Abstract
Ulcerative colitis is an inflammatory bowel disease (IBD) characterized by recurrent episodes of colonic inflammation and tissue degeneration in human or animal models. The contractile force generated by the smooth muscle is significantly attenuated, resulting in altered motility leading to diarrhea or constipation in IBD. The aim of this study is to clarify the altered contractility of circular and longitudinal smooth muscle layers in proximal colon of trinitrobenzen sulfonic acid (TNBS)-induced colitis mouse. Colitis was induced by direct injection of TNBS (120 mg/kg, 50% ethanol) in proximal colon of ICR mouse using a 30 G needle anesthetized with ketamin (50 mg/kg), whereas animals in the control group were injected of 50% ethanol alone. In TNBS-induced colitis, the wall of the proximal colon is diffusely thickened with loss of haustration, and showed mucosal and mucular edema with inflammatory infiltration. The colonic inflammation is significantly induced the reduction of colonic contractile activity including spontaneous contractile activity, depolarization-induced contractility, and muscarinic acetylcholine receptor-mediated contractile response in circular muscle layer compared to the longitudinal muscle layer. The inward rectification of currents, especially, important to Ca2+ and Na+ influx-induced depolarization and contraction, was markedly reduced in the TNBS-induced colitis compared to the control. The muscarinic acetylcholine-mediated contractile responses were significantly attenuated in the circular and longitudinal smooth muscle strips induced by the reduction of membrane expression of canonical transient receptor potential (TRPC) channel isoforms from the proximal colon of the TNBS-induced colitis mouse than the control.
Ulcerative colitis is an inflammatory bowel disease (IBD) characterized by recurrent episodes of colonic inflammation and tissue [1,2]. In humans, as well as in several different animal models of intestinal inflammation, the contractile force generated by the smooth muscle is significantly reduced, resulting in altered motility leading to diarrhea or constipation [3]. In IBD, intestinal dysfunctions as well as critical morphological changes have been reported in both smooth muscle and intrinsic [4,5], including bowel wall thickening [6,7], nerve ending proliferation, alteration of neurotransmitter contents and release, and altered in vitro contractile responses to neurotransmitters or mediators [8].
The upstroke of the gastrointestinal smooth muscle action potential is largely mediated by Ca2+ influx through voltage-gated L-type Ca2+ channels [9]. Disturbance of L-type Ca2+ channel activity has been reported as one of the causes of reduced colonic motility [10,11]. Patch clamp recordings of smooth muscle cells demonstrate significant decrease in the amplitude of the Ca2+ currents associated with decreased protein expression of pore forming α 1 subunit from inflamed tissues in dextran-sulphate sodium (DSS) and trinitrobenzene sulphonic acid (TNBS)-induced colitis models [12,13]. Although L-type Ca2+ channel currents were down-regulated, adenosine triphosphate (ATP)-sensitive K+ (KATP) channels are up-regulated in gastrointestinal smooth muscle cells from the DSS- and TNBS-induced colitis mouse, which is induced hyperpolarization of the gastrointestinal smooth muscle cells [14].
Acetylcholine is the principal excitatory neurotransmitter of the enteric nervous system and plays an essential role in the peristaltic activity in gastrointestinal tract [9]. In the smooth muscle cell of gastrointestinal tract, pharmacological and binding studies have typically shown the presence of muscarinic acetylcholine type 2 (M2R) and type 3 receptor (M3R), which are linked to the Gi/o and Gq/11 proteins, respectively [15]. Inflammation not only suppresses the amplitude of the muscarinic-induced contractions, but also appears to shift the degree of activation of the M2R and its associated signaling pathway [16,17].
Besides the changes on the expression level of L-type Ca2+ channels, KATP channels, and M2R and M3R, an underlying cause of the reduced contractility was investigated in circular and longitudinal smooth muscle layer from the proximal colon in TNBS-induced colitis mouse. The aims of this study were to confirm the alteration of 1) the structural changes of mucosal and muscular layer, 2) spontaneous contractile activity and depolarization-induced contractility, 3) muscarinic acetylcholine receptor (mAchR)-mediated contractility and the responses of mAchR agonists of circular and longitudinal smooth muscle cells in proximal colon of TNBS-induced colitis mouse compared to the vehicle-treated control.
Healthy adult male ICR mouse, weighing 30~35 g (Orient Bio, Seongnam, Korea), were anesthetized with intraperitoneal injection of ketamin (50 mg/kg). The abdomen was opened by midline laparotomy and the proximal colon was gently extruded. Colitis was induced by direct injection of 120 mg/kg TNBS (Tokyo Kasei Kogyo, Tokyo, Japan) in 50% ethanol using a 30 G needle into the colonic lumen 1 cm distal to the cecal-colonic junction. Animals in the normal control group were handled similarly, but 50% ethanol alone was administered instead. Animals in the control and TNBS-induced colitis group were used within 2 days.
Two days after the induction of inflammation, the proximal colon was opened along the mesenteric border and the luminal contents were washed in normal saline. Opened segments were pinned to the silicon base of dishes with the mucosal side facing up, and fixed in 10% formalin for 12 h, and washed in tap water for 12 h, and paraffin-embedded tissue sections were stained with hematoxylin and eosin.
The colon was excised from 50% ethanol-treated control group and TNBS-induced colitis ICR mouse. The muscular layer was isolated from mucosal layer of proximal colon. The muscle strips (1×1 cm) were mounted with a preload of 1 g along their circular or longitudinal axis in a bath filled with Krebs solution that contained (in mM): 137 NaCl, 5.4 KCl, 1.5 CaCl2, 1 MgCl2, 23.8 NaHCO3, and 5.5 glucose saturated with 95% O2 and 5% CO2 at 37℃. The responses of the strips were recorded with PowerLab/400 on a Chart 4.0. The muscle contraction was measured isometrically with a force-displacement transducer (MLT050; AD Instruments, Colorado Springs, CO, USA) and recorded with PowerLab/400 on a chart program (AD Instrumrnts).
