006; 112:744–760. PMID: 16842858.


15. Inoue R, Jensen LJ, Shi J, Morita H, Nishida M, Honda A, Ito Y. Transient receptor potential channels in cardiovascular function and disease. Circ Res. 2006; 99:119–131. PMID: 16857972.


16. Dalrymple A, Slater DM, Beech D, Poston L, Tribe RM. Molecular identification and localization of Trp homologues, putative calcium channels, in pregnant human uterus. Mol Hum Reprod. 2002; 8:946–951. PMID: 12356946.


17. Babich LG, Ku CY, Young HW, Huang H, Blackburn MR, Sanborn BM. Expression of capacitative calcium TrpC proteins in rat myometrium during pregnancy. Biol Reprod. 2004; 70:919–924. PMID: 14627551.
18. Ku CY, Babich L, Word RA, Zhong M, Ulloa A, Monga M, Sanborn BM. Expression of transient receptor channel proteins in human fundal myometrium in pregnancy. J Soc Gynecol Investig. 2006; 13:217–225.


19. Asano M, Nomura Y. Comparison of inhibitory effects of Y-27632, a Rho kinase inhibitor, in strips of small and large mesenteric arteries from spontaneously hypertensive and normotensive Wistar-Kyoto rats. Hypertens Res. 2003; 26:97–106. PMID: 12661918.


20. Holz GG 4th, Rane SG, Dunlap K. GTP-binding proteins mediate transmitter inhibition of voltage-dependent calcium channels. Nature. 1986; 319:670–672. PMID: 2419757.


21. Semtner M, Schaefer M, Pinkenburg O, Plant TD. Potentiation of TRPC5 by protons. J Biol Chem. 2007; 282:33868–33878. PMID: 17884814.


22. Kuriyama H, Suzuki H. Changes in electrical properties of rat myometrium during gestation and following hormonal treatments. J Physiol. 1976; 260:315–333. PMID: 978524.


23. Sanborn BM. Relationship of ion channel activity to control of myometrial calcium. J Soc Gynecol Investig. 2000; 7:4–11.


24. Parkington HC, Coleman HA. Excitability in uterine smooth muscle. Front Horm Res. 2001; 27:179–200. PMID: 11450426.


25. Guharay F, Sachs F. Stretch-activated single ion channel currents in tissue-cultured embryonic chick skeletal muscle. J Physiol. 1984; 352:685–701. PMID: 6086918.


26. Ohmori H. Mechanoelectrical transducer has discrete conductances in the chick vestibular hair cell. Proc Natl Acad Sci U S A. 1984; 81:1888–1891. PMID: 6584923.


27. Wellner MC, Isenberg G. Stretch effects on whole-cell currents of guinea-pig urinary bladder myocytes. J Physiol. 1994; 480:439–448. PMID: 7869258.


28. Pedersen SF, Nilius B. Transient receptor potential channels in mechanosensing and cell volume regulation. Methods Enzymol. 2007; 428:183–207. PMID: 17875418.


29. Christensen AP, Corey DP. TRP channels in mechanosensation: direct or indirect activation? Nat Rev Neurosci. 2007; 8:510–521. PMID: 17585304.


30. Raoux M, Colomban C, Delmas P, Crest M. The amine-containing cutaneous irritant heptylamine inhibits the volume-regulated anion channel and mobilizes intracellular calcium in normal human epidermal keratinocytes. Mol Pharmacol. 2007; 71:1685–1694. PMID: 17384225.


31. Strotmann R, Harteneck C, Nunnenmacher K, Schultz G, Plant TD. OTRPC4, a nonselective cation channel that confers sensitivity to extracellular osmolarity. Nat Cell Biol. 2000; 2:695–702. PMID: 11025659.


32. Liedtke W, Choe Y, Martí-Renom MA, Bell AM, Denis CS, Sali A, Hudspeth AJ, Friedman JM, Heller S. Vanilloid receptor-related osmotically activated channel (VR-OAC), a candidate vertebrate osmoreceptor. Cell. 2000; 103:525–535. PMID: 11081638.


33. Maroto R, Raso A, Wood TG, Kurosky A, Martinac B, Hamill OP. TRPC1 forms the stretch-activated cation channel in vertebrate cells. Nat Cell Biol. 2005; 7:179–185. PMID: 15665854.


