Abstract
Inflammation is closely related to the progression of cancer as well as tumorigenesis. Here, we investigated the effect of prostaglandin E2 (PGE2) and interleukin-1β (IL-1β) on E-cadherin expression in SNU719 gastric cancer cells. E-cadherin expression decreased as the dose or exposure time of PGE2 and IL-1β increased, whereas Snail expression increased with dose or time of PGE2 and IL-1β. E-cadherin expression reduced by PGE2 treatment increased after the transfection of Snail siRNA. Neutralization of IL-1β using anti-IL-1β antibody blocked the expression pattern of E-cadherin and Snail occurred by IL-1β treatment. However, there was no synergic effect of IL-1β and PGE2 on the expression pattern of E-cadherin and Snail. In conclusion, inflammatory mediators reduced E-cadherin expression by enhancing Snail expression in gastric cancer cells. Inflammation-induced transcriptional regulation of E-cadherin in gastric cancer has implications for targeted chemoprevention and therapy.
Gastric cancer is one of the world's most common cancers and represents a major clinical challenge. It is widely accepted that gastric cancer arises from Helicobacter pylori infection in a susceptible human. Inflammation may play an important role during gastric carcinogenesis induced by H. pylori. An inflammatory microenvironment promotes the changes observed in tumor cells that become metastatic and spread from the primary site (1). To stimulate this process, epithelial-to-mesenchymal transition (EMT) is required. During EMT, carcinoma cells become more motile and invasive by acquiring characteristics similar to embryonic mesenchymal cells, thereby allowing penetration of the stroma surrounding the initial neoplastic focus (2). Cell-cell adhesion mediated by E-cadherin is required for the maintenance of epithelial tissue architecture in adult organisms (3). The down-modulation of E-cadherin is an important marker for EMT (4). The loss of E-cadherin occurring during carcinoma progression can take place through gene mutations, hypermethylation of its promoter, and transcriptional repression (5). E-cadherin repressors that have been implicated in EMT are the Snail family, the basic helix-loop-helix factors E47 and Twist, and the two-handed zinc factors zinc finger E-box-binding homeobox 1 (ZEBl) and smad-interacting protein 1 (SIPl) (2).
Gastric cancer tissues release higher level of prostaglandin E2 (PGE2) when compared to non-neoplastic mucosa (6). PGE2 is related to carcinogenesis through immunosuppression, inhibiting apoptosis, increasing metastatic potential of epithelial cells and promoting angiogenesis (7, 8). Tsujii and Dubois (7) have demonstrated that the cyclooxygenase-2 (COX-2) overexpression was linked to inactivation of E-cadherin in intestinal epithelial cells. A recent study showed that down-regulation of COX-2 by celecoxib led to up-regulation of E-cadherin mRNA and protein levels in gastric cancer cell lines (9). Similar results were also observed in several human cancer cell lines (10-12). Interleukin-1β (IL-1β) is up-regulated in H. pylori-infected gastric tissue (13). IL-1β polymorphisms with T/T and T/C genotypes increase IL-1β production, and are closely related to the increased risk of gastric cancer (14). A recent study demonstrated that IL-1β treatment induced E-cadherin methylation in gastric cancer cells (15). In addition, IL-1β reduced E-cadherin expression by inducing Snail in a COX-2 dependent manner in head and neck squamous cell carcinoma (HNSCC) (16). Here, we investigated the effect of proinflammatory mediators on E-cadherin expression in gastric cancer cells.
Two gastric cancer cell lines (SNU719, SNU668) were obtained from the Korean Cell Line Bank (Seoul, Korea) and grown in RPMI 1640 (Life Technologies, Inc., Gaithersburg, MD, USA) supplemented with 10% fetal bovine serum (Life Technologies, Inc.) and gentamicin (10 µg/mL) in a 5% CO2 humidified atmosphere. PGE2 and IL-1β were purchased from Sigma (St. Louis, MO, USA) and dissolved in absolute ethanol or distilled water, respectively.
SNU719 cells (2 × 105 in 2 mL of RPMI 1640 without gentamicin) were plated in six-well plates. Twenty-four hours later the cells were transfected with vector alone or with 4 µg of pcDNA3.1-Snail (a gift of Dr. JI Yook, Department of Oral Pathology, College of Medicine Yonsei University, Seoul, Korea) using Lipofectamine 2000 (Life Technologies, Inc.) according to the manufacturer's instructions. Briefly, 4 µg of DNA was mixed with 250 µL of Opti-MEM I (Life Technologies, Inc.). The diluted DNA was combined with 10 µL of Lipofectamine 2000 diluted by 250 µL of Opti-MEM I (Life Technologies, Inc.) and incubated for 20 min at room temperature. This mixture was added to cells that had attained 90% confluence in six-well plates. After 48 hr, the cells were harvested for Western blot analysis.
