Abstract
A previous animal study has shown the effects of erythropoietin (EPO) and its non-erythropoietic carbamylated derivative (CEPO) on neurogenesis in the dentate gyrus. In the present study, we sought to investigate the effect of EPO on adult hippocampal neurogenesis, and to compare the ability of EPO and CEPO promoting dendrite elongation in cultured hippocampal neural progenitor cells. Two-month-old male BALB/c mice were given daily injections of EPO (5 U/g) for seven days and were sacrificed 12 hours after the final injection. Proliferation assays demonstrated that EPO treatment increased the density of bromodeoxyuridine (BrdU)-labeled cells in the subgranular zone (SGZ) compared to that in vehicle-treated controls. Functional differentiation studies using dissociated hippocampal cultures revealed that EPO treatment also increased the number of double-labeled BrdU/microtubule-associated protein 2 (MAP2) neurons compared to those in vehicle-treated controls. Both EPO and CEPO treatment significantly increased the length of neurites and spine density in MAP2(+) cells. In summary, these results provide evidences that EPO and CEPO promote adult hippocampal neurogenesis and neuronal differentiation. These suggest that EPO and CEPO could be a good candidate for treating neuropsychiatric disorders such as depression and anxiety associated with neuronal atrophy and reduced hippocampal neurogenesis.
The glycoprotein hormone erythropoietin (EPO) was originally identified as a hematopoietic growth factor [1] that crosses the blood-brain barrier to stimulate neurogenesis and activate brain neurotrophic, anti-apoptotic, anti-oxidant and anti-inflammatory signaling. These mechanisms underlie neuroprotective effects in nervous system disorders [2]. Carbamylated EPO (CEPO) is an EPO derivative without hematopoietic bioactivity and that does not bind to homodimeric EPO receptors [3]. However, it has been shown to induce neurogenesis and neuroprotection in various neurodegenerative models [4].
The association of EPO and its derivative with psychiatric diseases is a relatively new concept. In particular, adult hippocampal neurogenesis could be involved in the pathophysiology of affective disorders such as depression and anxiety [5]. EPO has interesting properties, which make it a candidate for investigation as a new therapeutic agent in psychiatric diseases [6]. However, EPO may be associated with increased thrombotic risk, whereas CEPO may be devoid of such side-effects [7].
Recent animal study showed that both EPO and its derivate CEPO treatment enhanced spatial and non-spatial recognition memory in adult healthy mice and increased the number of Neuronal Nuclei (NeuN)/BrdU double-labeled cells in the dentate gyrus [8]. Moreover, CEPO treatment produces antidepressant-like effects in comparison to vehicle- and EPO-treated animals in the tail suspension test [8]. Previous study using hippocampal slice cultures also demonstrated the neuroprotective effects of EPO and CEPO against oxygen-glucose deprivation and N-methyl-D-aspartate (NMDA) excitotoxicity [9]. It was recently reported that EPO promotes axonal and dendritic growth in hippocampal neuronal cultures (stained with Tau-1/MAP2) [10]. Previous in vitro results using neurosphere culture demonstrated that CEPO promotes neurite outgrowth, which was quantified by measuring the length and number of branches extending from soma of class III β-tubulin (Tuj1(+)) cells [11]. However, there have been no studies to compare the effects of EPO and CEPO on neurite outgrowth.
The aim of this study was to show the effect of EPO on adult hippocampal neurogenesis, and to compare the ability of EPO and CEPO promoting dendrite elongation in cultured hippocampal neural progenitor cells. For this, we investigated whether exogenous recombinant human EPO increased the number of BrdU(+) cells in the hippocampus of mice and the percentage of double-labeled BrdU/MAP2 in progenitor cell cultures. We demonstrated that EPO increased hippocampal neurogenesis in response to EPO and CEPO stimulation.