34. Spassova MA, Hewavitharana T, Xu W, Soboloff J, Gill DL. A common mechanism underlies stretch activation and receptor activation of TRPC6 channels. Proc Natl Acad Sci U S A. 2006; 103:16586–16591. PMID: 17056714.


35. Dietrich A, Kalwa H, Storch U, Mederos y Schnitzler M, Salanova B, Pinkenburg O, Dubrovska G, Essin K, Gollasch M, Birnbaumer L, Gudermann T. Pressure-induced and store-operated cation influx in vascular smooth muscle cells is independent of TRPC1. Pflugers Arch. 2007; 455:465–477. PMID: 17647013.


36. Gottlieb P, Folgering J, Maroto R, Raso A, Wood TG, Kurosky A, Bowman C, Bichet D, Patel A, Sachs F, Martinac B, Hamill OP, Honoré E. Revisiting TRPC1 and TRPC6 mechanosensitivity. Pflugers Arch. 2008; 455:1097–1103. PMID: 17957383.


37. Schnitzler JG, Siebert U, Jepson PD, Beineke A, Jauniaux T, Bouquegneau JM, Das K. Harbor porpoise thyroids: histologic investigations and potential interactions with environmental factors. J Wildl Dis. 2008; 44:888–901. PMID: 18957645.


38. Dalrymple A, Slater DM, Poston L, Tribe RM. Physiological induction of transient receptor potential canonical proteins, calcium entry channels, in human myometrium: influence of pregnancy, labor, and interleukin-1 beta. J Clin Endocrinol Metab. 2004; 89:1291–1300. PMID: 15001625.
39. Jemal I, Soriano S, Conte AL, Morenilla C, Gomis A. G proteincoupled receptor signalling potentiates the osmo-mechanical activation of TRPC5 channels. Pflugers Arch. 2014; 466:1635–1646. PMID: 24177920.


40. Gomis A, Soriano S, Belmonte C, Viana F. Hypoosmotic- and pressure-induced membrane stretch activate TRPC5 channels. J Physiol. 2008; 586:5633–5649. PMID: 18832422.


41. Storch U, Mederos y Schnitzler M, Gudermann T. G protein-mediated stretch reception. Am J Physiol Heart Circ Physiol. 2012; 302:H1241–H1249. PMID: 22227128.


42. Zou Y, Akazawa H, Qin Y, Sano M, Takano H, Minamino T, Makita N, Iwanaga K, Zhu W, Kudoh S, Toko H, Tamura K, Kihara M, Nagai T, Fukamizu A, Umemura S, Iiri T, Fujita T, Komuro I. Mechanical stress activates angiotensin II type 1 receptor without the involvement of angiotensin II. Nat Cell Biol. 2004; 6:499–506. PMID: 15146194.


43. Yasuda N, Miura S, Akazawa H, Tanaka T, Qin Y, Kiya Y, Imaizumi S, Fujino M, Ito K, Zou Y, Fukuhara S, Kunimoto S, Fukuzaki K, Sato T, Ge J, Mochizuki N, Nakaya H, Saku K, Komuro I. Conformational switch of angiotensin II type 1 receptor underlying mechanical stress-induced activation. EMBO Rep. 2008; 9:179–186. PMID: 18202720.


44. Mederos y Schnitzler M, Storch U, Meibers S, Nurwakagari P, Breit A, Essin K, Gollasch M, Gudermann T. Gq-coupled receptors as mechanosensors mediating myogenic vasoconstriction. EMBO J. 2008; 27:3092–3103. PMID: 18987636.