SNU719 and SNU668 cells (2 × 105 in 2 mL of RPMI 1640 without gentamicin) were plated in six-well plates. Twenty-four hours later, the cells were transfected with control siRNA alone or with Snail siRNA (Santa Cruz Biotechnology, Inc., Santa Cruz, CA, USA) using siRNA transfection reagent (Santa Cruz Biotechnology, Inc.) according to the manufacturer's instructions.
Cells were washed with cold phosphate buffered saline (PBS) and suspended in lysis buffer (1 × PBS, 1% Triton X-100) supplemented with complete miniprotease inhibitor mixture tablets (Boehringer Mannheim, Mannheim, Germany) on ice for 30 min. After removing cell debris by centrifugation, cell lysate protein concentrations were determined using bicinchoninic acid protein assay reagent (Pierce, Rockford, IL, USA) with bovine serum albumin as a standard. Twenty micrograms of protein was separated by 10% sodium dodecyl sulfate (SDS)-polyacrylamide gel and transferred to a nitrocellulose membrane. The membranes were blocked with 5% skim milk in Tris-buffered saline (TBS) for 1 hr at room temperature and probed with antibodies overnight at 4℃. The antibodies used were anti-COX-2 (1:500; Cayman Chemical, Ann Arbor, MI, USA), anti-E-cadherin (1:1,000, Transduction Laboratories, Lexington, KY, USA), anti-Snail, anti-Slug (1:750, Abcam plc., Cambridge, UK), and anti-glyceraldehydes 3-phosphate dehydrogenase (anti-GAPDH; 1:200, Santa Cruz Biotechnology, Inc.). After washing with TBS-0.05% Tween 20, the blots were treated with a horseradish peroxidase-conjugated anti-rabbit IgG antibody (1:5,000; Zymed Laboratories Inc., San Francisco, CA, USA) or an anti-mouse IgG antibody (1:5,000; Zymed Laboratories Inc.) for 1 hr at room temperature. Detection was performed by enhanced chemiluminescence (Pierce) and autoradiography. We examined optical density compared with GAPDH using Scion image (Scion Corporation, Frederick, USA).
As shown in Fig. 1A, there was a reciprocal correlation between E-cadherin expression and the expression of Snail and COX-2 in SNU719 and SNU668 cells. Ectopic expression of Snail reduced E-cadherin expression in SNU719 cells (Fig. 1B). Transfection of Snail siRNA in SNU668 cells did not enhance E-cadherin expression (data not shown). Therefore, SNU719 cells were thought to be more suitable for the purpose of the present study. Fig. 2 reveals the expression of E-cadherin and Snail in SNU719 cells treated with PGE2. E-cadherin expression decreased as the dose or exposure time of PGE2 increased, whereas Snail expression increased with dose or time of PGE2. Snail siRNA blocked the expression pattern of E-cadherin occurred by PGE2 treatment in SNU719 cells as shown in Fig. 3. Fig. 4 reveals the expression of E-cadherin and Snail in SNU719 cells treated with IL-1β. E-cadherin expression decreased as the dose or exposure time of IL-1β increased, whereas Snail expression increased with dose or time of IL-1β. However, the alteration of E-cadherin and Snail after IL-1β treatment was not so marked as that of PGE2 treatment. Neutralization of IL-1β using anti-IL-1β antibody blocked the expression pattern of E-cadherin and Snail induced by IL-1β treatment in SNU719 cells as shown in Fig. 5. However, there was no synergic effect of IL-1β and PGE2 on the expression pattern of E-cadherin and Snail as shown in Fig. 6. IL-1β enhanced COX-2 expression in SNU668 cells but did not induce COX-2 expression in SNU719 cells (data not shown). In addition, ectopic expression of COX-2 in SNU719 cells did not alter the expression of Snail and E-cadherin (data not shown). Therefore, we did not evaluate relationship among COX-2, E-cadherin and Snail.