The circular muscle layer isolated from proximal colon were placed in Ca2+-free Tyrode solution that contained (in mM): 135 NaCl, 5 KCl, 1 MgCl2, 10 glucose, and 10 HEPES (pH 7.4, adjusted with NaOH) and cut into small pieces of 2~3 mm in length with fine scissors. The muscle pieces were incubated in Tyrode solution for 30 min and then in a solution containing 2 mg/ml collagenase (Amano Enzyme Inc., Nakaku, Nagoya, Japan), 2 mg/ml papain (Roche, Indianapolis, IN, USA), 1 mg/ml bovine serum albumin (Sigma, St. Louis, MO, USA), and 30 µM Ca2+ at 37℃ for 45 min. The tissues were placed in sterilized Tyrode solution containing no enzyme, and triturated with a blunted-tipped pipette until a sufficient number of single cells were isolated. The freshly isolated cells were used in electrophysiological experiments.
Non-selective cationic currents (NSCCs) were recorded using the whole-cell variant of the patch clamp technique as described previously [18]. Patch electrodes were fabricated from a borosilicate glass capillary (Corning 7052, Garner Glass Co., Claremont, CA) using a P-97 Flaming Brown micropipette puller (Sutter Instrument Co., San Rafel, CA). The patch electrodes were fire polished on a microforge (Narishige, Tokyo, Japan), and had resistances of 1~3 MΩ when filled with the internal solution (in mM): 145 cesium glutamate, 8 NaCl, 2 ATP, 0.3 GTP, 10 EGTA, 1 free Mg2+, and 0.00001 free Ca2+, pH adjust to 7.4 (290 mOsm/kg H2O). An Ag/AgCl pellet was used to ground the bath. External recording solution contained (in mM): 145 NaCl, 2.8 KCl, 10 CsCl, 2 MgCl2, 10 glucose, 0.2 EGTA, 10 HEPES, 0.05 free Ca2+, pH adjusted to 7.4 (320 mOsm/kg H2O). Drugs were applied to cells via a gravity-fed fused silica capillary tube connected to an array of seven polyethylene tubes. The outlet of the perfusion system was located within 100 µm of the cell. The bath fusion rate was approximately 1~2 ml/min. The cell membrane capacitance and series resistance were compensated (>80%) electronically using an Axopatch-200B amplifier (Axon Instruments, Foster City, CA). Voltage protocol generation and data acquisition were performed using pClamp 6.0 software on a IBM computer equipped with an analog-to-digital converter (Digidata 1200, Axon Instruments). Current traces were generally low-pass filtered at 5 kHz using the 4-pole Bessel filter in the clamp amplifier, digitized at 2 kHz, and stored on the computer hard drive for later analysis.
Total RNA of the colonic smooth muscle cells was extracted using RNAiso plus reagent (TAKARA Bio, Otsu, Shiga, Japan). The concentration and purity of the total RNA were measured with ultraviolet spectrophotometer. Synthesis of the first strand cDNA was performed in a RT-PCR buffer containing 1 µg of total RNA using 200 U M-MLV (Moloney Murine Leukemia Virus) reverse transcriptase (Promega Co., Madison, WI, USA), 25 nM dNTP, 1 µg oligo dT, 20 U RNase inhibitor in a final volume of 25 µl at 42℃ for 1 h. The polymerase chain reaction (PCR) to detect transcripts of transient receptor potential (TRP) channel isoforms was performed by following steps. 1) single strand cDNA products were denatured at 94℃ for 1 min, then, subjected to PCR amplication (35 cycles). Each PCR cycle consisted of denaturing at 94℃ for 30 sec, annealing at 63℃ for 30 sec, and extension at 72℃ for 1 min in a minicycler (MJ Research Inc., Watertown, MA, USA). PCR buffer (50 µl) contained the transcribed cDNA, 10 pM primers, 10 pM dNTP, and 1.25 U of Tag polymerase (PerkinElmer, Norwalk, CT, USA). As an internal reference, glyceraldehyde-3-phosphate dehydrogenase (GapDH) gene was amplified. The resultant PCR products were separated on a 1.5% agarose gel and visualized by ethidium bromide (EtBR) staining. Specific PCR primers for TRP channel isoforms are listed in Table 1.
Real-time RT-PCR analysis was performed to quantify colitis-induced changes in gene expression. PCR amplification was carried out in an Rotor-Gene 3,000 thermal cycler (Corbett Research, Sydney, Australia) using the QuantiTect SYBR Green PCR kit (Quiagen, Valencia, CA, USA) in according to the manufactures instructions. Reaction mixture contained 1× QuantiTect SYBR Green PCR Master Mix (containing HotStarTaq DNA polymerase, PCR buffer, and SYBR Green 1), 500 ng template cDNA, and 0.5 µM primer pairs in a total volume of 10 µl. The mixture was initially heated at 95℃ for 15 min and cycled 45 times with two steps of melting at 95℃ for 15 sec and annealing/extending at 60℃ for 1 min. All reactions were carried out in triplicate. The specific DNA product was confirmed by analysis of a melting curve generated at the end of PCR. Primer sequences were summarized in Table 2. As an internal reference, the β-actin gene was amplified. To analyze the relative changes in gene expression from the real-time RT-PCR experiments, the comparative method was employed [19].