SUPPLEMENTARY MATERIALS
Supplementary data including one figure can be found with this article online at http://pdf.medrang.co.kr/paper/pdf/Kjpp/Kjpp018-06-09-s001.pdf
Figure S1
Effect of 2-APB on myometrial contraction evoked by high K-solution and voltage-dependent Ca2+ current in pregnant rat myometrium. (A) Left: a representative trace of 2-APB (10 µM) effect on myometrial contraction evoked by high-K (70 mM) solution. The high K-evoked contraction was completely abolished by 10 µM nifedipine. Right: summary of 2-APB effect on the high K-evoked contraction. (B) Left: a representative trace of 2-APB (10 mM) effect on voltage-dependent Ca2+ current in pregnant rat myocyte. Right: summary of 2-APB effect on voltage-dependent Ca2+ current.
Figure S2
Ionic selectivity of hypoosmotic swelling-induced outward rectifying current in rat pregnant myocyte. Bath solution 120 mM NMDG+. (A) I-V relationship of hypoosmotic swelling-induced current. (B) summary of normalized amplitude of hypoosmotic swelling-induced current. The current amplitudes in each groups were measured at -60 and +60 mV respecvtively.
Figure S3
Anion permeability of hypoosmotic swelling-induced outward rectifying current in rat pregnant myocyte. Pipette solution contained 50 mM CsCl + 70 mM Cs - methanesulfonate. (A) I-V relationship of hypoosmotic swelling-induced current. (B) summary of normalized amplitude of hypoosmotic swelling-induced current. The current amplitudes in each groups were measured at -60 and +60 mV respecvtively.
Fig. 1
Hypoosmotic swelling-induced outward rectifying current in rat pregnant myocyte. (A) A representative trace of whole cell current induced by hypoosmotic swelling. Membrane potential of myocyte was held at -60 mV. (B) I~V relationship of hypoosmotic swelling-induced current. The current was obtained from the same cell as in (A). (C) Summary of normalized amplitude of hypoosmotic swelling-induced current. The current amplitudes in each groups were measured at -60 and +60 mV respecvtively. *p<0.05, **p<0.01, ***p<0.001.