Two-month-old male BALB/c mice (BALB/cAnNHsd) were housed in a 12-hour light/dark cycle animal facility and intraperitoneally injected daily with saline or EPO (5 U/g) for seven days. The mice also received single daily intraperitoneal injections of BrdU (100 mg/kg of body weight at 30 mg/ml) for the final three days of EPO treatment. Animals were sacrificed under pentobarbital anesthesia 12 hours after the administration of BrdU. The mice were processed, and BrdU was analyzed histochemically as described previously [12]; BrdU(+) cells in the SGZ were counted. Cells were mounted in mounting medium using Vectashield® with 4', 6 diamidino-2-phenylindole (DAPI) (Vector Laboratories, Burlingame, CA). Statistical significance was determined using Student's t-test in Sigma Plot 2,000 software. All animal experiments were approved by the Institutional Animal Care and Use Committee of Hanyang University and were performed in accordance with relevant guidelines and regulations.
We used pregnant adult Sprague Dawley rats (Harlan Sprague Dawley, Indianapolis, IN, USA) and previously described conditions [13-15] with slight modifications. Briefly, hippocampi from embryonic day 16.5 embryos were dissected into calcium- and magnesium-free Hank's balanced salt solution (HBSS). The dissociated cells were plated onto 10-cm-diameter dishes coated with 15 µg/ml poly-L-ornithine and 1 µg/ml fibronectin (Invitrogen, Carlsbad, CA) at 2.5×104 cells/cm2 in N2 medium and were incubated at 37℃ in 95% air/5% CO2. Basic fibroblast growth factor (bFGF, 20 ng/ml, R&D Systems, Minneapolis, MN) was added daily for 2~3 days to increase the population of prolif erative progenitors, and the medium was changed at each bFGF addition. For high-density cultures, cells at 80% confluence were subcultured in N2 medium in the presence of 6×104 cells/cm2 bFGF in 24 well plates, and these subcultured cells were designated as "passaged once (P1)". All experiments were carried out using P1 neural progenitors, which were induced to differentiate via bFGF withdrawal and were maintained in differentiation medium (neurobasal medium) for 5 days with or without EPO and CEPO.
Cells were fixed in 4% paraformaldehyde/0.15% picric acid in PBS for 20 minutes. The primary antibodies monoclonal monoclonal MAP2 at 1:200 (Sigma-Aldrich, St. Louis, MO) and rat anti-BrdU at 1:400 (Accurate Chemical, Westbury, NY, USA) were used for neuronal differentiation and BrdU staining, respectively. For detection of primary antibodies, cells were incubated in PBS containing Cy3- (Jackson ImmunoResearch; 1:100), Cy2- (Jackson ImmunoResearch; 1:200) or FITC-labeled (Molecular Probes; 1:500) secondary antibodies for 1 hour at room temperature. Cells were then mounted in Vectashield mounting medium and photographed under a fluorescence microscope (Nikon). To evaluate neurite length, MAP2(+) neurons were photographed at 200× magnification. Processes were traced, and the lengths of neurites exiting the soma (1' dendrite) were measured (n=40 cells per treatment). For statistical analyses, at least ten random fields were counted for each condition. The results are presented as mean±S.E.M. of three independent experiments.
EPO was carbamylated as described by [16] via incubation at 37℃ with 2 M cyanate for either 1, 2, 3, 4, 5 or 6 hours. Carbamylated EPO was then used for dialysis. EPO was also carbamylated via incubation at 37℃ with 8, 16, 125, 1,000 or 2,000 mM cyanate for 6 hours. The CEPO was then subjected to extensive dialysis against a total of 12 liters of phosphate buffered saline at pH 7.4 at 4℃ to remove excess cyanate. An amount of CEPO corresponding to 10 to 20 U of EPO was then applied to the cultures.
Images were acquired through Z-stacks, which typically consisted of 10 scans at high zoom at 1-µm steps in the z axis. Each MAP2(+) neuron was clearly distinguishable from other cells. For analysis of spine density, we focused on first-order dendrite from cells. For each cell, 3 dendritic segments were used for spine analysis. Spine counting was conducted with a 60× objective using the DeltaVision Images (Applied Precision, Seattle, USA). The number of spines was counted in a 10 µm segment. The final value was averaged from six rats per group and expressed as the number of spines/10 µm. For analysis of dendritic arborization, the Z-trace feature was used to measure the three-dimensional length of the dendritic arbor, from three to five cells per rat. Within each cell, first-order dendrites arising from the cell body were separately traced.
