Abstract
Although various derivatives of caffeic acid have been reported to possess a wide variety of biological activities such as neuronal protection against excitotoxicity and anti-inflammatory property, the biological activity of 3,4,5-trihydroxycinnamic acid (THC), a derivative of hydroxycinnamic acids, has not been clearly examined. The objective of the present study is to evaluate the anti-inflammatory effects of THC on lipopolysaccharide (LPS)-stimulated BV2 microglial cells. THC significantly suppressed LPS-induced excessive production of nitric oxide (NO) and expression of iNOS, which is responsible for the production of iNOS. THC also suppressed LPS-induced overproduction of pro-inflammatory cytokines such as IL-1β and TNF-α in BV2 microgilal cells. Furthermore, THC significantly suppressed LPS-induced degradation of IκB, which retains NF-κB in the cytoplasm. Therefore, THC attenuated nuclear translocation of NF-κB, a major pro-inflammatory transcription factor. Taken together, the present study for the first time demonstrates that THC exhibits anti-inflammatory activity through the suppression of NF-κB transcriptional activation in LPS-stimulated BV2 microglial cells.
Microglia are resident immune cells in the central nervous system that respond to extracellular stimuli and play a crucial role in host defense and tissue repair in brain [1,2]. However, aberrant activation of microglia has also been proposed to play a pathogenic role in neuro-inflammatory conditions such as ischemia, multiple sclerosis, Parkinson's disease and Alzheimer's disease [3-6]. Under these CNS disease conditions, activated microglia have been suggested to contribute to neurodegenerative process by producing pro-inflammatory mediators such as NO and several cytokines such as tumor necrosis factor-α (TNF-α), interleukin-1β (IL-1β) [7-10]. Therefore, suppression of aberrant microglial activation may have valuable therapeutic potential for the treatment of inflammatory diseases in the CNS caused by activated microglia.
3,4,5-Trihydroxycinnamic acid (THC) is a derivative of hydroxycinnamic acids. Hydroxycinnamic acids have been reported to possess a wide variety of biological properties such as anti-inflammatory, anti-tumor, and neuroprotective activities [11-13]. Ester derivatives of hydroxycinnamic acids have been also reported to exhibit various biological activities [14]. Furthermore, it has been also reported that CAPE, caffeic acid phenylethyl ester, inhibits cytokine-mediated NF-κB activation in murine macrophage cells [15]. Although THC was previously identified in Rooibos tea (Aspalathus linearis) [16], its biological activity has not been extensively examined. Recently, it has been reported that THC inhibits human neutrophil elastase, which causes degradation of healthy tissues contributing to inflammatory diseases such as rheumatoid arthritis and psoriasis [11].
In the present study, in order to provide a novel pharmacological agent that could suppress abnormally activated microglia, the anti-inflammatory activity of THC, a derivative of hydroxycinnamic acids, and its underlying mechanism were examined in LPS-stimulated BV2 microglial cells.
Bacterial lipopolysaccharide (LPS) from Escherichia coli serotype 055:B5 was purchased from Sigma-Aldrich (St. Louis, MO, USA). 3,4,5-Trihydroxycinnamic aicd (THC) (Fig. 1) was purchased from AApin Chemicals Limited (Abingdon, UK). The compound was solubilized in dimethyl sulfoxide (DMSO) and added to the cell culture at the desired concentrations. The BV2 microglial cell line was maintained in Dulbecco's modified Eagle's medium (DMEM; Gibco BRL, Grand Island, NY, USA) containing 5% heat-inactivated fetal bovine serum (FBS; Gibco BRL) and 50 µg/ml gentamicin (Sigma, St. Louis, MO, USA) at 37℃, 5% CO2. In all experiments, cells were incubated in the presence of the indicated concentration of THC before the addition of LPS (200 ng/ml).
