Abstract
The secretion of insulin from pancreatic β-cells is triggered by the influx of Ca2+ through voltage-dependent Ca2+ channels. The resulting elevation of intracellular calcium ([Ca2+]i) triggers additional Ca2+ release from internal stores. Less well understood are the mechanisms involved in Ca2+ mobilization from internal stores after activation of Ca2+ influx. The mobilization process is known as calcium-induced calcium release (CICR). In this study, our goal was to investigate the existence of and the role of caffeine-sensitive ryanodine receptors (RyRs) in a rat pancreatic β-cell line, INS-1 cells. To measure cytosolic and stored Ca2+, respectively, cultured INS-1 cells were loaded with fura-2/AM or furaptra/AM. [Ca2+]i was repetitively increased by caffeine stimulation in normal Ca2+ buffer. However, peak [Ca2+]i was only observed after the first caffeine stimulation in Ca2+ free buffer and this increase was markedly blocked by ruthenium red, a RyR blocker. KCl-induced elevations in [Ca2+]i were reduced by pretreatment with ruthenium red, as well as by depletion of internal Ca2+ stores using cyclopiazonic acid (CPA) or caffeine. Caffeine-induced Ca2+ mobilization ceased after the internal stores were depleted by carbamylcholine (CCh) or CPA. In permeabilized INS-1 cells, Ca2+ release from internal stores was activated by caffeine, Ca2+, or ryanodine. Furthermore, ruthenium red completely blocked the CICR response in permeabilized cells. RyRs were widely distributed throughout the intracellular compartment of INS-1 cells. These results suggest that caffeine-sensitive RyRs exist and modulate the CICR response from internal stores in INS-1 pancreatic β-cells.
References
2. Hiriart M, Aguilar-Bryan L. Channel regulation of glucose sensing in the pancreatic β-cell. Am J Physiol Endocrinol Metab. 2008; 295:E1298–E1306.
3. Mears D. Regulation of insulin secretion in islets of Langerhans by Ca2+ channels. J Membr Biol. 2004; 200:57–66.
4. Varadi A, Rutter GA. Ca2+-induced Ca2+ release in pancreatic islet β-cells: critical evaluation of the use of endoplasmic reticulum-targeted “cameleons”. Endocrinology. 2004; 145:4540–4549.
5. Lemmens R, Larsson O, Berggren PO, Islam MS. Ca2+-induced Ca2+ release from the endoplasmic reticulum amplifies the Ca2+ signal mediated by activation of voltage-gated L-type Ca2+ channels in pancreatic β-cells. J Biol Chem. 2001; 276:9971–9977.
6. Graves TK, Hinkle PM. Ca2+-induced Ca2+ release in the pancreatic β-cell: direct evidence of endoplasmic reticulum Ca2+ release. Endocrinology. 2003; 144:3565–3574.
7. Hagar RE, Ehrlich BE. Regulation of the type III InsP3 receptor and its role in β cell function. Cell Mol Life Sci. 2000; 57:1938–1949.
8. Dyachok O, Tufveson G, Gylfe E. Ca2+-induced Ca2+ release by activation of inositol 1,4,5-trisphosphate receptors in primary pancreatic β-cells. Cell Calcium. 2004; 36:1–9.
9. Johnson JD, Kuang S, Misler S, Polonsky KS. Ryanodine receptors in human pancreatic β cells: localization and effects on insulin secretion. FASEB J. 2004; 18:878–880.
10. Bruton JD, Lemmens R, Shi CL, Persson-Sjögren S, Westerblad H, Ahmed M, Pyne NJ, Frame M, Furman BL, Islam MS. Ryanodine receptors of pancreatic β-cells mediate a distinct context-dependent signal for insulin secretion. FASEB J. 2003; 17:301–303.
11. Islam MS. The ryanodine receptor calcium channel of β-cells: molecular regulation and physiological significance. Diabetes. 2002; 51:1299–1309.
12. Kim BJ, Park KH, Yim CY, Takasawa S, Okamoto H, Im MJ, Kim UH. Generation of nicotinic acid adenine dinucleotide phosphate and cyclic ADP-ribose by glucagon-like peptide-1 evokes Ca2+ signal that is essential for insulin secretion in mouse pancreatic islets. Diabetes. 2008; 57:868–878.
