Abstract
Recent studies suggest that immunization with autologous dendritic cells (DCs) results in protective immunity and rejection of established tumors in various human malignancies. The purpose of this study is to determine whether DCs are generated from peripheral blood mononuclear cells (PBMNs) by using cytokines such as F1t-3 ligand (FL), granulocyte macrophage-colony stimulating factor (GM-CSF), IL-4, and TNF-α, and whether cytotoxic T cells activated against the thyroid cancer tissues by the DCs. Peripheral blood was obtained from 2 patients with thyroid cancer. DCs were established from PBMNs by culturing in the presence of FL, GM-CSF, IL-4, and TNF-α for 14 days. At day 14, the differentiated DCs was analyzed morphologically. The immunophenotypic features of DCs such as CDla, CD83, and CD86 were analyzed by immunofluorelescence microscopy. At day 18, DCs and T cells were incubated with thyroid cancer tissues or normal thyroid tissues for additional 4 days, respectively. DCs generated from the PBMNs showed the typical morphology of DCs. Activated cytotoxic T lymphocytes (CTLs) were observed also. DCs and the CTLs were attached to the cancer tissues on scanning electron microscope. The DCs activated the CTLs, which able to specifically attack the thyroid cancer. This study provides morphologic evidence that the coculture of T cells/cancer tissues activated the T cells and differentiated CTLs. The CTLs tightly adhered to cancer tissues and lysed cancer tissues vigorously. Therefore DCs could be used as potential vaccines in the immunotherapy.
Dendritic cells (DCs) are the most potent antigen presenting cells and are unique in their ability to stimulate T cells and initiate adaptive immunity. Recently, progress in the understanding of DCs biology have been relatively slow and difficult because of the paucity of these cells in blood and other tissues [1]. A major advance has been made by the establishment of ex vivo culture systems, which permits the induction of DCs from precursors [2]. Despite this progress the total number of DCs available for immunotherapy is still limited [3-6].
There has long been the goal of cancer researchers to heighten the patient's own immune response to their cancer, but to date the means to do this have remained elusive. Immunological elimination of tumors occurs primarily from the recognition of tumor antigens by CTLs capable of lysing cells that express that antigen. The signals that lead to T-cell activation are generated at the level of the immunological synapse, a specialized area of contact between T cells and antigen presenting cells (APCs) where adhesion molecules and T-cell receptors (TCRs) segregate into distinct supramolecular complexes [7,8]. At the synapse, the TCRs are sequentially triggered by peptide-MHC complexes, a process that allows the signal to be sustained for as long as the synapse is in place [9,10].
The current promising strategies seek to produce active immunity through the use of DCs [11-17]. They present not only an array of antigenic peptides needed to activate the appropriate antigen-specific T cells, but also produce potent costimulatory signals that drive quiescent T cells into the cell cycle and along the differentiation pathway, producing an expansion of effector cells [18].
The author therefore hypothesized that the autologous DCs derived from PBMNs of thyroid cancer patients will present altered cancer antigen to T cells and the activated CTLs lyse only thyroid cancer tissues but not normal tissues. To test this hypothesis, The author established a PBMNs/lymphocytes coculture system that allowed me to demonstrate the influence of these cytokines and to elucidate the role of DCs in T-cells activation. Furthermore, The author obtained the scanning electon microscope that activated CTLs adhered and destoryed the cancer tissues, however, normal tissues were not attacted by the T cells.
Histopaque-1077 and trypsin-EDTA were purchased from Sigma Chemical Co. (St. Louis, MO, USA), X-VIVO 20 medium was purchased from Bio Whittaker (Walkersville, MD, USA). Mouse human-anti CD1a, CD3, CD4, CD8, CD56, CD83 and CD86 antibodies were purchased from Serotec (Raleigh, NC USA). Flt3-ligand (FL), GM-CSF (CSF2), IL-4 and TNF-α were purchased from R&D systems (Minneapolis, MN, USA). Plastic tissue culture flasks were purchased from Corning (Corning, NY, USA). All other chemicals used in this study were the highest grade available.