Cell viability was determined by 3-(4,5-dimethylthiazol-2-yl)-2,5-diphenyltetrazolium bromide (MTT) assay. In brief, cells (5×105) were seeded in 12 well plates and treated with various concentrations of THC for 24 hr. MTT (0.5 mg/ml in PBS) was added to each well, and the cells were incubated for 3 hr at 37℃ and 5% CO2. The resulting formazan crystals were dissolved in dimethyl sulfoxied (DMSO). Absorbance was determined at 540 nm. The results were expressed as a percentage of surviving cells over control cells.
The production of NO was estimated by measuring the amount of nitrite, a stable metabolite of NO, using the Griess reagent as described [14]. After THC-pretreated BV2 microglial cells were stimulated with LPS in 12-well plates for 24 hr, and then 100 µl of the cell culture media was mixed with an equal volume of Griess reagent. Light absorbance was read at 540 nm. The results were expressed as a percentage of released NO from LPS-stimulated BV2 cells. To prepare a standard curve, sodium nitrite was used to prepare a standard curve.
The BV2 microglial cells were treated with THC in the absence or presence of LPS. After 24 hr incubation, TNF-α and IL-1β levels in culture media were quantified using monoclonal anti-TNF-α or IL-1β antibodies according to the manufacturer's instruction (R&D Systems).
The BV2 microglial cells were pretreated with THC for 1 hr and stimulated with LPS. Cells were washed with PBS and lysed with lysis buffer [50 mM Tris-HCl, pH 8.0, 150 mM NaCl, 0.02% sodium azide, 0.1% sodium dodecyl sulfate (SDS), 1% NP-40, 0.5% sodium deoxycholate, 1 mM phenyl methylsulfonyl fluoride]. Equal amounts of protein were separated on 10% SDS-polyacrylamide gel. Proteins were transferred to Hypond PVDF membrane (Amersham Biosciences, Piscataway, NJ, USA) and blocked in 5% skim milk in TBST for 1 hr at room temperature. Specific antibodies against inducible NO synthase (iNOS), IκB-α (1:1,000; Santa Cruz Biotechnology Inc) and β-actin (1:2,500; Sigma) were diluted in 5% skim milk. After thoroughly washing with TBST, horseradish peroxidase-conjugated secondary antibodies were applied. The blots were developed by the enhanced chemiluminescence detection (Amersham Biosciences).
The BV2 microglial cells were treated with THC in the absence or presence of LPS (200 ng/ml) for 6 h. Total RNA was isolated using the Total RNA Extraction Kit (iNtRON Biotechnology, Inc, USA) according to the manufacturer's instruction. The total RNA (2 µg) obtained from cells was reverse-transcribed using oligo-(dT) 15 primers (Promega, Madison, WI, USA). PCR amplification conditions using primer sets specific for iNOS, TNF-α, IL-1β and β-actin were optimized for each pair of primers. PCR primers were as follows: mouse iNOS forward, 5'-CCCTTC CGAAGTTTCTGGCAGCAG C-3'; iNOS reverse, 5'-GGCTGTCAGAGCCTCGTGGCTTTG G-3'; TNF-α forward, 5'-CATCTTCTCAAAATTCGAGTGACAA-3'; TNF-α reverse, 5'-ACTTGGGC AGATTGACCTCA G-3'; IL-1β forward, 5'-GCAACTGTTCCTGAACTC-3'; IL-1β reverse, 5'-CTCGGAGCCTG TAGTGCA-3'; β-actin forward, 5'-ATCCTGA AAGACCTCTATGC-3'; β-actin reverse, 5'-AACGCAGCTCAGTAACAGTC-3'. Parallel PCR analysis was run for the house keeping gene β-actin to normalize data for differences in mRNA quantity and integrity. PCR products were separated on agarose gel.
The BV2 microglial cells were cultured in sterile coverslips and pretreated with THC for 1 hr and stimulated with LPS. After 30 min, the cells were fixed in 4% paraformaldehyde for 20 min at room temperature. The fixed cells were then permeabilized with 0.1% Triton X-100 in PBS and blocked with 3% BSA. Afterwards, the cells were sequentially incubated with rabbit anti-p65 antibody (1 : 100; Santa Cruz Biotechnology Inc) at room temperature and Alexa 546-labeled goat anti-rabbit IgG (Molecular Probes, Eugene, OR, USA) at room temperature for 1 hr. Cells were washed with PBS, and then stained with 0.5 µg/ml of Hoechst staining solution for 10 min at room temperature. After washing with PBS, the coverslips were mounted and observed by confocal microscopy.