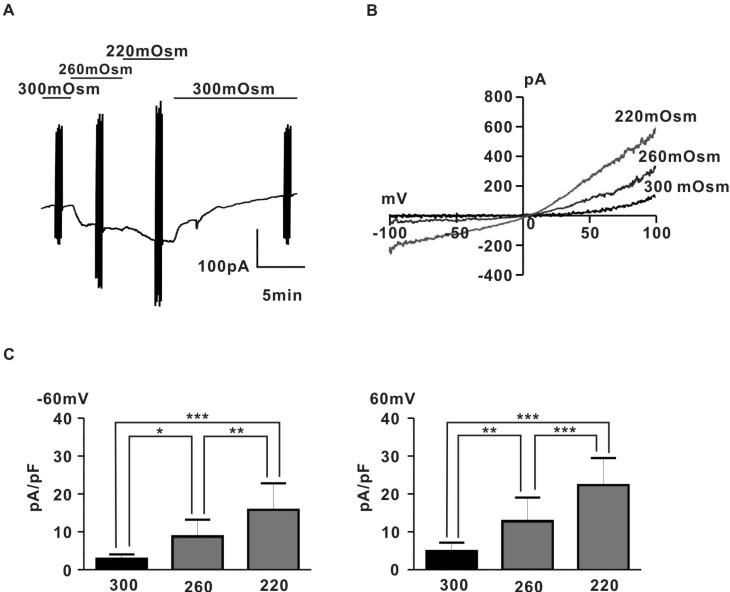
Fig. 2
Effect of GDPβS on Ihypo. (A) Left, Ihypo was recorded in rat pregnant myocyte. The representative I~V relationships of Ihypo by voltage ramp of -100 to 100 mV during 500-ms durations. Right, summary of normalized amplitude of Ihypo. The current amplitudes in each group were measured at -60 and +60 mV respectively. (B) A representative trace of Ihypo in the presence of GDPβS (2 mM) in pipette solution. Hypoosmotic solution was applied to pregnant rat myocyte after 7 min dialysis with GDPβS in the pipette solution. Right, summary of normalized amplitude of Ihypo. The current amplitudes in each group were measured at -60 and +60 mV respectively. (A) *p<0.05, **p<0.01. (B) p>0.05.
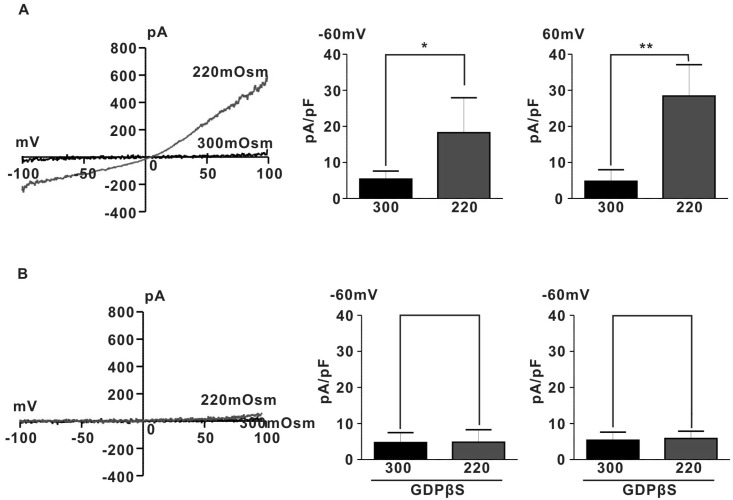
Fig. 3
Effects of Lanthanides and 2-APB on Ihypo. (A) Left, I~V relationship of Ihypo in presence of Gd3+ and Gd3+ plus 2-APB. Right, summary of effects for Gd3+ and Gd3+ plus 2-APB on Ihypo. (B) Left, I~V relationship of Ihypo in presence of La3+ and La3+ plus 2-APB. Right, summary of effects for La3+ and La3+ plus 2-APB on Ihypo. *p<0.05, **p<0.01, ***p<0.001.
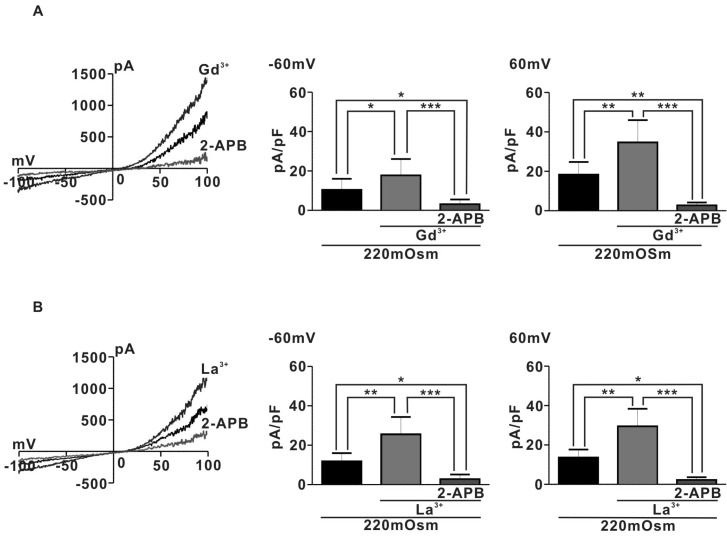
Fig. 4
Effects of Lanthanide and 2-APB on stretch-induced contraction in rat pregnant myometrium. (A) Left, a representative trace of augmenting effect of 1µM Gd3+ on SMC and effect of 2-APB on the augmenting effect of Gd3+. Right, a representative trace of augmenting effect of 1µM La3+ on SMC and effect of 2-APB on the augmenting effect of La3+ (B) Left, summary of effects for Gd3+ and Gd3+ plus 2-APB on mean frequency and amplitude of SMC. Right, summary of effects for La3+ and La3+ plus 2-APB on mean frequency and amplitude of SMC. **p<0.01.
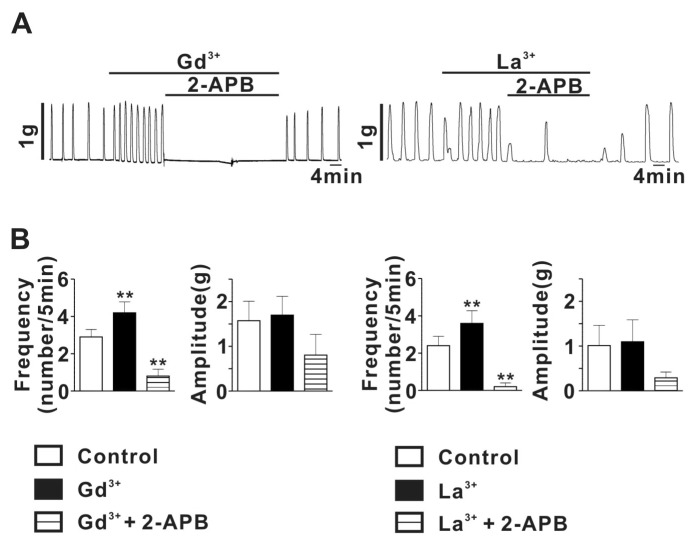