The effect of repeated administration of EPO (5 U/g, once daily for seven days) on hippocampal neurogenesis in the dentate gyrus was first examined at the level of cell proliferation. To achieve this, we processed tissue for BrdU (a marker of cell proliferation) immunohistochemistry 12 hours after the completion of treatment. We observed dense staining of BrdU(+) cells in the SGZ of both groups (EPO and vehicle). However, immunohistochemical analysis revealed that EPO produced a significant 122.3±7.9% increase in BrdU(+) cells in the SGZ compared to the number in the vehicle-treated controls (Fig. 1; p<0.05). These results indicate that EPO increases the proliferation of progenitor cells in the adult hippocampus.
In order to determine whether EPO affects cell differentiation in cultured hippocampal progenitor cells, passaged cells were allowed to differentiate in neurobasal medium for five days, followed by dual immunocytochemistry with anti-BrdU/ant-MAP2 (Fig. 2A). EPO significantly increased the fraction of BrdU(+) cells colabeled with MAP2 (neuronal marker) compared with the level observed in the control groups. In particular, the fraction of BrdU(+) cells was significantly increased in a dose-dependent manner, to a maximum of 22.1±1.7% at a dose of 10 U, compared with 14.1±2.3% in control cultures (n=3, p<0.01) and 19.2±0.8% at a dose of 5 U (n=3, p<0.05) (Fig. 2B, C). These results indicate that sub-chronic EPO treatment enhances neuronal differentiation in hippocampal progenitor cells.
To examine whether EPO and CEPO enhance neurite outgrowth, neurite outgrowth was quantified by measuring the lengths of branches extending from MAP2(+) cell soma. In the control group, MAP2(+) cells exhibited a few short branches. In contrast, MAP2(+) cells exhibited complex branching patterns when neural progenitor cells were incubated with EPO or CEPO. In addition, the dendritic lengths of MAP2(+) neurons were increased by EPO and CEPO treatments (Fig. 3). Quantitative analysis revealed that treatment with EPO and CEPO significantly increased the lengths of dendrites compared to those in the vehicle-treated controls (in µm; CTL: 65.4±6.2; EPO: 10 U, 90.1±3.9, 20 U, 95.8±1.1, p<0.05; CEPO: 10 U, 101.7±1.7, 20 U, 103.6±1.2, p<0.01). These results indicate that EPO and CEPO promote neurite outgrowth. However, there was no difference in neurite outgrowth between EPO and CEPO treatments at either dose level.
To test if EPO and CEPO can also promote the spine formation, we examined spine number in cells treated with EPO or CEPO. Treatment cells with EPO or CEPO significantly increased the number of spine in MAP2(+) cells (Fig. 4). Together, the results demonstrate that EPO and CEPO increase spine formation in addition to neuritie outgrowth.
We demonstrated that EPO treatment facilitates in vivo proliferation and in vitro differentiation of hippocampal neural progenitor cells under basal conditions. These results confirm prior findings [8,16] that EPO treatment increases neurogenesis in the hippocampus. Ransome and Turnley [17] used two-month-old female C57BL/6 mice to demonstrate that seven-day systemic treatment with recombinant human EPO (5 U/g) increased the number of BrdU(+) cells in the SGZ by 30% compared to those in vehicle-treated controls [17]. Leconte et al. [8] used adult male Swiss mice (aged ten weeks) to show that both EPO and CEPO (40 mg/kg, twice a week) administered over a six-week period increased the number of NeuN/BrdU double-labeled cells in the dentate gyrus [8].