PBMNs were centrifuged by density gradient method using Ficoll-Hypaque (Histopaque). The leukocytes were washed twice with phosphate buffered saline (PBS), and resuspended in medium X-VIVO 20 for liquid culture at 37℃ in plastic tissue culture flasks.
The purified PBMNs were adjusted to 1.5×105 cells/ml in X-VIVO 20 medium supplemented with 50 ng/ml of FL, 50 ng/ml of GM-CSF, 25 ng/ml of IL-4 and 10 ng/ml of TNF-α. The cells were incubated in 75 cm2 flasks in a humidified atmosphere of 5% CO2 at 37℃ [19].
On 5, 8, and 14 days of culture, total cell counts and viability were assessed by hemocytometer counts of dilutions prepared in trypan blue solution. DCs were scored as the cells with veils and long projection cell bodies.
At day 15, DCs were incubated with T cells and lysates of the cancer tissues or normal tissues, respectively. At day 18, intact cancer tissues were incubated with the autologous activated T cells for additional 4 days. The morphology of the cancer tissues and T cells were examined by scanning electron microscopy.
For the examination of tissue samples by light microscopy, the tissues obtained from thyroid cancer patients were fixed with 10% formaldehyde and embedded in parpaffin. The thin sections were dried, deparpffinized, and dehydrated in a graded alcohol series. The samples were stained with Hematoxylin-Eosin.
The generated DCs were stained with fluorescein isothiocyanate (FITC)-conjugated murine monoclonal antibodies for 15 minutes at 25℃. After washing with PBS, the cells were resuspended in PBS and 1% paraformaldehyde, and examined with Olympus Binocular Microscope (BX50F-3). The monoclonal antibodies used for this study were CD1a-FITC, CD83-FITC and CD86-FITC.
For the study of cocultures by scanning electron microscopy, the cells and tissues were removed and fixed with 4% paraformaldehyde/2.5% glutaraldehyde/0.02% picric acid in 0.1 M sodium cacodylate buffer (pH 7.3). After rinsing the cells with 0.06 M phosphate buffer, samples for scanning electron microscopy were prepared and investigated using a DSM 940A (Carl Zeiss; Germany).
The PBMNs were cultured in 75 cm2 flasks at a humidified atmosphere of 5% CO2 and 37℃. With these conditions, freshly isolated PBMNs showed a homogenous population of equally sized and round cells under the phase contrast micrograph (Fig. 1A). After 5 days of culture spindle-shape cells appeared in the flasks (Fig. 1B). Some adherent cell clusters with the speculated and elongated cytoplasmic projection were found on 8 days of culture (Fig. 1C). These cells were separated, and became motile, veiled and non-adherent cells at 14 days of culture (Fig. 2).
The mature DC directly contacted with thyroid cancer tissues showed profuse pseudopods and ruffled veils (Fig. 5). The lymphocytes cocultured with normal thyroid tissues were not activated (Fig. 6). The lymphocytes cocultured with the thyroid cancer tissues were activated and showed profuse pseudopods interdigitating with papillary carcinoma tissues. The CTLs attacked the cancer tissues vigorously (Fig. 7).
This paper describes the induction of DCs from PBMNs purified from the patients with thyroid cancer with an one-step process. In this study, DCs were established from the PBMNs of the thyroid cancer patients by culturing in the presence of FL, GM-CSF, IL-4 and TNF-α for 14 days. the DCs and autologous T cells were incubated with the lysates of the normal thyroid tissues or cancer tissues, respectively for 3 days. At day 18, intact cancer tissues or normal thyroid tissues were incubated with autologous T cell, respectively.
Under the scanning electron microscopy, the morphologies of mature dendritic cells, naive T cells, and CTLs were visualized. The differentiated DCs showed the typical morphology of veiled cells with profuse and ruffled pseudopods. The size of cytotoxid T lymphocytes was twice as large as that of naive T cells.
This study provides morphologic evidence that the coculture of T cells/cancer tissues activated the T cells and differentiated CTLs. The CTLs tightly adhered to cancer tissues and lysed cancer tissues vigorously. However normal tissues were not attacked by the T cells.
Therefore DCs could be used as potential vaccines in the immunotherapy, which suggest efficient strategy for minimal residual disease of cancer after chemotherapy or surgery.
References
1. Steinman RM. The dendritic cell system and its role in immunogenicity. Annu Rev Immunol. 1991; 9:271–296. PMID: 1910679.