Morphological changes of proximal colon confirmed by histological analysis after experimental colitis induced by direct injection of 2,4,6-trinitrobenzenesulfonic acid (TNBS) in proximal colon using the surgical method. As shown in Fig. 1A, the control mice administrated 50% ethanol displayed little evidence of inflammation in the mucosal and muscular layers of the colon. At 2 days after inject to TNBS (120 mg/kg), the wall of the colon is diffusely thickened with loss of haustration and folding, and there is evidence of submucosal edema and distortion of mucosal architecture. Also, extensive infiltration by inflammatory cells, including polymorphonuclear leukocytes, lymphocytes and macrophages, was apparent in the muscular layer (Fig. 1B).
It is examined that the effect of the TNBS-induced colonic inflammation on the spontaneous rhythmic contraction evoked from the proximal colonic circular and longitudinal smooth muscle layers. Fig. 2 shows the typical spontaneous contractile activity (SCA) of circular smooth muscle strips isolated from the control and TNBS-induced colitis proximal colon. As shown in Fig. 2A, at 2 days after induction of colitis, the SCA of circular smooth muscle layer in proximal colon was markedly reduced the amplitude (from 0.41±0.17 to 0.07±0.03 g, n=5) and frequency (from 3.1±0.3 to 1.8±0.2 cycles/min, n=5) (Fig. 2C and D). In longitudinal smooth muscle layer, however, after induction of colitis, the SCA was slightly reduced the amplitude (from 0.22±0.08 to 0.07±0.03 g, n=8) and frequency (from 2.2±0.8 to 1.5±0.6 cycles/min, n=5) (Fig. 2B, E, F).
It is also examined the alteration in depolarization-induced contractile response of the circular and longitudinal smooth muscle layer from the inflamed proximal colon. As shown in Fig. 3A and C, the maximum active tension of circular muscle layer evoked by high K+ (50 mM), an artificial condition assumed to neurotransmitter-mediated depolarization, was markedly attenuated by TNBS-induced colonic inflammation (0.34±0.09 g, n=5) than the control (1.28±0.16 g, n=5). In case of longitudinal smooth muscle layer, however, the high K+-induced maximum tension was slightly decreased in TNBS-induced inflamed colon (0.20±0.04 g, n=4) than the control (0.47±0.09 g, n=4) (Fig. 3B and D).
As illustrated in Fig. 4A and C, carbachol, a non-specific muscarinic acetylcholine receptor (mAchR) agonist, caused a concentration-dependent increase of contraction in both circular and longitudinal smooth muscle strips from proximal colon of the control and TNBS-induced colitis mouse. However, the contractile response to cholinergic receptor stimulation by carbachol was markedly reduced in the circular (Fig. 4B) and longitudinal (Fig. 4D) smooth muscle strips from the proximal colon of the TNBS-induced colitis mouse than the control. Indeed, in circular smooth muscle strips from the control, absolute maximum tension induced by carbachol (10-5 M) was 2.06±0.19 g (n=7), whereas, it's from the TNBS-induced colitis, maximum tension was 0.47±0.04 g (n=7) (Fig. 5A). However, absolute maximum tension induced by carbachol (10-5 M) in the longitudinal smooth muscle strips were slightly decreased in TNBS-induced colitis (0.76±0.10 g, n=7) than the control (0.37±0.04 g, n=7) (Fig. 5B). Besides the carbachol, oxotremorine M, a specific muscarinic acetylcholine receptor agonist, was also evoked concentration-dependent contractile response in circular and longitudinal smooth muscle strips from the proximal colon of the control and TNBS-induced colitis, however, contractile response in circular and longitudinal smooth muscle strips were markedly attenuated in TNBS-induced colitis compared to the control (Fig. 5C and D).
To clarify the reduced contractility of circular smooth muscle layer, the non-selective cation channel (NSCC) currents, a routine source for elevation of intracellular Ca2+ concentration, were confirmed. The carbachol-induced NSCC currents were recorded in the circular smooth muscle cells from the proximal colon of the control and TNBS-induced colitis mouse using the whole cell patch clamp configuration, replacing K+ with Cs+ (145 mM) to block K+ channels and using nimodipine (1 µM) to block L-type Ca2+ channels. As shown in Fig. 6A, application of carbachol (1 µM) induced NSCC currents in circular smooth muscle cells. The current-voltage relationship of carbachol-induced NSCC currents revealed a pattern of inward or outward rectification and a reversal potential of 0 mV. These rectifications of current-voltage relation were markedly reduced by TNBS-induced colonic inflammation. The inward rectification of the NSCC currents, especially, important to Ca2+ and Na+ influx-induced depolarization and contraction, was markedly reduced in the TNBS-induced colitis (8.4±6.7 pA, n=6) compared to the control (56.8±9.8 pA, n=6) at -60 mV of the membrane potential (Fig. 6B).
RT-PCR was performed to confirm the canonical transient receptor potential (TRPC) expression pattern in the circular smooth muscle cells from the mouse proximal colon, and reveal the changes of the TRPC expression levels on TNBS-induced colonic inflammatory condition. Fig. 7 illustrated that the expression pattern of the TRPC channel isoforms in the whole brain, and proximal colonic smooth muscle cells of the control and TNBS-induced colitis mouse. The TRPC channel isoforms, classified to store-operated channels (TRPC1/4/5) and receptor-operated channels (TRPC3/6/7), are fully expressed in the whole brain and proximal colonic smooth muscle cells of the ICR mouse. Particularly, the expression level of the TRPC channel isoforms were markedly reduced in proximal colonic smooth muscle cells from the TNBS-induced colitis mouse (lower panel in Fig. 7). These results are not demonstrated the absolute expression levels because conventional RT-PCR is the qualitative analysis methods. Accordingly, quantitative real-time RT-PCR was performed to clarify the reduction amounts of the TRPC channel expression in the colonic smooth muscle cells from the TNBS-induced colitis mouse compared to the control. As shown in Fig. 8, consistent to the muscle tension and patch clamp data, quantitative real-time RT-PCR revealed that TNBS-induced colonic inflammation down-regulated the mRNA encoding TRPC1 (0.22), TRPC2 (0.14), TRPC3 (0.01), TRPC4 (0.01), TRPC5 (0.01), TRPC6 (0.01), TRPC7 (0.01) than the control, TRPC1 (0.54), TRPC2 (1.00), TRPC3 (0.12), TRPC4 (0.17), TRPC5 (0.23), TRPC6 (0.70), TRPC7 (0.46), normalized to expression amounts of the whole brain.