We further demonstrated that both EPO and CEPO treatments increased the lengths of neurites in adult hippocampal neural progenitor cell cultures when continuously applied for five days during differentiation. This result is consistent with prior studies showing that EPO can enhance neurite outgrowth [10,18]. In particular, a recent study showed that EPO differentially stimulated neurite outgrowth in polarizing hippocampal neurons based on the timing of administration with respect to the stage of neuronal polarization. It also provided evidence that EPO can promote axonal growth and branching through activation of the PI-3 kinase/Akt pathway in polarizing hippocampal neurons [10]. In this study, we showed for the first time that CEPO promotes neurite outgrowth at least as efficiently as does EPO in adult hippocampal neural progenitor cell cultures under basal conditions.
The neurogenesis effects of EPO appear to be mediated by various mechanisms. Endogenous and exogenous EPO can bind and stimulate the EPO receptor to induce phosphorylation of JAK2 [19]. Activated JAK2 induces various signaling pathways through several adaptor proteins such as phosphoinositol 3-kinase (PI3-K), signal transducer and activator of transcription 5 (STAT5) and Nuclear factor kappa B (NF-κB) [20]. All of these signaling pathways have been known to affect the gene transcription in neuronal survival related to EPO.
Unlike EPO, the cellular mechanisms responsible for the neurogenic effects of CEPO have not been fully elucidated. CEPO does not bind the EPO receptor but may exert neurogenesis effects via alternative binding sites [4]. Previous study using neural progenitor cells derived from the SVZ of the adult mouse indicate that CEPO enhances in vitro neurogenesis which is mediated by the Sonic hedgehog (Shh) signaling pathway [21].
In summary, the results of present studies provide evidences that EPO and CEPO promote adult hippocampal neurogenesis. Adult hippocampal neurogenesis has been known to plays an important role in learning and memory processes [22] and mood regulation such as depression and anxiety [5]. Although, to date, there has been no clinical trial demonstrating EPO or CEPO treatment is effective in patients with major depressive disorder. Miskowiak et al. have recently reported cognitive and neural effects of a single dose of EPO (40,000 U; i.v.) in human in a manner independent of any hematological effects in healthy volunteers [22-25]. Although further studies are required to clarify the mechanisms underlying the neurogenesis and neuroprotective effects of CEPO, EPO and CEPO could be a good candidate for developing new neuroprotective strategies [23-25]. Additional studies are warranted to investigate whether the reduced hippocampal neurogenesis associated with neuropsychiatric disorders such as depression and anxiety may benefit from CEPO treatment.
Figures and Tables
Fig. 1
Effect of erythropoietin (EPO) administration on cell proliferation in the dentate gyrus of adult mice. (A) BrdU labeling was used to assess cell proliferation. BrdU(+) cells were observed in the SGZ of the dentate gyrus (DG). (B) Stereological three-dimensional counts revealed that EPO treatment produced a significant increase (saline, 3,440±175 cells/mm3 vs. EPO, 4,228±286 cells/mm3, n=4 animals in each group) in BrdU(+) cells compared with those in saline treatment (control group, CTL) when analyzed 12 hours after treatment completion. *p<0.05. Scale bar: 200 µm.
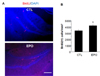
Fig. 2
Effects of EPO treatment on differentiation of cultured adult hippocampal progenitor cells. (A) Cells were prepared as described in Methods. (B) Representative microscopic images showing that BrdU(+) cells differentiated into neuronal cells (MAP2(+) in green) (arrows) in the presence of EPO (10 U). (C) Quantitative analysis of BrdU/MAP2 doubly-labeled cells among total BrdU(+) cells. The results indicate that the percentage of BrdU(+) cells labeled for MAP2 was significantly increased by EPO treatment compared to that in the control group (CTL). *p<0.05, **p<0.01. Scale bar: 200 µm.
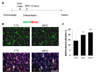
Fig. 3
EPO and CEPO increase the lengths of neurites. The lengths of neurites extending from the cell soma (arrows) were counted in neurons immunostained for MAP2 (n=40 cells per treatment). (A) Cells were prepared as described in Methods. (B, C) Treatment with EPO and EPO significantly (p<0.05) increased neurite outgrowth compared with that in the control group (CTL). *p<0.05, **p<0.01. Scale bar: 50 µm.