All values shown in the figures are expressed as the mean±SD obtained from at least three independent experiments. Statistical analysis was carried out by one-way analysis of variance (ANOVA) with Tukey's post-hoc test using SPSS software 12K (SPSS, Chicago, IL, USA). A value of p<0.05 was considered as statistically significant.
Based on that NO production has been widely used as a representative measure of inflammatory activation of microglia [17], we measured suppressive effects of THC on NO production in LPS-stimulated BV2 microglial cells. Cells were incubated with indicated concentrations of THC (0, 0.1, 1, 10, 25, 50 or 100 µM) for 1 hr prior to LPS treatment and Griess reaction was used as an index for NO synthesis. As shown in Fig. 2A, LPS markedly increased NO production in BV2 microglial cells. However, THC significantly inhibited NO production in LPS-stimulated BV2 micgroglia cells in a concentration dependent manner (Fig. 2A). In addition, THC showed no significant cytotoxicity in concentration ranges, in which THC exhibits suppressive activity of NO production, irrespective of LPS presence (data not shown).
As THC was found to inhibit NO production in Fig. 2A, we examined the mRNA and protein expression levels of iNOS upon THC treatment, using RT-PCR and Western blot analyses, respectively. LPS resulted in markedly increased expression of iNOS protein, which was significantly suppressed with THC treatment in a concentration-dependent manner (Fig. 2B). Consistent with the decrease of iNOS protein, THC also significantly attenuated LPS-induced up-regulation of iNOS mRNA expression (Fig. 2C). These results demonstrate that THC possesses significant inhibitory effects on LPS-induced expression of iNOS, which is considered as a major source of cytotoxic NO.
To examine the effects of THC on TNF-α and IL-1β gene expression at the transcriptional level, RT-PCR was performed in the LPS-stimulated BV2 microglial cells. THC significantly suppressed LPS-induced expression of these genes in a concentration-dependent manner (Fig. 3A). In accordance with the change in gene expression of cytokines, THC resulted in a significant suppression of LPS-induced extracellular release of these cytokines (Fig. 3B).
Given the previous report that the transcription factor NF-κB is a predominant regulator of iNOS and numerous pro-inflammatory genes [17,18] and that IκB inhibits the nuclear translocation of p65 subunit of NF-κB, we investigated whether THC inhibits LPS-induced nuclear translocation of NF-κB and degradation of cytosolic IκB. THC blocked LPS-induced degradation of IκB in a concentration-dependent manner (Fig. 4). The cellular localization of p65 was investigated by immunofluorescence staining. Nuclear accumulation of p65 was strongly induced after stimulation of BV2 microglial with LPS (Fig. 5A). However, pretreatment of THC exhibited a significant attenuation of LPS-induced nuclear translocation of p65. Line scanning analysis of confocal images clearly demonstrated the intensity of intracellular localization of p65 subunit (Fig. 5B). These results clearly indicate that THC suppresses LPS-induced NF-κB activation in BV2 microglial cells.
The present study clearly demonstrated that THC exhibits anti-inflammatory activity in LPS-stimulated BV2 microglial cells. THC significantly suppressed LPS-induced production of NO, upregulation of iNOS expression, and release of pro-inflammatory cytokines such as TNF-α and IL-1β. Furthermore, THC significantly attenuated LPS-induced IκB degradation and consequent nuclear translocation of NF-κB, a pro-inflammatory transcription factor.