The present study showed that IL-1β and PGE2 reduced E-cadherin expression by enhancing Snail expression in gastric cancer cells. A defining characteristic of EMT is the loss of E-cadherin (4). Transcriptional repression is a predominant mechanism controlling the expression of E-cadherin in most carcinomas. Inflammation is closely associated not only with tumorigenesis, but also related to the progression of cancer. EMT takes center stage as convergence point between inflammation and the progression of cancer (17). Recent studies showed that IL-1β plays an important role in the pathogenesis of cancer and its level has been correlated with tumor invasiveness and poor prognosis (18, 19). IL-1β inhibits gastric acid secretion and its certain polymorphism leads to an increased risk of gastric cancer in H. pylori infection (20). Furthermore, in a transgenic mouse model, IL-1β induces gastric inflammation and neoplastic change (21). A recent study showed that IL-1β treatment induced E-caherin promoter hypermethytlation in several gastric cancer cell lines (15). In this study, IL-1β reduced E-cadherin expression by enhancing Snail expression in SNU719 gastric cancer cells, which was blocked by the neutralization of IL-1β using anti-IL-1β antibody. Moreover, recent studies reported that IL-1β exposure in HNSCC cells led to a significant decrease of E-cadherin expression by inducing the expression of Snail (16). This research group also documented that reduced E-cadherin expression by Snail modulated by IL-1β treatment occurred in a COX-2 dependent manner. However, in our study, IL-1β did not induce COX-2 expression in SNU719 cells. This finding was explained by the finding that SNU719 cells revealed transcriptional silencing of COX-2 by promoter hypermethylation (22). So, we could not verify whether IL-1β reduced E-cadherin expression by inducing Snail in a COX-2 dependent in gastric cancer cells. Taken together, IL-1β can reduce E-cadherin expression through transcriptional repression in gastric cancer cells.
Augmentation of PGE2 by COX-2 activation or prostaglandin dehydrogenase inactivation repressed E-cadherin expression through the induction of Snail and ZEB1 in non-small cell lung cancer and colon cancer (11, 23). E-cadherin expression was sequentially reduced via PGE2-EP2 signaling pathway during ultraviolet-induced squamous cell carcinoma progression (24). E-cadherin was upregulated and localized at intercellular junctions and the cytoplasmic membrane in etodolac-stimulated colon cancer cells (25). Down-regulation of COX-2 by celecoxib in gastric cancer cells led to up-regulation of E-cadherin (9). Short-term treatment of celecoxib in the patients of gastric cancer up-regulated E-cadherin expression (26). In the present study, we have demonstrated that PGE2 treatment for SNU719 gastric cancer cells reduced E-cadherin expression by enhancing Snail expression. This finding was confirmed as follows. First, reciprocal correlation between expression of E-cadherin and Snail was dependent on the dose or exposure time of PGE2. Second, Snail siRNA blocked the expression pattern of E-cadherin induced by PGE2 treatment.
In this study, ectopic expression of Snail reduced E-cadherin expression in SNU719 cells, however transfection of Snail siRNA in SNU668 cells did not enhance E-cadherin expression. The histopathology of SNU668 cells is signet ring cell carcinoma. Somatic alterations of the E-cadherin gene and the loss of E-cadherin expression have been reported in poorly differentiated and signet ring cell type than in well differentiated type (27, 28). In addition, SNU668 showed partial methylation in E-cadherin promoter (29). These findings suggest that the inactivation of E-cadherin in SNU668 cells take place through gene mutation or promoter hypermethylation, although other EMT regulators cannot be excluded.
In conclusion, inflammatory mediators reduced E-cadherin expression by enhancing Snail expression in gastric cancer cells. Inflammation-induced transcriptional regulation of E-cadherin in gastric cancer has implications for targeted chemoprevention and therapy.
Figures and Tables
Fig. 1
Western blot analyses of E-cadherin, COX-2 and Snail in SNU719 and SNU668 cells. (A) Endogenous expression of E-cadherin, COX-2 and Snail in SNU719 and SNU668 cells. (B) Expression of E-cadherin after ectopic expression of Snail in SNU719 cells. Twenty µg of protein was separated by 10% SDS-polyacrylamide gel electrophoresis and transferred to a nitrocellulose membrane. The bottom represents GAPDH, which was used as a loading control.
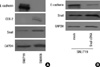
Fig. 2
Western blot analyses of E-cadherin and Snail in SNU719 cells treated with PGE2. (A, B) E-cadherin expression decreases as the dose or exposure time of PGE2 increased, whereas Snail expression increases with dose or time of PGE2. Twenty µg of protein was separated by 10% SDS-polyacrylamide gel electrophoresis and transferred to a nitrocellulose membrane. The bottom represents GAPDH, which was used as a loading control.
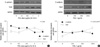
Fig. 3
Western blot analyses of E-cadherin in SNU719 cells treated with PGE2 after the transfection of Snail siRNA. Snail siRNA blocks the expression pattern of E-cadherin induced by PGE2 treatment. Twenty µg of protein was separated by 10% SDS-polyacrylamide gel electrophoresis and transferred to a nitrocellulose membrane. The bottom represents GAPDH, which was used as a loading control.
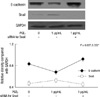
Fig. 4
Western blot analyses of E-cadherin and Snail in SNU719 cells treated with IL-1β. (A, B) E-cadherin expression decreases as the dose or exposure time of IL-1β increased, whereas Snail expression increased with dose or time of IL-1β. Twenty µg of protein was separated by 10% SDS-polyacrylamide gel electrophoresis and transferred to a nitrocellulose membrane. The bottom represents GAPDH, which was used as a loading control.
