Abstract
To evaluate the effect of chlorogenic acid (CGA), a polyphenol abundant in coffee, on retinal vascular leakage in the rat model of diabetic retinopathy, Sprague-Dawley rats were divided into four groups: controls, streptozotocin-induced diabetic rats, and diabetic rats treated with 10 and 20 mg/kg chlorogenic acid intraperitoneally daily for 14 days, respectively. Blood-retinal barrier (BRB) breakdown was evaluated using FITC-dextran. Vascular endothelial growth factor (VEGF) distribution and expression level was evaluated with immunohistochemistry and Western blot analysis. Expression of tight junction proteins, occludin and claudin-5, and zonula occludens protein, ZO-1 was also evaluated with immunohistochemistry and Western blot analysis. BRB breakdown and increased vascular leakage was found in diabetic rats, with increased VEGF expression and down-regulation of occludin, claudin-5, and ZO-1. CGA treatment effectively preserved the expression of occludin, and decreased VEGF levels, leading to less BRB breakdown and less vascular leakage. CGA may have a preventive role in BRB breakdown in diabetic retinopathy by preserving tight junction protein levels and low VEGF levels.
Diabetic retinopathy causes breakdown of the blood-retinal barrier (BRB), resulting in vascular leakage and subsequent macular edema, which is a major cause of visual loss in diabetic retinopathy. Increased BRB permeability is associated with changes in expression, phosphorylation and distribution of tight junction proteins in retinal vessels (1-3), with vascular endothelial growth factor (VEGF) playing an important role (4, 5). VEGF levels are increased in diabetic rats and humans, and correlate with BRB breakdown and neovascularization, which, in turn, can be prevented by VEGF inhibition. Occludin and claudin are crucial to tight junction impermeability in endothelial cells, and are major components of the vascular barrier (6-8). Zonula occludens proteins (ZO-1, 2, and 3) also play an important role in BRB function (9).
Chlorogenic acid (CGA) is a polyphenol found in various agricultural products such as coffee, beans, potatoes, and apples, and is formed by esterification of caffeic and quinic acids. Anti-bacterial, anti-inflammatory, anti-oxidant, and anti-carcinogenic activities of CGA have been demonstrated in many studies (10-17). Also, several mechanisms have been proposed for beneficial effects of CGA on glucose metabolism. CGA may delay glucose absorption in the small intestine (18), and decrease hepatic glucose output (19, 20). Also, anti-oxidant effects, and action as a metal chelator (21) may contribute to the prevention in the development of insulin resistance and glucose intolerance. Recently, CGA was found to stimulate glucose transport in skeletal muscle (22). However, its role in diabetic retinopathy has not yet been determined.
In this study, we investigated the effects of CGA on vascular permeability in the streptozotocin (STZ)-induced diabetic retinopathy rat model.
The animals used were maintained in accordance with the ARVO Statement for the Use of Animals in Ophthalmic and Vision Research. The animal studies were performed after receiving approval of the institutional animal care and use committee (IACUC, approval number: BA1103-078/013-01) in Seoul National University Bundang Hospital. Male Sprague-Dawley rats of 6-7 weeks old were divided into four groups (8 rats per group): control, diabetics, and two treatment groups with different doses of CGA (10 and 20 mg/kg/day). Diabetes was induced with intraperitoneal injection of streptozotocin (Sigma-Aldrich, St. Louis, MO, USA, 65 mg/kg, in 10 mM citrate buffer, pH 4.5). Two days later, blood glucose levels were checked with a blood glucose meter (SD CodeFree, Standard Diagnostics, Inc., Seoul, Korea), and levels of > 250 mg/dL were considered diabetic. Blood glucose levels were checked intermittently throughout the study duration to confirm the maintenance of the diabetic condition. After maintenance of the diabetic condition was confirmed for at least one week, intraperitoneal injection of CGA (Sigma-Aldrich) was given daily in the treatment groups for 14 days. Intraperitoneal administration was selected because bioavailability after oral administration of CGA in rats has been shown to be variable in a previous study (22). The rats were sacrificed after treatment for 14 days and enucleated for evaluation.