Phenylpropanoids are a group of plant-derived secondary metabolites that are biosynthesized from phenylalanine to coumaric acid and other acids including caffeic acid, ferulic acid, and trihydroxycinnamic acid. Hydroxycinnamic acids and their ester derivatives have been reported to possess a broad spectrum of pharmacological activities including antitumor, antiviral, anti-inflammatory, immunosuppressive, and neuroprotective activities [12-14]. Although it has been, recently, reported that chemically synthesized THC exerts inhibitory activity against human neutrophil elastase, which is involved in the regulation of inflammatory processes [11], the biological properties of THC have not been compared to those of other hydroxycinnamic acids. In the present study, anti-inflammatory activities of THC, originally present in Aspalathus linearis [16], was examined in comparison with caffeic acid, a prototype hydroxycinnamic acid. Both THC and caffeic acid showed significant suppression of LPS-induced NO production in BV2 microglial cells (Fig. 2A). However, THC exhibited significantly greater suppression on LPS-induced NO production compared to caffeic acid. Furthermore, THC showed suppression in a concentration-dependent manner.
Aberrant activation of microglia has been reported to contribute to neuronal damage in several pathologic conditions by releasing pro-inflammatory mediators such as TNF-α, IL-1β and NO [19,20]. Accordingly, suppression of microglial activation and subsequent release of cytokines have been demonstrated to protect neuronal damage in several inflammation-related CNS diseases [3,21,22]. The present study clearly showed that THC suppresses LPS-induced expression of TNF-α and IL-1β and their extracellular release. However, effects of THC on other pro-inflammatory cytokines have not been examined. Therefore, further studies are necessary to examine the effect of THC on the release and gene expression of other cytokines. In addition, the present study showed that THC significantly suppressed LPS-induced NO production, a well-known pro-inflammatory mediator, through the suppression of iNOS gene expression.
NF-κB is an important transcription factor for pro-inflammatory mediators such as iNOS, IL-1β and TNF-α [23,24] and inappropriate regulation of NF-κB and its downstream genes have been associated with various pathological conditions including cancer and autoimmune diseases [25,26]. It has been reported that LPS causes the nuclear translocation of p65 subunit of NF-κB through IκB degradation [27,28]. In accordance with these reports, the present study showed that THC significantly attenuated LPS-induced IκB degradation and consequent nuclear translocation of p65 in LPS-induced BV2 microglial cells (Fig. 5A).
In conclusion, the results clearly demonstrate that THC possesses anti-inflammatory activity such as suppression of NO production and cytokine release by inhibiting nuclear transclocation of NF-κB in LPS-stimulated BV2 microglial cells. The present study strongly suggests that THC might be a valuable therapeutic agent in the treatment of inflammation-related brain pathologies such as ischemia and neurodegenerative diseases. Due to the lack of reports on THC, although no noticeable cytotoxic property of THC was observed in the concentration ranges used in the present study, further studies are necessary to extensively detect any possible toxicities of THC. This endeavor could enhance the possibility of THC for therapeutic application.
Figures and Tables
Fig. 2
THC inhibited NO production (A) and expression levels of iNOS protein (B) and mRNA (C) in LPS-stimulated BV2 microglial cells. (A) BV2 cells were pretreated with various concentrations of THC for 1 hr before incubation with LPS (200 ng/ml) for 24 hr. The amounts of nitrite in the supernatants were measured using Griess reagent. THC exhibited a significant suppression of LPS- induced NO production in a concentration-dependent manner. (B) The cell lysates were subjected to SDS-PAGE, and then protein levels of iNOS were determined by Western blot analysis. (C) After LPS treatment for 6 hr, total mRNA levels of iNOS were examined by RT-PCR. The β-actin was used as an internal control. Quantification of iNOS production was performed by densitometric analysis (lower). THC significantly suppressed LPS-induced iNOS expression in a concentration-dependent manner. The data are expressed as mean±S.D. (n=3), and are representative of three or more independent experiments. *p<0.05, **p<0.01 and ***p<0.001 indicate statistically significant differences from treatment with LPS alone.