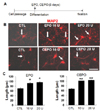
Fig. 4
EPO and CEPO increase the spine density. (A) Representative images of high-magnification Z-stack projections of dendrites of MAP2(+) hippocampal cells. (B) The density of dendritic spines (arrows) was significantly increased by EPO and CEPO (***p<0.001). n=9-15 MAP2(+) neurons per each group. The data were expressed as the number of spines per 10 µm. Scale bar: 10 µm.
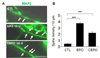
ACKNOWLEDGEMENTS
This research was supported by a grant from the Korea Health Industry Development Institute (A090168) awarded to H. Son.
References
1. Jelkmann W. Developments in the therapeutic use of erythropoiesis stimulating agents. Br J Haematol. 2008. 141:287–297.
2. Sirén AL, Fasshauer T, Bartels C, Ehrenreich H. Therapeutic potential of erythropoietin and its structural or functional variants in the nervous system. Neurotherapeutics. 2009. 6:108–127.
3. Leist M, Ghezzi P, Grasso G, Bianchi R, Villa P, Fratelli M, Savino C, Bianchi M, Nielsen J, Gerwien J, Kallunki P, Larsen AK, Helboe L, Christensen S, Pedersen LO, Nielsen M, Torup L, Sager T, Sfacteria A, Erbayraktar S, Erbayraktar Z, Gokmen N, Yilmaz O, Cerami-hand C, Xie QW, Coleman T, Cerami A, Brines M. Derivatives of erythropoietin that are tissue protective but not erythropoietic. Science. 2004. 305:239–242.
4. Xiong Y, Mahmood A, Zhang Y, Meng Y, Zhang ZG, Qu C, Sager TN, Chopp M. Effects of posttraumatic carbamylated erythropoietin therapy on reducing lesion volume and hippocampal cell loss, enhancing angiogenesis and neurogenesis, and improving functional outcome in rats following traumatic brain injury. J Neurosurg. 2011. 114:549–559.
5. David DJ, Wang J, Samuels BA, Rainer Q, David I, Gardier AM, Hen R. Implications of the functional integration of adult-born hippocampal neurons in anxiety-depression disorders. Neuroscientist. 2010. 16:578–591.
6. Miskowiak KW, Vinberg M, Harmer CJ, Ehrenreich H, Knudsen GM, Macoveanu J, Hansen AR, Paulson OB, Siebner HR, Kessing LV. Effects of erythropoietin on depressive symptoms and neurocognitive deficits in depression and bipolar disorder. Trials. 2010. 11:97.
7. Kirkeby A, Torup L, Bochsen L, Kjalke M, Abel K, Theilgaardmonch K, Johansson PI, Bjørn SE, Gerwien J, Leist M. High-dose erythropoietin alters platelet reactivity and bleeding time in rodents in contrast to the neuroprotective variant carbamyl-erythropoietin (CEPO). Thromb Haemost. 2008. 99:720–728.
8. Leconte C, Bihel E, Lepelletier FX, Bouët V, Saulnier R, Petit E, Boulouard M, Bernaudin M, Schumann-bard P. Comparison of the effects of erythropoietin and its carbamylated derivative on behaviour and hippocampal neurogenesis in mice. Neuropharmacology. 2011. 60:354–364.
9. Montero M, Poulsen FR, Noraberg J, Kirkeby A, Van BJ, Leist M, Zimmer J. Comparison of neuroprotective effects of erythropoietin (EPO) and carbamylerythropoietin (CEPO) against ischemia-like oxygen-glucose deprivation (OGD) and NMDA excitotoxicity in mouse hippocampal slice cultures. Exp Neurol. 2007. 204:106–117.
10. Ransome MI, Turnley AM. Erythropoietin promotes axonal growth in a model of neuronal polarization. Mol Cell Neurosci. 2008. 38:537–547.