Retinal vascular leakage was visualized using FITC-dextran (Sigma-Aldrich). Under anesthesia, intracardiac injection of FITC-dextran dissolved in phosphate buffered saline (PBS) (100 mg/mL) was performed. After 1 hr, the eyes were enucleated and fixed with 4% paraformaldehyde for 2 hr. The retina was carefully isolated, and flat-mounted, and examined under fluorescence microscopy (Carl Zeiss Meditech, Chester, VA, USA).
The eyes were enucleated and fixed in 10% PBS-buffered formaldehyde and 4 µm sections were prepared from paraffin-embedded tissues. After deparaffinization and hydration by sequential immersion in xylene and graded alcohol solutions, the tissue was treated with proteinase K for 5 min at 37℃, and then blocking solution (6% goat serum) in PBS for 30 min, and then incubated for overnight at 4℃ with anti-occludin (1:100; Zymed Laboratories, San Francisco, CA, USA), anti-claudin-5 (1:100; Abcam, Cambridge, UK), anti-ZO-1 (1:100; Zymed), or anti-VEGF (1:100, Santa Cruz, Dallas, TX, USA) antibodies. After incubation, the tissues were washed and incubated with an Alexa 488-conjugated secondary antibody (goat anti-rabbit IgG, 1:250) for 30 min. The slides were mounted in aqueous mounting medium (Vector Laboratories, Burlingame, CA, USA) and observed under light microscopy (Carl Zeiss Meditech). Immunohistochemical experiments were repeated at least three times (five slides for each eye from different animals per experiment) on different experimental days.
Retinas were carefully isolated and lysates were prepared with 0.1% Triton X-100 extraction buffer containing phenylmethylsulfonyl fluoride and dithiothreitol. Protein concentrations in tissue lysates were determined by using a protein assay (Bradford Protein Assay; Bio-Rad, Hercules, CA, USA) and the protein concentrations were adjusted to include equal total protein loading. Samples were mixed with an equal volume of sodium dodecyl sulfate (SDS) sample buffer and analyzed for occludin, claudin-5, ZO-1, and VEGF expression by SDS-polyacrylamide gel electrophoresis. Proteins were transferred electrophoretically to nitrocellulose membranes (GE Healthcare, Piscataway, NJ, USA) and rabbit polyclonal antibodies (1:1,000 in PBS with Tween 20) were used. Membranes were washed and incubated with horseradish peroxidase-tagged goat anti-rabbit antibody (1:10,000; Jackson ImmunoResearch, Suffolk, UK) and visualized. To ensure the equal loading of protein in each lane, the blots were stripped and re-probed with an antibody against β-actin (1:5,000; Santa Cruz). Quantification of the densitometry was performed by the NIH Image program (developed by Wayne Rasband, National Institutes of Health, Bethesda, MD; available at http://rsb.info.nih.gov/ij/index.html).
The body weights and blood glucose values at 14 days after chlorogenic acid administration are listed in Table 1. Body weights of diabetic rats were significantly less than those of non-diabetic rats, irrelevant to the administration of CGA (385 ± 20 g in control group versus 211 ± 30 g in STZ only group, 234 ± 42 g in STZ and CGA 10 mg/dL group, 240 ± 27 g in STZ and CGA 20 mg/dL group, P < 0.001). High non-fasting blood glucose was maintained in rats after induction of diabetes, significantly higher than in non-diabetic rats, also irrelevant to the dose of CGA (116 ± 3 mg/dL in control group versus 427 ± 64 mg/dL in STZ only group, 471 ± 65 mg/dL in STZ and CGA 10 mg/dL group, 460 ± 38 mg/dL in STZ and CGA 20 mg/dL group, P < 0.001).
To evaluate the effect of CGA on increased hyperpermeability of retina vessels in the diabetic rat model, the rats were perfused with FITC-dextran for 1 hr, and the retinas were isolated and flat-mounted. The diabetic rat showed increased leakage of FITC-dextran fluorescence compared to the control. This leakage was decreased in rats injected with 10 mg/kg CGA, and further decreased with 20 mg/kg CGA injection (Fig. 1).
On immunohistochemistry of VEGF, increased expression of VEGF was detected in the diabetic retina in the inner layer compared to the control. This increase of VEGF was attenuated with CGA administration, with further decrease with increasing dose. On Western blot analysis, VEGF level was increased nearly threefold in the diabetic retina compared to control, which was statistically significantly attenuated with 20 mg/kg CGA administration (Fig. 2).