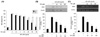
Fig. 3
THC inhibited gene expression (A) and release (B) of TNF-α and IL-1β in LPS-stimulated BV2 microglial cells. (A) BV2 microglia cells were pretreated with the indicated concentrations of THC for 1 hr before LPS treatment (200 ng/ml), and total RNA was isolated at 6 hr after LPS treatment and mRNA levels were determined by RT-PCR analysis. THC significantly suppressed expression of TNF-α and IL-1β. Data from triplicate determination are shown (mean±SD). (B) Cell culture media were collected and subjected to TNF-α and IL-1β ELISA. Data represent three independent experiments in triplicate and are expressed as mean±SD. *p<0.05 and **p<0.01 indicate statistically significant differences from treatment with LPS alone. ##p<0.01 indicates statistically significant differences between indicated groups.
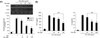
Fig. 4
Inhibitory effects of THC on LPS-induced IκB-α degration in BV2 microlial cells. Total cell lysates obtained 15 min after the LPS stimulation were subjected to Western blotting to assess the levels of IκB-α degradation (top). Quantification of IκB-α degradation was performed by densitometric analysis (lower). The β-actin was used as an internal control. Data from triplicate determination are shown (mean±S.D.). *p<0.05 and **p<0.01 indicate statistically significant differences from treatment with LPS alone.
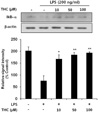
Fig. 5
THC suppressed the nuclear localization of NF-κB in LPS-stimulated BV2 microglial cells. (A) BV2 microglia cells were pretreated with THC for 1 hr prior to stimulation with 200 ng/ml LPS for 1 hr. Localization of NF-κB p65 subunit was determined using an anti-p65 antibody and an Alexa 546-labeled goat anti-rabbit IgG antibody. Nuclei were visualized by Hoechst staining. Cells were visualized using confocal scanning microscopy. Scale bar=20 µm. (B) NF-κB localization was further examined by line scannin.
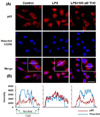
References
1. Sugama S. Stress-induced microglial activation may facilitate the progression of neurodegenerative disorders. Med Hypotheses. 2009. 73:1031–1034.
2. Perry VH, Gordon S. Macrophages and microglia in the nervous system. Trends Neurosci. 1988. 11:273–277.
3. Hailer NP. Immunosuppression after traumatic or ischemic CNS damage: it is neuroprotective and illuminates the role of microglial cells. Prog Neurobiol. 2008. 84:211–233.
4. Itagaki S, McGeer PL, Akiyama H, Zhu S, Selkoe D. Relationship of microglia and astrocytes to amyloid deposits of Alzheimer disease. J Neuroimmunol. 1989. 24:173–182.
5. Matsumoto H. Some markers reflecting the pathology and disease activity of multiple sclerosis. No To Shinkei. 1992. 44:95–102.
6. McGeer PL, McGeer EG. Glial cell reactions in neurodegenerative diseases: pathophysiology and therapeutic interventions. Alzheimer Dis Assoc Disord. 1998. 12:Suppl 2. S1–S6.
7. Tuttolomondo A, Di Raimondo D, di Sciacca R, Pinto A, Licata G. Inflammatory cytokines in acute ischemic stroke. Curr Pharm Des. 2008. 14:3574–3589.
8. Merrill JE, Benveniste EN. Cytokines in inflammatory brain lesions: helpful and harmful. Trends Neurosci. 1996. 19:331–338.
9. Chao CC, Hu S, Close K, Choi CS, Molitor TW, Novick WJ, Peterson PK. Cytokine release from microglia: differential inhibition by pentoxifylline and dexamethasone. J Infect Dis. 1992. 166:847–853.
10. Lee IS, Lim J, Gal J, Kang JC, Kim HJ, Kang BY, Choi HJ. Anti-inflammatory activity of xanthohumol involves heme oxygenase-1 induction via NRF2-ARE signaling in microglial BV2 cells. Neurochem Int. 2011. 58:153–160.
11. Steinbrecher T, Hrenn A, Dormann KL, Merfort I, Labahn A. Bornyl (3,4,5-trihydroxy)-cinnamate--an optimized human neutrophil elastase inhibitor designed by free energy calculations. Bioorg Med Chem. 2008. 16:2385–2390.