2. Lee DH, Park JS, Eo WK, Kim WM, Kang K. Differentiation induction of dendritic cell phenotypes from human leukemic cell lines. Korean J Physiol Pharmacol. 2001; 5:79–86.
3. McKenna HJ, de Vries P, Brasel K, Lyman SD, Williams DE. Effect of flt3 ligand on the ex vivo expansion of human CD34+ hematopoietic progenitor cells. Blood. 1995; 86:3413–3420. PMID: 7579445.


4. Young JC, Varma A, Digiusto D, Backer MP. Retention of quiescent hematopoietic cells with high proliferative potential during ex vivo stem cell culture. Blood. 1996; 87:545–556. PMID: 8555476.


5. Piacibello W, Sanavio F, Garetto L, Severino A, Bergandi D, Ferrario J, Fagioli F, Berger M, Aglietta M. Extensive amplification and self-renewal of human primitive hematopoietic stem cells from cord blood. Blood. 1997; 89:2644–2653. PMID: 9108381.


6. Lyman SD, James L, Johnson L, Brasel K, de Vries P, Escobar SS, Downey H, Splett RR, Beckmann MP, McKenna HJ. Cloning of the human homologue of the murine flt3 ligand: a growth factor for early hematopoietic progenitor cells. Blood. 1994; 83:2795–2801. PMID: 8180375.


7. Monks CR, Freiberg BA, Kupfer H, Sciaky N, Kupfer A. Three-dimensional segregation of supramolecular activation clusters in T cells. Nature. 1998; 395:82–86. PMID: 9738502.


8. Grakoui A, Bromley SK, Sumen C, Davis MM, Shaw AS, Allen PM, Dustin ML. The immunological synapse: a molecular machine controlling T cell activation. Science. 1999; 285:221–227. PMID: 10398592.


9. Lanzavecchia A, Lezzi G, Viola A. From TCR engagement to T cell activation: a kinetic view of T cell behavior. Cell. 1999; 96:1–4. PMID: 9989490.
10. Lanzavecchia A, Sallusto F. Antigen decoding by T lymphocytes: from synapses to fate determination. Nat Immunol. 2001; 2:487–492. PMID: 11376334.


12. Ockert D, Schmitz M, Hampl M, Rieber EP. Advances in cancer immunotherapy. Immunol Today. 1999; 20:63–65. PMID: 10098323.


13. Nestle FO, Burg G, Dummer R. New perspectives on immunobiology and immunotherapy of melanoma. Immunol Today. 1999; 20:5–7. PMID: 10081221.


14. Steinman RM. Dendritic cells and immune-based therapies. Exp Hematol. 1996; 24:859–862. PMID: 8690042.
15. Schuler G, Steinman RM. Dendritic cells as adjuvants for immune-mediated resistance to tumors. J Exp Med. 1997; 186:1183–1187. PMID: 9379142.


16. Lotze MT. Getting to the source: dendritic cells as therapeutic reagents for the treatment of patients with cancer. Ann Surg. 1997; 226:1–5. PMID: 9242331.


17. Colaco CA. DC-based cancer immunotherapy: the sequel. Immunol Today. 1999; 20:197–198. PMID: 10203720.


18. Bottomly K. T cells and dendritic cells get intimate. Science. 1999; 283:1124–1125. PMID: 10075571.


19. Lee DH. Dendritic Cells-based vaccine and immune monitoring for hepatocellular carcinoma. Korean J Physiol Pharmacol. 2010; 14:11–14. PMID: 20221274.