Gastrointestinal motility disorder is a common characteristic of intestinal inflammation in human and drug- or infection-induced animal models [20]. Intestinal inflammation results in the disturbance of motility, which may reflect alteration in smooth muscle function and enteric nervous system [21]. Several animal models have been used to study the pathogenesis of inflammatory bowel disease (IBD), including Chron's disease and ulcerative colitis. Animal models of gastrointestinal inflammation can be divided into four classes: spontaneous colitis models, inducible colitis models with a normal immune system, adoptive transfer models in immuno-compromised hosts, and genetically engineered models [22]. In several established animal models, inducible colitis model is frequently used to study for inflammation-induced motility dysfunction and mechanisms, particularly, TNBS- [23], oxazolone- [24], DSS- [25], carrageenan- [26], and peptidoglycan-polysaccharide (PG-PS)-induced colitis models [27]. Especially, TNBS induces acute inflammation that progresses over several weeks to a chronic stage [23,28]. Typically, mucosal injury and inflammatory cell infiltration are observed within 2 hours, and the features of chronic inflammation and lymphocytic cell infiltration occur at 48 hours after exposure to TNBS and persist over several weeks [29,30]. Several evidences suggest that the colitis seen in TNBS model is regarded as a major model of ulcerative colitis and Crohn's disease [22]. Therefore, the present study was used the TNBS-induced colitis model to investigate the mechanisms for colitis-induced colonic motility changes. Previously, it was reported that TNBS treatment would induce changes the colonic architectures of the mucosal and muscular layer, such as loss of haustration and folding of the colon wall, submucosal and mucosal edema, infiltration of inflammatory cells, and segmentation of the muscular layer [31]. We have previously reported that mucosal architecture changes in ulcerative and tuberculous colitis patients in human [32]. In the present study, it was clearly confirmed that alteration of colonic architectures induced by TNBS-induced colitis using histological analysis with hematoxylin-eosin staining (Fig. 1B).
Typically, the normal colon generates at least several types of contractile patterns in vivo. These are phasic contractions, tone, migrating motor complexes, and giant migrating contractions [33]. These contractile patterns are under the control of several systems including the neurogenic, myogenic, and endocrine systems [34]. Recent morphological and functional studies suggest that the interstitial cells of Cajal (ICCs) are pacemakers in the musculature of the gastrointestinal tract [35,36]. The ICCs are arranged in distinctive patterns close to nerve and smooth muscle cells in the muscle layer [37,38], where they generate pacemaker currents to develop electrical slow waves in the gastrointestinal smooth muscles [36]. ICC is almost completely abolished within the longitudinal and circular muscle layers and numbers of ICC at the level of the myenteric and deep muscular plexuses are significantly reduced in tissues from patients with Crohn's disease [39]. The damage to the ICC network represents an underlying morphologic substrate for decreased smooth muscle contractile activity, thereby contributing to the inhibition of smooth muscle contraction [34]. Therefore, in the present study, alterations of spontaneous contractile activity were investigated in circular and longitudinal smooth muscle strips isolated from the proximal colon of the control and TNBS-induced colitis mouse (Fig. 2), and found that the amplitude and frequency of spontaneous contractile activity were markedly reduced in circular muscle strips, whereas, slightly reduced in longitudinal muscle of the TNBS-induced colitis mouse (Fig. 2).
The rising of the gastrointestinal smooth muscle action potential is considerably mediated by Ca2+ influx through voltage-gated L-type Ca2+ channels. Down-regulation of these channel activity has been reported that one of the factors of reduced gastrointestinal motility [10,11]. The present study is also found that the contractile activity evoked by high K+ (50 mM), a similar condition for L-type Ca2+ channel activation, was markedly reduced in circular and longitudinal smooth muscle strips from the TNBS-induced colonic inflammation mouse compared to the control (Fig. 3). Initially, it was reported that the activity of L-type Ca2+ channels markedly down-regulated in DSS-induced experimental colitis [10]. It was also reported that the expression of the α 1C main subunit of L-type Ca2+ channels was significantly attenuated in acetic acid and ethanol-induced gastrointestinal inflammation, and resulting associated with suppression of current densities [40]. On the other hand, several groups have reported that the expression of the α 1C main subunit remained unchanged, although the activity of L-type Ca2+ channels was decreased, in the TNBS-induced and DSS-treated colitis models [13,41]. These contrary reports may be due to differences in the methods used to induce colonic inflammtion and in the animal species [42]. Recently, it was reported that tumor necrosis factor α (TNF α)-induced nuclear factor-κ B (NF-κ B) resulted in suppression of the α 1C mRNA expression, as the possible mechanisms of down-regulated α 1C subunit expression in colonic smooth muscle cells in inflammatory conditions [43]. More recently, however, it has been reported that the suppression of mRNA expression of the α 1C subunit is not only mechanism involved in decreased α 1C protein expression, and that mechanisms such as accelerated protein degradation and decreased mRNA stability are involved, because of the half life of the α 1C subunit was about 14 days through the study using the smooth muscle specific α 1C knockout mouse [44].