13. Johnson JD, Misler S. Nicotinic acid-adenine dinucleotide phosphate-sensitive calcium stores initiate insulin signaling in human β cells. Proc Natl Acad Sci U S A. 2002; 99:14566–14571.
14. Islam MS, Larsson O, Nilsson T, Berggren PO. Effects of caffeine on cytoplasmic free Ca2+ concentration in pancreatic β-cells are mediated by interaction with ATP-sensitive K+ channels and L-type voltage-gated Ca2+ channels but not the ryanodine receptor. Biochem J. 1995; 306:679–686.
15. Rutter GA, Theler JM, Li G, Wollheim CB. Ca2+ stores in insulin-secreting cells: lack of effect of cADP ribose. Cell Calcium. 1994; 16:71–80.
16. Chen TH, Lee B, Yang C, Hsu WH. Effects of caffeine on intracellular calcium release and calcium influx in a clonal β-cell line RINm5F. Life Sci. 1996; 58:983–990.
17. Gamberucci A, Fulceri R, Pralong W, Bánhegyi G, Marcolongo P, Watkins SL, Benedetti A. Caffeine releases a glucose-primed endoplasmic reticulum Ca2+ pool in the insulin secreting cell line INS-1. FEBS Lett. 1999; 446:309–312.
18. Dror V, Kalynyak TB, Bychkivska Y, Frey MH, Tee M, Jeffrey KD, Nguyen V, Luciani DS, Johnson JD. Glucose and endoplasmic reticulum calcium channels regulate HIF-1β via presenilin in pancreatic β-cells. J Biol Chem. 2008; 283:9909–9916.
19. Takasawa S, Kuroki M, Nata K, Noguchi N, Ikeda T, Yamauchi A, Ota H, Itaya-Hironaka A, Sakuramoto-Tsuchida S, Takahashi I, Yoshikawa T, Shimosegawa T, Okamoto H. A novel ryanodine receptor expressed in pancreatic islets by alternative splicing from type 2 ryanodine receptor gene. Biochem Biophys Res Commun. 2010; 397:140–145.


20. Li F, Zhang ZM. Comparative identification of Ca2+ channel expression in INS-1 and rat pancreatic β cells. World J Gastroenterol. 2009; 15:3046–3050.
21. Park HS, Betzenhauser MJ, Won JH, Chen J, Yule DI. The type 2 inositol (1,4,5)-trisphosphate (InsP3) receptor determines the sensitivity of InsP3-induced Ca2+ release to ATP in pancreatic acinar cells. J Biol Chem. 2008; 283:26081–26088.
22. Choi KJ, Kim KS, Kim SH, Kim DK, Park HS. Caffeine and 2-aminoethoxydiphenyl borate (2-APB) have different ability to inhibit intracellular calcium mobilization in pancreatic acinar cell. Korean J Physiol Pharmacol. 2010; 14:105–111.


23. Rousseau E, Ladine J, Liu QY, Meissner G. Activation of the Ca2+ release channel of skeletal muscle sarcoplasmic reticulum by caffeine and related compounds. Arch Biochem Biophys. 1988; 267:75–86.
25. Ehrlich BE, Kaftan E, Bezprozvannaya S, Bezprozvanny I. The pharmacology of intracellular Ca2+-release channels. Trends Pharmacol Sci. 1994; 15:145–149.
26. Zalk R, Lehnart SE, Marks AR. Modulation of the ryanodine receptor and intracellular calcium. Annu Rev Biochem. 2007; 76:367–385.


27. Drews G, Krippeit-Drews P, Düfer M. Electrophysiology of islet cells. Adv Exp Med Biol. 2010; 654:115–163.


28. Braun M, Ramracheya R, Bengtsson M, Zhang Q, Karanauskaite J, Partridge C, Johnson PR, Rorsman P. Voltage-gated ion channels in human pancreatic β-cells: electrophysiological characterization and role in insulin secretion. Diabetes. 2008; 57:1618–1628.
29. Kang G, Holz GG. Amplification of exocytosis by Ca2+-induced Ca2+ release in INS-1 pancreatic β cells. J Physiol. 2003; 546:175–189.