Expression of tight junction proteins occludin and claudin-5, and zonula occludens protein ZO-1 was evaluated with immunohistochemistry. Occludin, claudin-5, and ZO-1 were found to be decreased in the diabetic retina compared to control. With CGA 20 mg/kg administration, the decrease of occludin in the diabetic retina was restored to a level similar to control. Claudin-5 level did not increase significantly with CGA administration. No change in ZO-1 expression was observed with CGA administration (Fig. 3, 4).
BRB breakdown is the hallmark of many vascular diseases in the retina. The major component of this functional barrier has long been recognized to be at the level of the tight junctions between adjacent endothelial cells. Appropriate function of these junctional proteins in endothelial cells are essential for the maintenance of the normal function of the retina, and decreased expression of these proteins are known to cause various vascular pathologies, including diabetic retinopathy. Many previous studies have demonstrated decreased occludin in retinal endothelial cells in STZ-induced diabetic rats, while the change in claudin-5 was variable (2, 24, 25). VEGF is thought to play a significant role in this pathology (1), and administration of VEGF in retinal endothelial cells and the retina caused decrease of tight junction proteins and subsequent increased leakage (26).
In this study, we demonstrated that treatment with CGA reduces VEGF expression, and restores the decrease of occludin, decreasing BRB breakdown. Partial restoration of claudin-5 level was also observed, but was not statistically significant. ZO-1 level was not affected with CGA treatment. In previous studies, many beneficial effects of CGA on the metabolism of glucose have been noted, with the possibility of improved systemic glucose control. Delayed absorption in the small intestine through the inhibition of glucose-6-phosphate translocase 1 and reduction of the sodium gradient driven apical glucose transport is thought to be one of the mechanisms (18). Also, CGA is thought to stimulate the secretion of glucagon-like peptide-1, which is known to have a beneficial effect on the response to glucose in pancreatic beta cells (27). In the liver, CGA is thought to inhibit glucose-6-phosphatase, decreasing hepatic glucose output (19, 20). However, in our study, the blood glucose levels in CGA-treated rats were not significantly less than those of diabetic rats not treated with CGA, and the beneficial effect of CGA on diabetic retinopathy can be thought to have resulted from a different mechanism of action. CGA is thought to have many other features, such as anti-oxidant, anti-microbial, anti-mutagenic, anti-obesity activities, and the exact mechanism of the reduction of retinal vascular leakage of CGA in diabetes remains to be revealed. Anti-oxidative effect of decrease of p38 MAPK and NF-κB, and anti-inflammatory effect and blockage of TNF-α are possible mechanisms. Also, occludin was the sole tight junction protein affected by CGA administration, which may represent its importance in the pathogenesis of diabetic retinopathy, however, its role also remains to be evaluated in future studies. One other notable factor is that the administration of CGA was started immediately after the induction of diabetes, possibly indicating the protective role in the prevention of the development of vascular leakage in diabetic retinopathy, rather than its role as a treatment in diabetic retinopathy.
There are many limitations in this study. The duration of chlorogenic acid administration was rather short, compared to the chronic nature of diabetes. Also, quantitative measurements and studies on the possible molecules by which chlorogenic acid might affect vascular permeability were not performed. Further studies should be conducted to elucidate the molecular and cellular mechanism underlying the protective effect of CGA against retinal vascular leakage induced by diabetes.
In summary, CGA shows a dose dependent protective effect on diabetic retinopathy in the diabetic rat model. It effectively blocks the increase of VEGF and decrease of occludin, and minimally effects claudin-5, although its mechanism remains to be revealed. Based on these findings, CGA may be suggested as a supplementary treatment for patients with diabetic retinopathy, and possibly other retinal vascular diseases caused by VEGF-dependent leakage.
Figures and Tables
Fig. 1
Leakage of retinal vessels with FITC-dextran injection. Chlorogenic acid decreased the hyperpermeability of retinal vessels in diabetic rats (scale bar: 100 µm). STZ, streptozotocin; CGA10, chlorogenic acid 10 mg/kg; CGA20, chlorogenic acid 20 mg/kg.
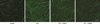
Fig. 2
VEGF expression with immunohistochemistry and Western blot analysis. VEGF expression in endothelial cells (arrows) was suppressed by chlorogenic acid administration in diabetic rats (scale bar: 50 µm). *P < 0.05 compared with control, †P < 0.05 compared with STZ. STZ, streptozotocin; CGA10, chlorogenic acid 10 mg/kg; CGA20, chlorogenic acid 20 mg/kg; GCL, ganglion cell layer; IPL, inner plexiform layer; INL, inner nuclear layer; OPL, outer plexiform layer; ONL, outer nuclear layer.