Acetylcholine is regarded to be the principal neurotransmitter involved in gastrointestinal smooth muscle contraction in various contractile factors. Muscarinic acetylcholine receptor (mAchR) type 2 (M2R) and type 3 (M3R) are thought to be involved in contractile activity of the gastrointestinal smooth muscles [45,46], although, which are expressed five isoforms of mAchR (M1R-M5R) [47]. It has been reported that the M2R can directly activate L-type Ca2+ channels and non-selectivive cation channel (NSCC) via c-src, phosphoinositide 3-kinase (PI3K) and protein kinase C (PKC), and contribute to gastrointestinal smooth muscle contraction [48]. In a TNBS-induced guinea-pig inflammation model, although the acetylcholine-mediated contractile force of the circular smooth muscle is signifintly decreased, that of longitudinal smooth muscle is increased [3]. In acetic acid-induced dog inflammation model, the acetylcholine-mediated contractile activity is unchanged in the longitudinal smooth muscle cells, whereas that is decreased in circular smooth muscle cells [49]. In this study, carbachol-, an acetylcholine receptor non-selective agonist, induced contractile activity is significantly decreased over 10 folds in circular smooth muscle strips from the proximal colon of the TNBS-induced colitis mouse compared to the control (Fig. 4A and 4B). On the one hand, carbachol-induced contractility is slightly decreased or unchanged in longitudinal smooth muscle strips from the TNBS-induced colitis mouse than the control (Fig. 4C and 4D). These differences in responses to colonic inflammation between circular and longitudinal smooth muscle strips may be due to the degree of dependence on Gi/o protein-coupled M2R for carbachol-induced contractility [49]. On the other hand, circular smooth muscle contraction may be principally mediated by Gq/11 protein-coupled M3R, whereas the longitudinal smooth muscle contraction may be mainly mediated by M2R [50]. This may be explained by the model proposed Ehlert, in which M2R is a conditional receptor but M3R is the direct acting receptor for muscarinic stimulation by acetylcholine [18]. When inflammation shifts the balance of involvement of M2R and M3R in gastrointestinal smooth muscle contraction toward predominance of M2R, the effect of this change in balance is limited to the longitudinal smooth muscle, because M2R are not involved in circular smooth muscle contraction [49,50].
It is noteworthy that muscarinic stimulation induces a depolarization or evokes excitatory junction potentials, and causes the excitation-contraction coupling of the gastrointestinal smooth muscle cells [51]. Depolarization of the gastrointestinal smooth muscle cells induced by mAchR activation is evoked to the non-selective cation channel (NSCC) currents [52,53]. Recently, it has been reported that the canonical transient receptor potential (TRPC) channels were principal candidate for NSCC activated by mAchR activation [54]. The TRPC channels all appear to be activated in response to phospholipase C (PLC)-coupled receptors [55]. Within the TRPC channel family, there are two structurally divided subgroups, which is receptor-operated cation channels (TRPC3/6/7) and store-operated cation channels (TRPC1/4/5) [56]. One functional characteristic distinguishing these two subgroups is the ability of diacylglycerol (DAG) to activate TRPC3/6/7 channels, whereas TRPC1/4/5 activate intracellular Ca2+ store depletion [57]. Therefore, TRPC channels can be activated in response to the PLC-coupled mAchR activation [55]. Although, a numerous study of the motility dysfunctions in gastrointestinal inflammation were intensively accomplished, a small minority study of the NSCC were achieved to reveal a cause for down-regulation of the mAchR-mediated gastrointestinal motility in inflammation condition. In the present study, NSCC channel currents were confirmed to clarify the reduced contractility of circular smooth muscle from the TNBS-induced colitis mouse. The carbachol-induced inwardly rectified cationic currents were significantly reduced in membrane potentials below 0 mV (Fig. 6). These results suggested that the reduced Ca2+ and Na+ influx through the smooth muscle membrane by carbachol induced to the attenuation of contractile and depolarizing force of smooth muscle cells. To clarify the resources for the reduction of NSCC channel currents, the membrane expression of TRPC channel isoforms were investigated in total RNA from the proximal colonic smooth muscle cells using RT-PCR (Fig. 7) and quantitative realtime RT-PCR analysis (Fig. 8). All isoforms of TRPC channel are significantly reduced the expression levels in TNBS-induced colitis mouse comparaed to the control (Fig. 8). Taken together, these data suggested that mAchR-mediated gastrointestinal motility reduction fundamentally caused by attenuation of the NSCC channel current density originated from the down-expression of TRPC channel isoforms in proximal colonic smooth muscle cells from the TNBS-induced colitis mouse. To date, it is not reported that the correlation between inflammation or inflammatory cytokines and TRPC channel functions. Consequently, collecting data propose the mechanisms for the functional failure of the smooth muscle cells in inflammatory conditions.
In conclusions, significant changes occur in morphological features of the colonic architectures, and mechanical properties and pharmacological responsiveness of circular than longitudinal muscle layer from the proximal colon of the TNBS-induced colitis mouse. The wall of the proximal colon in the TNBS-induced colitis mouse is diffusely thickened with loss of haustration, and showed mucosal and mucular edema with inflammatory infiltration. The TNBS-induced colitis is significantly induced the reduction of colonic contractile activity, such as spontaneous contractile activity, depolarization-induced contractility, and muscarinic acetylcholine receptor-mediated contractile response in circular muscle layer compared to the longitudinal muscle layer.
Figures and Tables
Fig. 1
Photomicrographs of sections from colon of a control (A) and TNBS-induced colitis mouse (B) stained with hematoxylin-eosin. Note the loss of haustration and folding, submucosal and mucosal edema, distortion of mucosal architecture, thickening of the muscularis mucosae, and appearance of infiltration by inflammatory cells in the muscle layer. Each frame indicates a typical trace out of a five independent experiments.