30. Dyachok O, Gylfe E. Ca2+-induced Ca2+ release via inositol 1,4,5-trisphosphate receptors is amplified by protein kinase A and triggers exocytosis in pancreatic β-cells. J Biol Chem. 2004; 279:45455–45461.
31. Foskett JK, White C, Cheung KH, Mak DO. Inositol trisphosphate receptor Ca2+ release channels. Physiol Rev. 2007; 87:593–658.
32. Islam MS, Larsson O, Berggren PO. Cyclic ADP-ribose in β cells. Science. 1993; 262:584–586.
33. Islam MS, Rorsman P, Berggren PO. Ca2+-induced Ca2+ release in insulin-secreting cells. FEBS Lett. 1992; 296:287–291.
34. Takasawa S, Nata K, Yonekura H, Okamoto H. Cyclic ADP-ribose in insulin secretion from pancreatic β cells. Science. 1993; 259:370–373.
35. Mitchell KJ, Lai FA, Rutter GA. Ryanodine receptor type I and nicotinic acid adenine dinucleotide phosphate receptors mediate Ca2+ release from insulin-containing vesicles in living pancreatic β-cells (MIN6). J Biol Chem. 2003; 278:11057–11064.
Fig. 1.
Caffeine stimulated calcium mobilization from internal stores in intact INS-1 cells. The representative traces show the effects of repetitive 30 mM caffeine stimulation on [Ca2+]i increases in the presence (A) and absence (B) of extracellular Ca2+. The data were obtained from 5 and 7 separate experiments, respectively. INS-1 cells were responsive to repetitive caffeine stimulation in normal extracellular Ca2+ buffer, but only responded to the first caffeine stimulation in Ca2+ free solution. (C) A 50 μM of ruthenium red markedly reduced the [Ca2+]i peak in the absence of extracellular Ca2+. Data were normalized to control values and expressed as mean %±S.E. Asterisk indicates the value is significantly different from the corresponding value of caffeine alone (p<0.05).
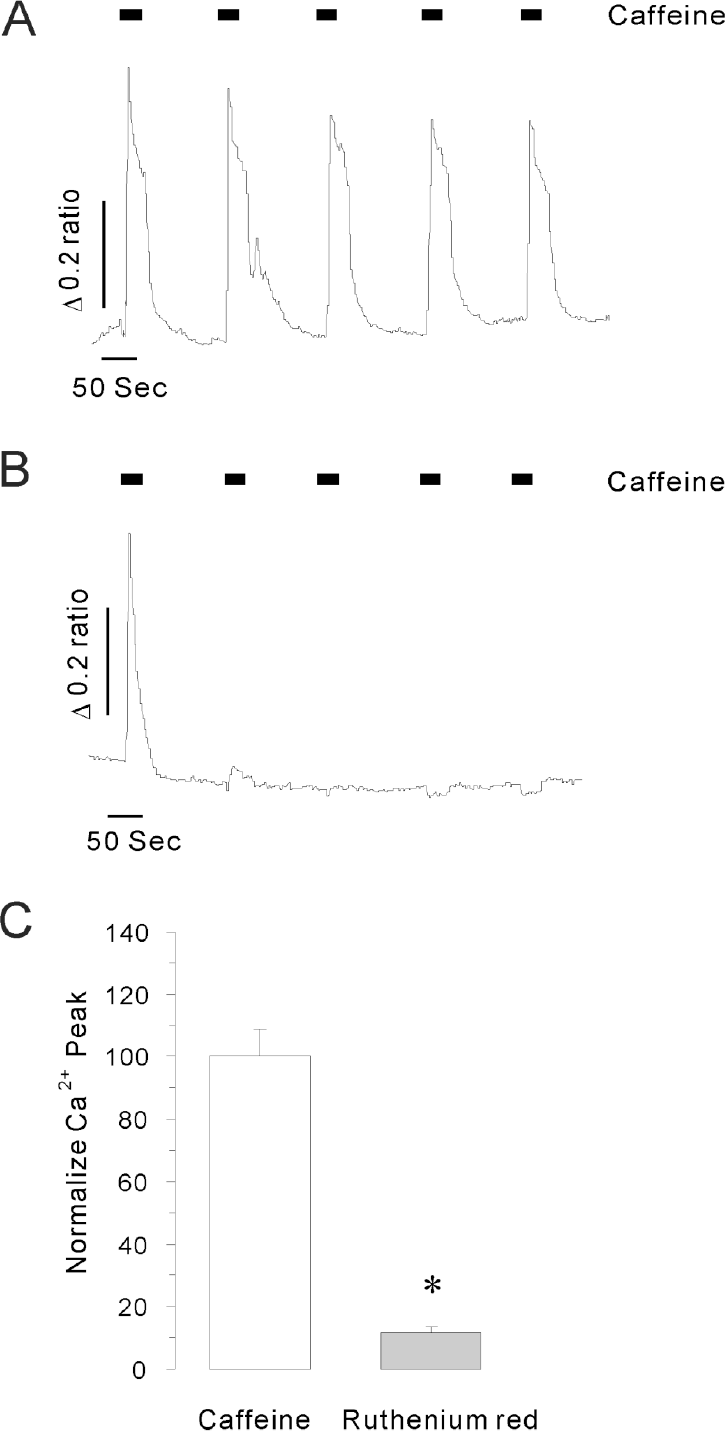
Fig. 2.