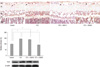
Fig. 3
Expression of occludin, claudin-5, and ZO-1 with immunohistochemistry. Occludin, claudin-5, and ZO-1 staining in the vascular endothelium of the ganglion cell layer (arrows) were found to be decreased in the diabetic retina compared to control. With CGA 20 mg/kg administration, the decrease was restored, and staining of occludin and claudin-5 in the endothelial cell junctions were increased. However, ZO-1 expression remained grossly unchanged. (scale bar: 50 µm). STZ, streptozotocin; CGA10, chlorogenic acid 10 mg/kg; CGA 20, chlorogenic acid 20 mg/kg; GCL, ganglion cell layer; IPL, inner plexiform layer; INL, inner nuclear layer; OPL, outer plexiform layer; ONL, outer nuclear layer.
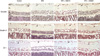
Fig. 4
Expression of occludin, claudin-5, and ZO-1 with Western blot analysis. Occludin expression was decreased in diabetic rats, and restored with chlorogenic acid administration. Claudin-5 was decreased in diabetic rats, but did not increase to significant levels with chlorogenic acid administration. ZO-1 decreased in diabetic rats, but showed no change with chlorogenic acid administration. *P < 0.05 compared with control, †P < 0.05 compared with STZ. STZ, streptozotocin; CGA10, chlorogenic acid 10 mg/kg; CGA20, chlorogenic acid 20 mg/kg.
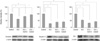
References
1. Antonetti DA, Barber AJ, Khin S, Lieth E, Tarbell JM, Gardner TW. Vascular permeability in experimental diabetes is associated with reduced endothelial occludin content: vascular endothelial growth factor decreases occludin in retinal endothelial cells: Penn State Retina Research Group. Diabetes. 1998. 47:1953–1959.
2. Barber AJ, Antonetti DA. Mapping the blood vessels with paracellular permeability in the retinas of diabetic rats. Invest Ophthalmol Vis Sci. 2003. 44:5410–5416.
3. Barber AJ, Antonetti DA, Gardner TW. Altered expression of retinal occludin and glial fibrillary acidic protein in experimental diabetes: the Penn State Retina Research Group. Invest Ophthalmol Vis Sci. 2000. 41:3561–3568.
4. Antonetti DA, Barber AJ, Hollinger LA, Wolpert EB, Gardner TW. Vascular endothelial growth factor induces rapid phosphorylation of tight junction proteins occludin and zonula occluden 1: a potential mechanism for vascular permeability in diabetic retinopathy and tumors. J Biol Chem. 1999. 274:23463–23467.
5. Harhaj NS, Felinski EA, Wolpert EB, Sundstrom JM, Gardner TW, Antonetti DA. VEGF activation of protein kinase C stimulates occludin phosphorylation and contributes to endothelial permeability. Invest Ophthalmol Vis Sci. 2006. 47:5106–5115.
6. Hirase T, Staddon JM, Saitou M, Ando-Akatsuka Y, Itoh M, Furuse M, Fujimoto K, Tsukita S, Rubin LL. Occludin as a possible determinant of tight junction permeability in endothelial cells. J Cell Sci. 1997. 110:1603–1613.
7. Turksen K, Troy TC. Barriers built on claudins. J Cell Sci. 2004. 117:2435–2447.
8. Koto T, Takubo K, Ishida S, Shinoda H, Inoue M, Tsubota K, Okada Y, Ikeda E. Hypoxia disrupts the barrier function of neural blood vessels through changes in the expression of claudin-5 in endothelial cells. Am J Pathol. 2007. 170:1389–1397.
9. Wittchen ES, Haskins J, Stevenson BR. Protein interactions at the tight junction: actin has multiple binding partners, and ZO-1 forms independent complexes with ZO-2 and ZO-3. J Biol Chem. 1999. 274:35179–35185.
10. Dos Santos MD, Almeida MC, Lopes NP, de Souza GE. Evaluation of the anti-inflammatory, analgesic and antipyretic activities of the natural polyphenol chlorogenic acid. Biol Pharm Bull. 2006. 29:2236–2240.