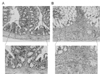
Fig. 2
Effects of TNBS-induced colonic inflammation on the SCA of the circular and longitudinal muscle layer from the proximal colon of the control and TNBS-induced colitis mouse. Representative traces for SCA of the control (A) and TNBS-induced colitis (B). SCA was recorded with a preload of 1 g along their circular axis in a bath filled with Krebs solution maintained at 37℃ in an atmosphere of 95% O2-5% CO2. The square box (right panel) present magnified rhythmic SCA traces (A, B). Summary for effects of TNBS-induced colonic inflammation on the average cycle rate and amplitude of SCA of the circular (C, D) and longitudinal (E, F) muscle layer from proximal colon. The data are represented to the mean±SD. Numbers in parenthesis are the number in a series of experiments. **p<0.01, ***p<0.005 vs control.
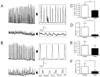
Fig. 3
Effects of TNBS-induced colonic inflammation on depolarization-induced contractility. Representative traces for high K+ (50 mM)-induced contractile response of the circular (A) and longitudinal (B) smooth muscle layer from the proximal colon of the control (left panel) and TNBS-induced colitis mouse (right panel). Contractile response was recorded with a preload of 1 g along their circular or longitudinal axis in a bath filled with Krebs solution maintained at 37℃ in an atmosphere of 95% O2-5% CO2. Summary of the contractile response induced by high K+ (50 mM) in circular (C) and longitudinal (D) smooth muscle layer from the proximal colon of the control and TNBS-induced colitis mouse. The data are represented to the mean±SD. Numbers in parenthesis are the number in a series of experiments. *p<0.05, ***p<0.005 vs control.
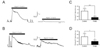
Fig. 4
Effects of TNBS-induced colonic inflammation on the carbachol-induced contractions in the circular (A, B) and longitudinal (C, D) smooth muscle layer from the proximal colon. Representative traces for contractile response induced by log scale concentration of carbachol (3×10-7~10-5 M) in proximal colonic circular (A, B) and longitudinal (C, D) smooth muscle layer from the control (A, C) and TNBS-induced colitis mouse (B, D).
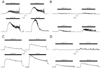
Fig. 5
Summary of the carbachol- and oxotremorine M-induced contractile responses in proximal colon. The response evoked by application of carbachol (A, B) in log scale concentration (3×10-7~3×10-5 M) and oxotremorine M (C, D) in log scale concentration (3×10-8~3×10-6 M) in the circular (A, C) and longitudinal (B, D) smooth muscle layer from the proximal colon of the control (○) and TNBS-induced colitis mouse (□). The data are represented to the mean±SD from seven experiments.
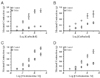
Fig. 6
Effects of TNBS-induced colonic inflammation on the NSCC currents in the circular smooth muscle cells. Whole cell currents were recorded under the condition of 145 mM Cs+ in internal solution. Slow ramp depolarizations from +100 mV to -100 mV were applied at a holding potential of -60 mV. Representative traces of the current-voltage relationship were obtained from the circular smooth muscle cells in control and inflamed proximal colon (A). Bar graphs show mean current amplitudes of carbachol-induced NSCC currents at -60 mV (B). The data are represented to the mean±SD. Numbers in parenthesis are the number in a series of experiments. ***p<0.005 vs control.
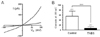
Fig. 7
Qualitative RT-PCR analysis of mRNA encoding TRPC channel isoforms expressed in whole brain (upper), control (middle), and TNBS-induced inflamed colon (lower panel). Each total RNA isolated from whole brain and dissociated circular smooth muscle cells of the control and TNBS-induced colitis mouse proximal colon were reverse transcribed, and amplified by PCR with TRPC channel isoform-specific primers (Table 1). The resultant PCR product were separated and visualized on an agarose gel containing ethidium bromide (EtBR). GapDH RNA as an internal control and whole brain RNA as a positive control were used.
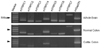
Fig. 8
Fold changes in gene expression of TRPC channel isoforms assessed by real-time quantitative RT-PCR. To analyze the relative changes in gene expression from the real-time PCR experiments, the comparative 2-△△CT method was used. The △CT value for each isoform gene was calculated using the equation: △CT (control or TNBS-induced colitis)=CT (TRPC isoform)-CT (reference, i.e., GapDH). The averaged CT value was obtained from triplicate of each PCR reaction. The fold change in gene expression of a TRPC isoform was finally obtained from the formula 2-△△CT, where the △△CT (TRPC isoform) value was the difference between △CT (colitis) and △CT (control) values. The point on the Y-axis (dotted line) indicates expression amounts in the whole brain. n=5. *p<0.05, **p<0.01, ***p<0.005 vs control.
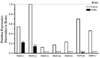
References
1. Reddy SN, Bazzocchi G, Chan S, Akashi K, Villanueva-Meyer J, Yanni G, Mena I, Snape WJ Jr. Colonic motility and transit in health and ulcerative colitis. Gastroenterology. 1991. 101:1289–1297.
2. Annese V, Bassotti G, Napolitano G, Usai P, Andriulli A, Vantrappen G. Gastrointestinal motility disorders in patients with inactive Crohn's disease. Scand J Gastroenterol. 1997. 32:1107–1117.
3. Martinolle JP, Garcia-Villar R, Fioramonti J, Bueno L. Altered contractility of circular and longitudinal muscle in TNBS-inflamed guinea pig ileum. Am J Physiol. 1997. 272:G1258–G1267.
4. Jacobson K, McHugh K, Collins SM. Experimental colitis alters myenteric nerve function at inflamed and noninflamed sites in the rat. Gastroenterology. 1995. 109:718–722.
5. Lin A, Lourenssen S, Stanzel RD, Blennerhassett MG. Selective loss of NGF-sensitive neurons following experimental colitis. Exp Neurol. 2005. 191:337–343.