KCl triggered Ca2+ release from internal stores in intact INS-1 cells. (A) The representative trace shows the effect of 45 mM KCl on [Ca2+]i increases in the presence and absence of extracellular Ca2+. The data were obtained from 6 separate experiments. [Ca2+]i elevation was not observed in Ca2+ free medium. (B) Effects of CPA plus caffeine, CPA alone or caffeine alone on KCl-induced [Ca2+]i peaks in the presence of extracellular Ca2+. The data were obtained from at least 5 separate experiments. Data were normalized to the initial [Ca2+]i peak and expressed as mean % ± S.E. Asterisks indicate that the values are significantly different from the corresponding value for control (p<0.05). Intracellular Ca2+ store depletion reduced depolarization-induced Ca2+ mobilization. (C) Representative trace shows the effect of ruthenium red on KCl-induced [Ca2+]i elevations. The data were obtained from 6 separate experiments. A 50 μM of ruthenium red significantly reduced depolarization-induced Ca2+ mobilization; the effect was restored after washout of the ruthenium red.
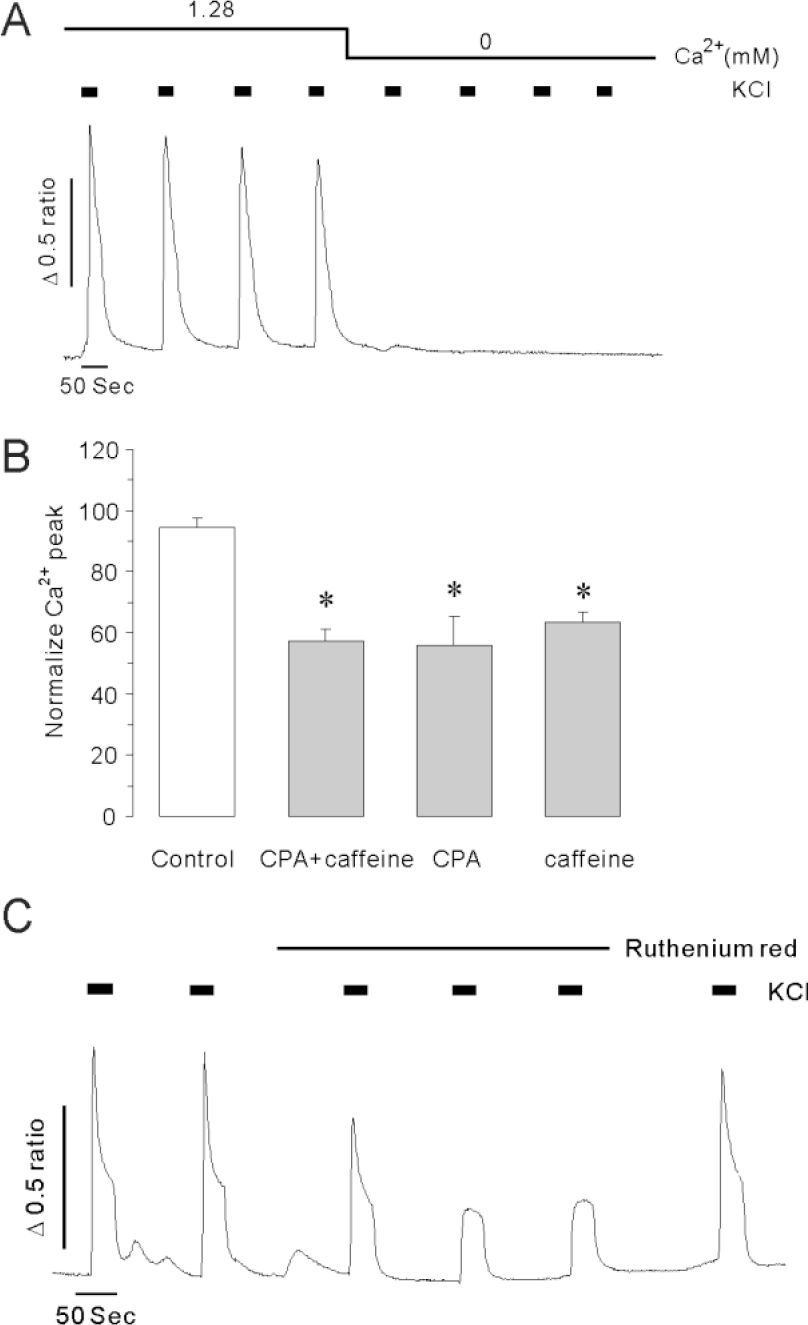
Fig. 3.
Effects of internal calcium store depletion on caffeine-induced calcium release. (A) The representative trace shows the 30 mM caffeine-induced [Ca2+]i rise after internal store depletion by 10 μM carbamylcholine (CCh) in Ca2+ free solution. (B) The representative trace shows the 10 μM CCh-induced [Ca2+]i rise under store depleted conditions induced by pretreatment with 30 mM caffeine in the absence of extracellular Ca2+. (C) A representative trace of the caffeine effect under store depleted condition induced by pretreatment of cells with 10 μM cyclopiazonic acid (CPA) in