11. Puupponen-Pimiä R, Nohynek L, Meier C, Kähkönen M, Heinonen M, Hopia A, Oksman-Caldentey KM. Antimicrobial properties of phenolic compounds from berries. J Appl Microbiol. 2001. 90:494–507.
12. Ma CM, Kully M, Khan JK, Hattori M, Daneshtalab M. Synthesis of chlorogenic acid derivatives with promising antifungal activity. Bioorg Med Chem. 2007. 15:6830–6833.
13. Cho AS, Jeon SM, Kim MJ, Yeo J, Seo KI, Choi MS, Lee MK. Chlorogenic acid exhibits anti-obesity property and improves lipid metabolism in high-fat diet-induced-obese mice. Food Chem Toxicol. 2010. 48:937–943.
14. Lee JH, Park JH, Kim YS, Han Y. Chlorogenic acid, a polyphenolic compound, treats mice with septic arthritis caused by Candida albicans. Int Immunopharmacol. 2008. 8:1681–1685.
15. Xu Y, Chen J, Yu X, Tao W, Jiang F, Yin Z, Liu C. Protective effects of chlorogenic acid on acute hepatotoxicity induced by lipopolysaccharide in mice. Inflamm Res. 2010. 59:871–877.
16. Kim C, Yu HG, Sohn J. The anti-angiogenic effect of chlorogenic acid on choroidal neovascularization. Korean J Ophthalmol. 2010. 24:163–168.
17. Shi H, Dong L, Bai Y, Zhao J, Zhang Y, Zhang L. Chlorogenic acid against carbon tetrachloride-induced liver fibrosis in rats. Eur J Pharmacol. 2009. 623:119–124.
18. McCarty MF. A chlorogenic acid-induced increase in GLP-1 production may mediate the impact of heavy coffee consumption on diabetes risk. Med Hypotheses. 2005. 64:848–853.
19. Arion WJ, Canfield WK, Ramos FC, Schindler PW, Burger HJ, Hemmerle H, Schubert G, Below P, Herling AW. Chlorogenic acid and hydroxynitrobenzaldehyde: new inhibitors of hepatic glucose 6-phosphatase. Arch Biochem Biophys. 1997. 339:315–322.
20. Herling AW, Burger H, Schubert G, Hemmerle H, Schaefer H, Kramer W. Alterations of carbohydrate and lipid intermediary metabolism during inhibition of glucose-6-phosphatase in rats. Eur J Pharmacol. 1999. 386:75–82.
21. Rodriguez de Sotillo DV, Hadley M. Chlorogenic acid modifies plasma and liver concentrations of: cholesterol, triacylglycerol, and minerals in (fa/fa) Zucker rats. J Nutr Biochem. 2002. 13:717–726.
22. Ong KW, Hsu A, Tan BK. Chlorogenic acid stimulates glucose transport in skeletal muscle via AMPK activation: a contributor to the beneficial effects of coffee on diabetes. PLoS One. 2012. 7:e32718.
23. Gonthier MP, Verny MA, Besson C, Rémésy C, Scalbert A. Chlorogenic acid bioavailability largely depends on its metabolism by the gut microflora in rats. J Nutr. 2003. 133:1853–1859.
24. Bucolo C, Ward KW, Mazzon E, Cuzzocrea S, Drago F. Protective effects of a coumarin derivative in diabetic rats. Invest Ophthalmol Vis Sci. 2009. 50:3846–3852.
25. Leal EC, Martins J, Voabil P, Liberal J, Chiavaroli C, Bauer J, Cunha-Vaz J, Ambrósio AF. Calcium dobesilate inhibits the alterations in tight junction proteins and leukocyte adhesion to retinal endothelial cells induced by diabetes. Diabetes. 2010. 59:2637–2645.
26. Kim JH, Kim JH, Yu YS, Min BH, Kim KW. Protective effect of clusterin on blood-retinal barrier breakdown in diabetic retinopathy. Invest Ophthalmol Vis Sci. 2010. 51:1659–1665.
27. Johnston KL, Clifford MN, Morgan LM. Coffee acutely modifies gastrointestinal hormone secretion and glucose tolerance in humans: glycemic effects of chlorogenic acid and caffeine. Am J Clin Nutr. 2003. 78:728–733.