11. Wang L, Zhang ZG, Gregg SR, Zhang RL, Jiao Z, Letourneau Y, Liu X, Feng Y, Gerwien J, Torup L, Leist M, Noguchi CT, Chen ZY, Chopp M. The Sonic hedgehog pathway mediates carbamylated erythropoietin-enhanced proliferation and differentiation of adult neural progenitor cells. J Biol Chem. 2007. 282:32462–32470.
12. Son H, Yu IT, Hwang SJ, Kim JS, Lee SH, Lee YS, Kaang BK. Lithium enhances long-term potentiation independently of hippocampal neurogenesis in the rat dentate gyrus. J Neurochem. 2003. 85:872–881.
13. Amoureux MC, Cunningham BA, Edelman GM, Crossin KL. N-CAM binding inhibits the proliferation of hippocampal progenitor cells and promotes their differentiation to a neuronal phenotype. J Neurosci. 2000. 20:3631–3640.
14. Johe KK, Hazel TG, Muller T, Dugich-djordjevic MM, Mckay RD. Single factors direct the differentiation of stem cells from the fetal and adult central nervous system. Genes Dev. 1996. 10:3129–3140.
15. Kim JS, Chang MY, Yu IT, Kim JH, Lee SH, Lee YS, Son H. Lithium selectively increases neuronal differentiation of hippocampal neural progenitor cells both in vitro and in vivo. J Neurochem. 2004. 89:324–336.
16. Hörkkö S, Savolainen MJ, Kervinen K, Kesäniemi YA. Carbamylation-induced alterations in low-density lipoprotein metabolism. Kidney Int. 1992. 41:1175–1181.
17. Ransome MI, Turnley AM. Systemically delivered Erythropoietin transiently enhances adult hippocampal neurogenesis. J Neurochem. 2007. 102:1953–1965.
18. Kretz A, Happold CJ, Marticke JK, Isenmann S. Erythropoietin promotes regeneration of adult CNS neurons via Jak2/Stat3 and PI3K/AKT pathway activation. Mol Cell Neurosci. 2005. 29:569–579.
19. Noguchi CT, Asavaritikrai P, Teng R, Jia Y. Role of erythropoietin in the brain. Crit Rev Oncol Hematol. 2007. 64:159–171.
20. van der Kooij MA, Groenendaal F, Kavelaars A, Heijnen CJ, Van BF. Neuroprotective properties and mechanisms of erythropoietin in in vitro and in vivo experimental models for hypoxia/ischemia. Brain Res Rev. 2008. 59:22–33.
21. Wang L, Zhang ZG, Gregg SR, Zhang RL, Jiao Z, Letourneau Y, Liu X, Feng Y, Gerwien J, Torup L, Leist M, Noguchi CT, Chen ZY, Chopp M. The Sonic hedgehog pathway mediates carbamylated erythropoietin-enhanced proliferation and differentiation of adult neural progenitor cells. J Biol Chem. 2007. 282:32462–32470.
22. Deng W, Aimone JB, Gage FH. New neurons and new memories: how does adult hippocampal neurogenesis affect learning and memory? Nat Rev Neurosci. 2010. 11:339–350.
23. Lapchak PA. Carbamylated erythropoietin to treat neuronal injury: new development strategies. Expert Opin Investig Drugs. 2008. 17:1175–1186.
24. Kim SS, Lee KH, Sung DK, Shim JW, Kim MJ, Jeon GW, Chang YS, Park WS. Erythropoietin attenuates brain injury, subventricular zone expansion, and sensorimotor deficits in hypoxic-ischemic neonatal rats. J Korean Med Sci. 2008. 23:484–491.
25. Ehrenreich H, Degner D, Meller J, Brines M, Béhé M, Hasselblatt M, Woldt H, Falkai P, Knerlich F, Jacob S, Von AN, Maier W, Brück W, Rüther E, Cerami A, Becker W, Sirén AL. Erythropoietin: a candidate compound for neuroprotection in schizophrenia. Mol Psychiatry. 2004. 9:42–54.