Fig. 1
Phase contrast micrograph of freshly isolated PBMNs showing a homogenous population of equally sized and round cells. Original magnification ×400 (A). After 5 days of culture with medium containing GM-CSF, IL-4, TNF-α and FL, spindle-shape cells showed in the center. Original magnification ×400 (B). After 8 days of culture with medium containing GM-CSF, IL-4, TNF-α and FL, the cell with large-cell bodies and long dendritic projections was visible. Original magnification ×400 (C).
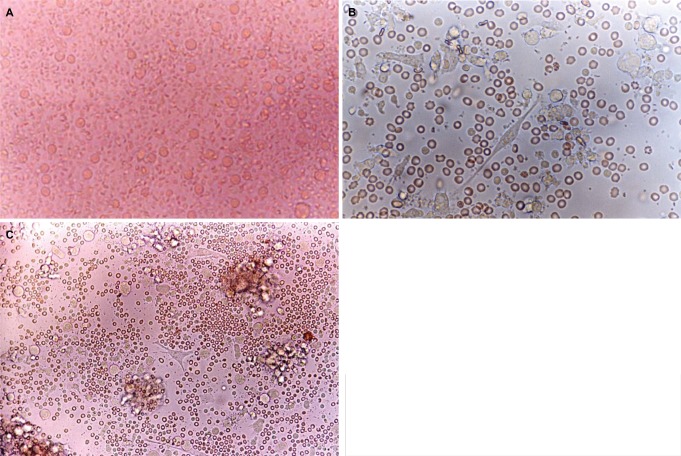
Fig. 2
The cells with long cytoplasmic projections were visible at 14 days of culture in medium containing GM-CSF, IL-4, TNF-α and FL. Original magnification ×400.
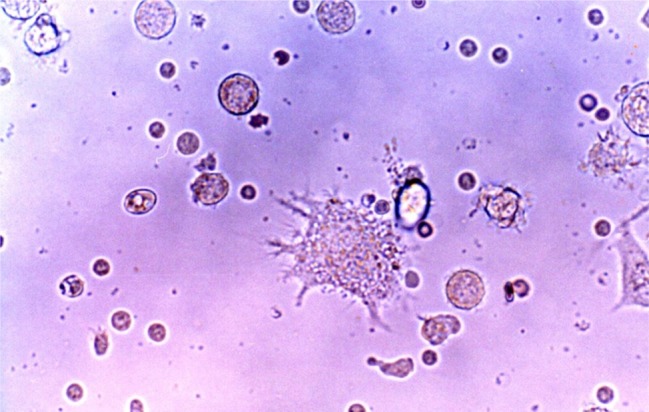
Fig. 3
The microscopic finding of non-neoplastic thyroid tissue culture after being formalin fixed paraffin embedded. This view shows dilated thyroid follicles. H-E stain. Original magnification ×100.