6. Lu G, Qian X, Berezin I, Telford GL, Huizinga JD, Sarna SK. Inflammation modulates in vitro colonic myoelectric and contractile activity and interstitial cells of Cajal. Am J Physiol. 1997. 273:G1233–G1245.
7. Chang IY, Glasgow NJ, Takayama I, Horiguchi K, Sanders KM, Ward SM. Loss of interstitial cells of Cajal and development of electrical dysfunction in murine small bowel obstruction. J Physiol. 2001. 536:555–568.
8. Blennerhassett MG, Vignjevic P, Vermillion DL, Collins SM. Inflammation causes hyperplasia and hypertrophy in smooth muscle of rat small intestine. Am J Physiol. 1992. 262:G1041–G1046.
9. Malykhina AP, Akbarali HI. Inflammation-induced "channelopathies" in the gastrointestinal smooth muscle. Cell Biochem Biophys. 2004. 41:319–330.
10. Akbarali HI, Pothoulakis C, Castagliuolo I. Altered ion channel activity in murine colonic smooth muscle myocytes in an experimental colitis model. Biochem Biophys Res Commun. 2000. 275:637–642.
11. Shi XZ, Sarna SK. Impairment of Ca2+ mobilization in circular muscle cells of the inflamed colon. Am J Physiol Gastrointest Liver Physiol. 2000. 278:G234–G242.
12. Kinoshita K, Sato K, Hori M, Ozaki H, Karaki H. Decrease in activity of smooth muscle L-type Ca2+ channels and its reversal by NF-kappaB inhibitors in Crohn's colitis model. Am J Physiol Gastrointest Liver Physiol. 2003. 285:G483–G493.
13. Kang M, Morsy N, Jin X, Lupu F, Akbarali HI. Protein and gene expression of Ca2+ channel isoforms in murine colon: effect of inflammation. Pflugers Arch. 2004. 449:288–297.
14. Jin X, Malykhina AP, Lupu F, Akbarali HI. Altered gene expression and increased bursting activity of colonic smooth muscle ATP-sensitive K+ channels in experimental colitis. Am J Physiol Gastrointest Liver Physiol. 2004. 287:G274–G285.
15. Sawyer GW, Ehlert FJ. Muscarinic M3 receptor inactivation reveals a pertussis toxin-sensitive contractile response in the guinea pig colon: evidence for M2/M3 receptor interactions. J Pharmacol Exp Ther. 1999. 289:464–476.
16. Shi XZ, Sarna SK. Inflammatory modulation of muscarinic receptor activation in canine ileal circular muscle cells. Gastroenterology. 1997. 112:864–874.
17. Ehlert FJ, Sawyer GW, Esqueda EE. Contractile role of M2 and M3 muscarinic receptors in gastrointestinal smooth muscle. Life Sci. 1999. 64:387–394.
18. Hamill OP, Marty A, Neher E, Sakmann B, Sigworth FJ. Improved patch-clamp techniques for high-resolution current recording from cells and cell-free membrane patches. Pflugers Arch. 1981. 391:85–100.
19. Livak KJ, Schmittgen TD. Analysis of relative gene expression data using real-time quantitative PCR and the 2(-Delta Delta C(T)) Method. Methods. 2001. 25:402–408.
20. Snape WJ Jr, Matarazzo SA, Cohen S. Abnormal gastrocolonic response in patients with ulcerative colitis. Gut. 1980. 21:392–396.
21. Vermillion DL, Huizinga JD, Riddell RH, Collins SM. Altered small intestinal smooth muscle function in Crohn's disease. Gastroenterology. 1993. 104:1692–1699.
22. Hibi T, Ogata H, Sakuraba A. Animal models of inflammatory bowel disease. J Gastroenterol. 2002. 37:409–417.
23. Morris GP, Beck PL, Herridge MS, Depew WT, Szewczuk MR, Wallace JL. Hapten-induced model of chronic inflammation and ulceration in the rat colon. Gastroenterology. 1989. 96:795–803.
24. Boirivant M, Fuss IJ, Chu A, Strober W. Oxazolone colitis: A murine model of T helper cell type 2 colitis treatable with antibodies to interleukin 4. J Exp Med. 1998. 188:1929–1939.
25. Okayasu I, Hatakeyama S, Yamada M, Ohkusa T, Inagaki Y, Nakaya R. A novel method in the induction of reliable experimental acute and chronic ulcerative colitis in mice. Gastroenterology. 1990. 98:694–702.
26. Marcus AJ, Marcus SN, Marcus R, Watt J. Rapid production of ulcerative disease of the colon in newly-weaned guinea-pigs by degraded carrageenan. J Pharm Pharmacol. 1989. 41:423–426.
27. Kitsukawa Y, Saito H, Suzuki Y, Kasanuki J, Tamura Y, Yoshida S. Effect of ingestion of eicosapentaenoic acid ethyl ester on carrageenan-induced colitis in guinea pigs. Gastroenterology. 1992. 102:1859–1866.
28. Jurjus AR, Khoury NN, Reimund JM. Animal models of inflammatory bowel disease. J Pharmacol Toxicol Methods. 2004. 50:81–92.
29. Yamada Y, Marshall S, Specian RD, Grisham MB. A comparative analysis of two models of colitis in rats. Gastroenterology. 1992. 102:1524–1534.
30. Elson CO, Sartor RB, Tennyson GS, Riddell RH. Experimental models of inflammatory bowel disease. Gastroenterology. 1995. 109:1344–1367.
31. Depoortere I, Van Assche G, Thijs T, Geboes K, Peeters TL. Differential changes in ACh-, motilin-, substance P-, and K+-induced contractility in rabbit colitis. Am J Physiol. 1999. 277:G61–G68.