12. Nagasaka R, Chotimarkorn C, Shafiqul IM, Hori M, Ozaki H, Ushio H. Anti-inflammatory effects of hydroxycinnamic acid derivatives. Biochem Biophys Res Commun. 2007. 358:615–619.
13. Kim YC. Neuroprotective phenolics in medicinal plants. Arch Pharm Res. 2010. 33:1611–1632.
14. Lee JW, Cheong IY, Kim HS, Lee JJ, Lee YS, Kwon YS, Kim MJ, Lee HJ, Kim SS, Chun W. Anti-inflammatory activity of 1-docosanoyl cafferate isolated from rhus verniciflua in LPS-stimulated BV2 microglial cells. Korean J Physiol Pharmacol. 2011. 15:9–15.
15. Lee Y, Shin DH, Kim JH, Hong S, Choi D, Kim YJ, Kwak MK, Jung Y. Caffeic acid phenethyl ester-mediated Nrf2 activation and IkappaB kinase inhibition are involved in NFkappaB inhibitory effect: structural analysis for NFkappaB inhibition. Eur J Pharmacol. 2010. 643:21–28.
16. Rabe C, Steenkamp JA, Joubert E, Burger JF, Ferreira D. Phenolic metabolites from rooibos tea (Aspalathus linearis). Phytochem. 1994. 35:1559–1565.
17. Ock J, Kim S, Suk K. Anti-inflammatory effects of a fluorovinyloxyacetamide compound KT-15087 in microglia cells. Pharmacol Res. 2009. 59:414–422.
18. Zheng LT, Ryu GM, Kwon BM, Lee WH, Suk K. Anti-inflammatory effects of catechols in lipopolysaccharide-stimulated microglia cells: inhibition of microglial neurotoxicity. Eur J Pharmacol. 2008. 588:106–113.
19. Kreutzberg GW. Microglia: a sensor for pathological events in the CNS. Trends Neurosci. 1996. 19:312–318.
20. Graeber MB, Streit WJ. Microglia: biology and pathology. Acta Neuropathol. 2010. 119:89–105.
21. Aquilano K, Baldelli S, Rotilio G, Ciriolo MR. Role of nitric oxide synthases in Parkinson's disease: a review on the antioxidant and anti-inflammatory activity of polyphenols. Neurochem Res. 2008. 33:2416–2426.
22. Ray B, Lahiri DK. Neuroinflammation in Alzheimer's disease: different molecular targets and potential therapeutic agents including curcumin. Curr Opin Pharmacol. 2009. 9:434–444.
23. Kuprash DV, Udalova IA, Turetskaya RL, Rice NR, Nedospasov SA. Conserved kappa B element located downstream of the tumor necrosis factor alpha gene: distinct NF-kappa B binding pattern and enhancer activity in LPS activated murine macrophages. Oncogene. 1995. 11:97–106.
24. Siebenlist U, Franzoso G, Brown K. Structure, regulation and function of NF-kappa B. Annu Rev Cell Biol. 1994. 10:405–455.
25. Li Q, Verma IM. NF-kappaB regulation in the immune system. Nat Rev Immunol. 2002. 2:725–734.
26. Karin M, Takahashi T, Kapahi P, Delhase M, Chen Y, Makris C, Rothwarf D, Baud V, Natoli G, Guido F, Li N. Oxidative stress and gene expression: the AP-1 and NF-kappaB connections. Biofactors. 2001. 15:87–89.
27. Moon DO, Park SY, Lee KJ, Heo MS, Kim KC, Kim MO, Lee JD, Choi YH, Kim GY. Bee venom and melittin reduce proinflammatory mediators in lipopolysaccharide-stimulated BV2 microglia. Int Immunopharmacol. 2007. 7:1092–1101.
28. Zheng LT, Ock J, Kwon BM, Suk K. Suppressive effects of flavonoid fisetin on lipopolysaccharide-induced microglial activation and neurotoxicity. Int Immunopharmacol. 2008. 8:484–494.