Ca2+ free solution. All data were obtained from at least 5 separate experiments. Caffeine failed to increase [Ca2+]i after internal Ca2+ store depletion induced by pretreatment with CCh or CPA.
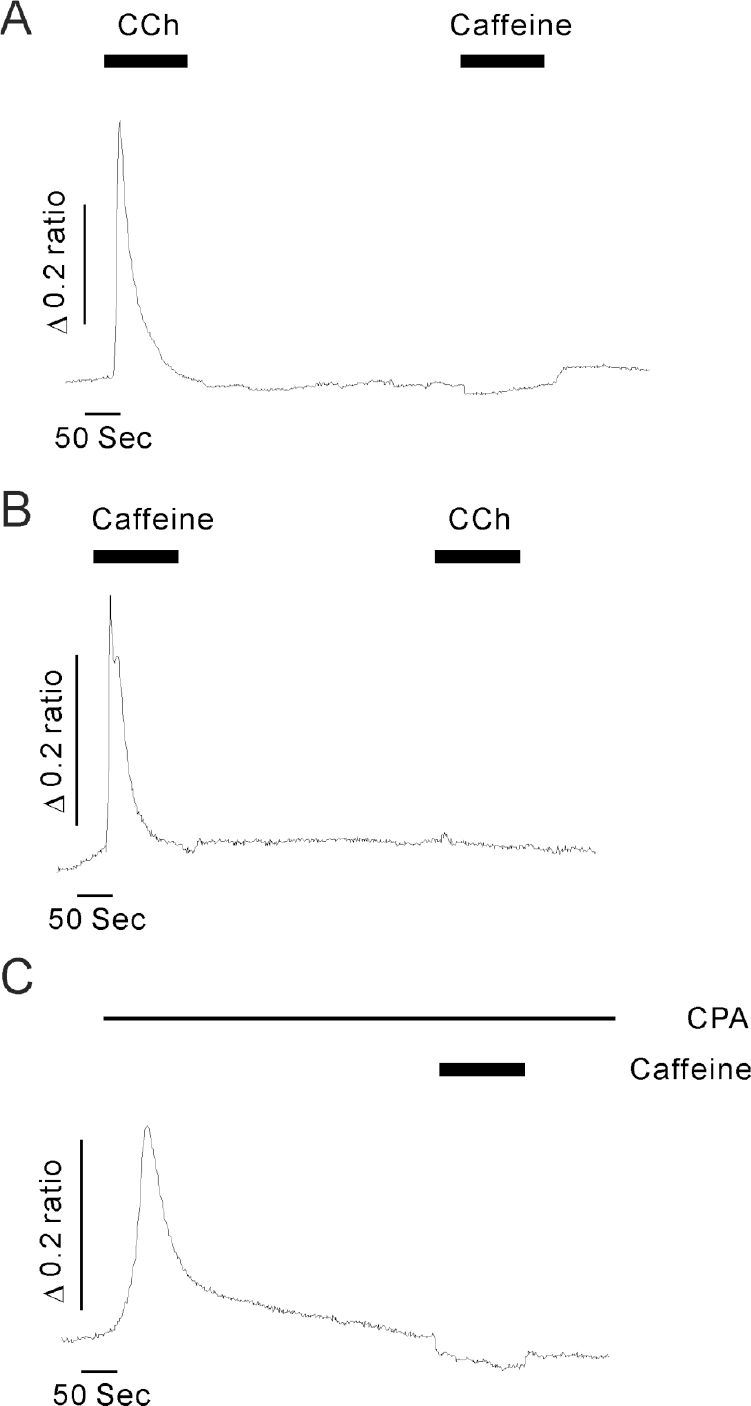
Fig. 4.
Caffeine, Ca2+ or ryanodine-induced calcium release in permeabilized INS-1 cells. (A) 10 mM caffeine (☐), 10 μM Ca2+ (⋄) or 1 μM ryanodine (Δ) significantly stimulated Ca2+ release from internal stores in permeabilized INS-1 cells. Arrows indicate the starting point of each drug perfusion. (B) Summarized Ca2+ release rates (S–1) induced by caffeine, Ca2+ or ryanodine. Data were summarized from at least 5 experiments. Asterisks indicate that the values are significantly different from the corresponding value for control (p<0.05). (C) The blocking effect of 50 μM ruthenium red on 10 μM Ca2+-induced Ca2+ release in permeabilized INS-1 cells. (D) Summarized data showing the effects of ruthenium red on Ca2+-induced Ca2+ release rates in permeabilized cells. Asterisk indicates that the value is significantly different from the corresponding value of Ca2+ (p<0.05). Ca2+ release induced by elevated Ca2+ was completely blocked by ruthenium red, a RyR blocker, in permeabilized INS-1 cells.
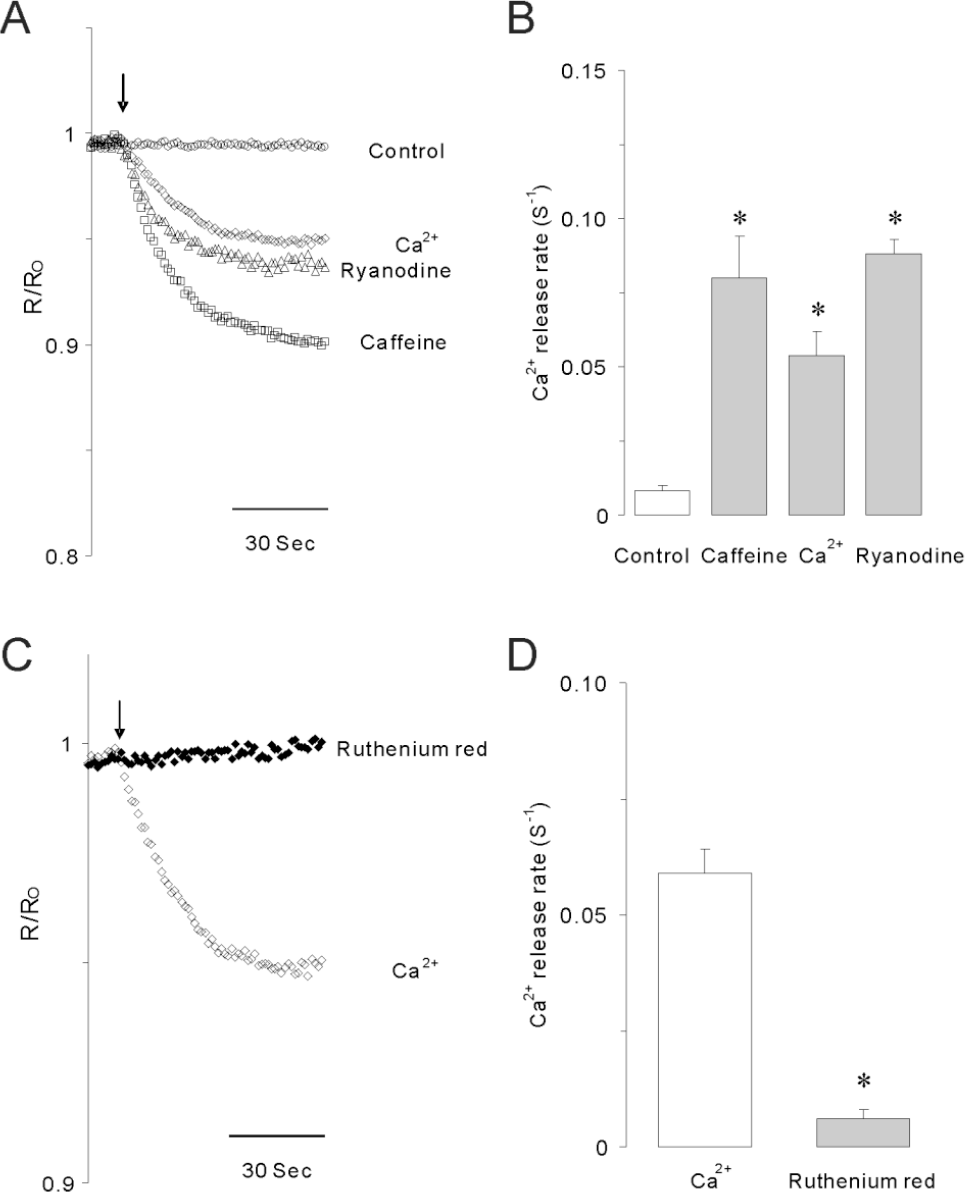
Fig. 5.
The expression of ryanodine receptors in INS-1 rat insulinoma cells. The fluorescence image (A) and the bright image (B) show the expression and distribution of RyRs in the intracellular compartments. The images were obtained from 4 separate experiments. Immunocytochemistry was done using primary RyR antibody as described under experimental procedures. The scale bar is 10 μm.
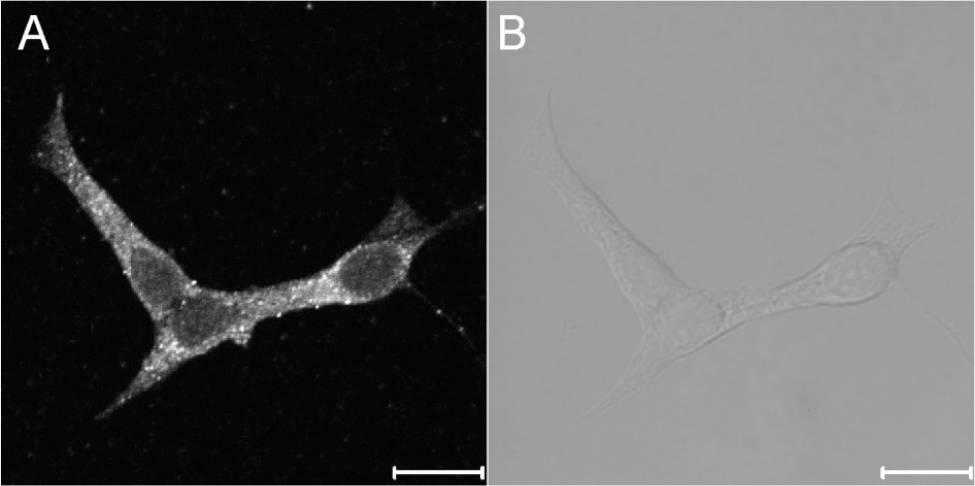