32. Kwon SC, Won KJ, Jung SH, Lee KP, Lee DY, Park ES, Kim B, Cheon GJ, Han KH. Proteomic analysis of colonic mucosal tissue from tuberculous and ulcerative colitis patients. Korean J Physiol Pharmacol. 2012. 16:193–198.
33. Sarna SK. Neuronal locus and cellular signaling for stimulation of ileal giant migrating and phasic contractions. Am J Physiol Gastrointest Liver Physiol. 2003. 284:G789–G797.
34. Kinoshita K, Horiguchi K, Fujisawa M, Kobirumaki F, Yamato S, Hori M, Ozaki H. Possible involvement of muscularis resident macrophages in impairment of interstitial cells of Cajal and myenteric nerve systems in rat models of TNBS-induced colitis. Histochem Cell Biol. 2007. 127:41–53.
35. Sanders KM. A case for interstitial cells of Cajal as pacemakers and mediators of neurotransmission in the gastrointestinal tract. Gastroenterology. 1996. 111:492–515.
36. Thuneberg L. Interstitial cells of Cajal: intestinal pacemaker cells? Adv Anat Embryol Cell Biol. 1982. 71:1–130.
37. Faussone-Pellegrini MS, Thuneberg L. Guide to the identification of interstitial cells of Cajal. Microsc Res Tech. 1999. 47:248–266.
38. Rumessen JJ. Identification of interstitial cells of Cajal. Significance for studies of human small intestine and colon. Dan Med Bull. 1994. 41:275–293.
39. Porcher C, Baldo M, Henry M, Orsoni P, Julé Y, Ward SM. Deficiency of interstitial cells of Cajal in the small intestine of patients with Crohn's disease. Am J Gastroenterol. 2002. 97:118–125.
40. Liu X, Rusch NJ, Striessnig J, Sarna SK. Down-regulation of L-type calcium channels in inflamed circular smooth muscle cells of the canine colon. Gastroenterology. 2001. 120:480–489.
41. Kinoshita K, Sato K, Hori M, Ozaki H, Karaki H. Decrease in activity of smooth muscle L-type Ca2+ channels and its reversal by NF-kappaB inhibitors in Crohn's colitis model. Am J Physiol Gastrointest Liver Physiol. 2003. 285:G483–G493.
42. Ohama T, Hori M, Ozaki H. Mechanism of abnormal intestinal motility in inflammatory bowel disease: how smooth muscle contraction is reduced? J Smooth Muscle Res. 2007. 43:43–54.
43. Shi XZ, Pazdrak K, Saada N, Dai B, Palade P, Sarna SK. Negative transcriptional regulation of human colonic smooth muscle Cav1.2 channels by p50 and p65 subunits of nuclear factor-kappaB. Gastroenterology. 2005. 129:1518–1532.
44. Wegener JW, Schulla V, Koller A, Klugbauer N, Feil R, Hofmann F. Control of intestinal motility by the Ca(v)1.2 L-type calcium channel in mice. FASEB J. 2006. 20:1260–1262.
45. Stengel PW, Gomeza J, Wess J, Cohen ML. M2 and M4 receptor knockout mice: muscarinic receptor function in cardiac and smooth muscle in vitro. J Pharmacol Exp Ther. 2000. 292:877–885.
46. Matsui M, Motomura D, Fujikawa T, Jiang J, Takahashi S, Manabe T, Taketo MM. Mice lacking M2 and M3 muscarinic acetylcholine receptors are devoid of cholinergic smooth muscle contractions but still viable. J Neurosci. 2002. 22:10627–10632.
47. Preiksaitis HG, Krysiak PS, Chrones T, Rajgopal V, Laurier LG. Pharmacological and molecular characterization of muscarinic receptor subtypes in human esophageal smooth muscle. J Pharmacol Exp Ther. 2000. 295:879–888.
48. Jin X, Morsy N, Shoeb F, Zavzavadjian J, Akbarali HI. Coupling of M2 muscarinic receptor to L-type Ca channel via c-src kinase in rabbit colonic circular smooth muscle. Gastroenterology. 2002. 123:827–834.
49. Shi XZ, Sarna SK. Differential inflammatory modulation of canine ileal longitudinal and circular muscle cells. Am J Physiol. 1999. 277:G341–G350.
50. Jadcherla SR. Inflammation inhibits muscarinic signaling in in vivo canine colonic circular smooth muscle cells. Pediatr Res. 2002. 52:756–762.
51. Bolton TB. Cholinergic mechanisms in smooth muscle. Br Med Bull. 1979. 35:275–283.
52. Benham CD, Bolton TB, Lang RJ. Acetylcholine activates an inward current in single mammalian smooth muscle cells. Nature. 1985. 316:345–347.
53. Inoue R, Isenberg G. Acetylcholine activates nonselective cation channels in guinea pig ileum through a G protein. Am J Physiol. 1990. 258:C1173–C1178.
54. Zhu MH, Lee YM, Jin N, So I, Kim KW. The transient receptor potential protein homologue TRPC4/5 as a candidate for the nonselective cationic channel activated by muscarinic stimulation in the murine stomach. Neurophysiol. 2003. 35:302–307.
55. Venkatachalam K, van Rossum DB, Patterson RL, Ma HT, Gill DL. The cellular and molecular basis of store-operated calcium entry. Nat Cell Biol. 2002. 4:E263–E272.
56. Freichel M, Vennekens R, Olausson J, Hoffmann M, Müller C, Stolz S, Scheunemann J, Weissgerber P, Flockerzi V. Functional role of TRPC proteins in vivo: lessons from TRPC-deficient mouse models. Biochem Biophys Res Commun. 2004. 322:1352–1358.
57. Clapham DE. TRP channels as cellular sensors. Nature. 2003. 426:517–524.