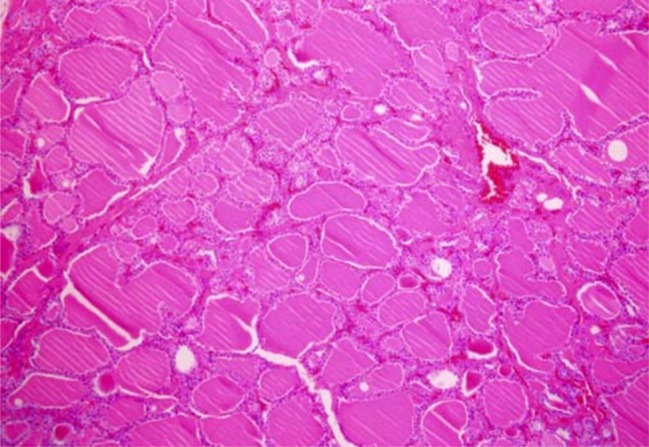
Fig. 4
Round to oval cells are arranged in clusters, and acinar pattern with intersecting scanty amyloid deposits. Tumor cells contains uniform, round to oval nuclei with finely granular chromatin, and small and visible nucleoli. Cytoplasm is abundant eosinophilic. H-E stain. Original magnification ×400.
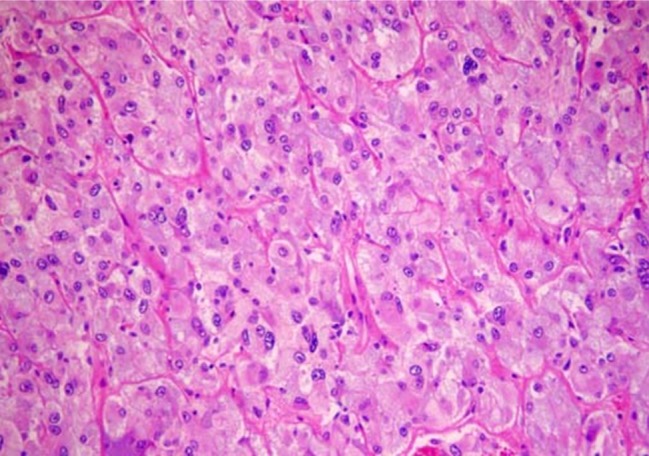
Fig. 5
Scanning electron micrograph of a veiled cell. A veiled cell is attached to the papillary thyroid cancer tissue. Original magnification ×5,000.
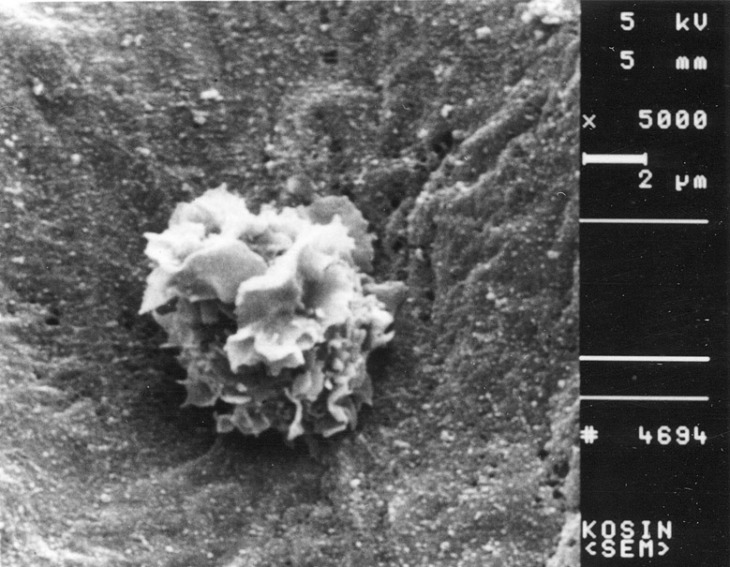
Fig. 6
Scanning electron micrograph of a non-activated T cell. This view shows a T cell cultured with normal thyroid tissue. Original magnification ×5,000.
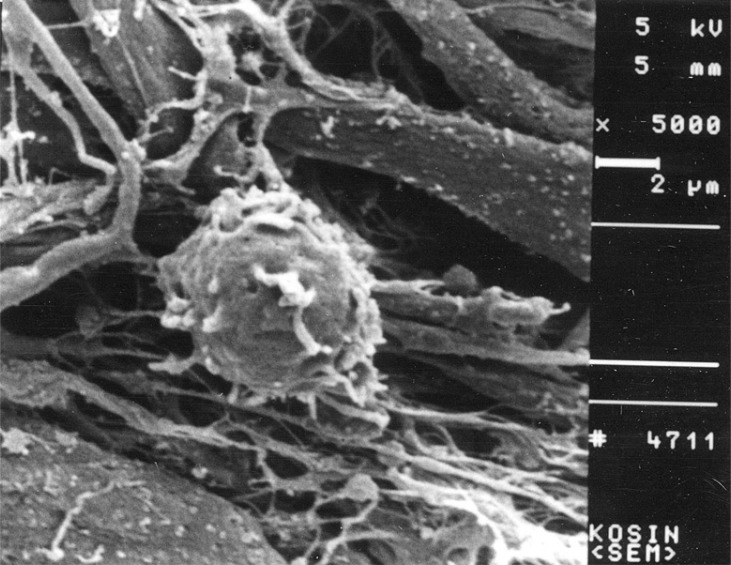