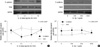
Fig. 5
Western blot analyses of E-cadherin and Snail in SNU719 cells treated with IL-1β after neutralization using anti-IL-1β antibody. Neutralization of IL-1β blocks the expression pattern of E-cadherin and Snail induced by IL-1β treatment. Twenty µg of protein was separated by 10% SDS-polyacrylamide gel electrophoresis and transferred to a nitrocellulose membrane. The bottom represents GAPDH, which was used as a loading control.
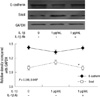
Fig. 6
Western blot analyses of E-cadherin and Snail in SNU719 cells treated with both PGE2 and IL-1β. There is no synergic effect of IL-1β and PGE2 on the expression pattern of E-cadherin and Snail. Twenty µg of protein was separated by 10% SDS-polyacrylamide gel electrophoresis and transferred to a nitrocellulose membrane. The bottom represents GAPDH, which was used as a loading control.
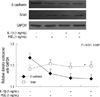
References
1. Mantovani A, Allavena P, Sica A, Balkwill F. Cancer-related inflammation. Nature. 2008. 454:436–444.
2. Guarino M, Rubino B, Ballabio G. The role of epithelial-mesenchymal transition in cancer pathology. Pathology. 2007. 39:305–318.
3. Vleminckx K, Kemler R. Cadherins and tissue formation: integrating adhesion and signaling. Bioessays. 1999. 21:211–220.
4. Thiery JP. Epithelial-mesenchymal transitions in tumour progression. Nat Rev Cancer. 2002. 2:442–454.
5. Hirohashi S. Inactivation of the E-cadherin-mediated cell adhesion system in human cancer. Am J Pathol. 1998. 153:333–339.
6. Uefuji K, Ichikura T, Mochizuki H. Cyclooxygenase-2 expression is related to prostaglandin biosynthesis and angiogenesis in human gastric cancer. Clin Cancer Res. 2000. 6:135–138.
7. Tsujii M, DuBois RN. Alterations in cellular adhesion and apoptosis in epithelial cells overexpressing prostaglandin endoperoxide synthase 2. Cell. 1995. 83:493–501.
8. Tsujii M, Kawano S, DuBois RN. Cyclooxyenase-2 expression in human colon cancer cells increases metastatic potential. Proc Natl Acad Sci U S A. 1997. 94:3336–3340.
9. Sitarz R, Leguit RJ, de Leng WW, Morsink FH, Polkowski WP, Maciejewski R, Offerhaus GJ, Milne AN. Cyclooxygenase-2 mediated regulation of E-cadherin occurs in conventional but not early-onset gastric cancer cell lines. Cell Oncol. 2009. 31:475–485.
10. Dohadwala M, Yang SC, Luo J, Sharma S, Batra RK, Huang M, Lin Y, Goodglick L, Krysan K, Fishbein MC, et al. Cyclooxygenase-2-dependent regulation of E-cadherin: prostaglandin E2 induces transcriptional repressors ZEB1 and snail in non-small cell lung cancer. Cancer Res. 2006. 66:5338–5345.
11. Jang TJ, Jeon KH, Jung KH. Cyclooxgenase-2 expression is related to the epithelial-to-mesenchymal transition in human colon cancers. Yonsei Med J. 2009. 50:818–824.
12. Jang TJ, Cha WH, Lee KS. Reciprocal correlation between the expression of cyclooxygenase-2 and E-cadherin in human transitional cell carcinomas. Virchows Arch. 2010. 457:319–328.
13. Yamaoka Y, Kita M, Kodama T, Sawai N, Kashima K, Imanishi J. Induction of various cytokines and development of severe mucosal inflammation by cagA gene positive Helicobacter pylori strains. Gut. 1997. 41:442–451.
14. El-Omar EM, Carrington M, Chow WH, McColl KE, Bream JH, Young HA, Herrera J, Lissowska J, Yuan CC, Rothman N, et al. Interleukin-1 polymorphisms associated with increased risk of gastric cancer. Nature. 2000. 404:398–402.
15. Qian X, Huang C, Cho CH, Hui WM, Rashid A, Chan AO, et al. E-cadherin promoter hypermethylation induced by interleukin-1b treatment or H. pylori infection in human gastric cancer cell lines. Cancer Lett. 2008. 263:107–113.
16. St. John MA, Dohadwala M, Luo J, Wang G, Lee G, Shih H, Heinrich E, Krysan K, Walser T, Hazra S, et al. Proinflammatory mediators upregulates Snail in head and neck squamous cell carcinoma. Clin Cancer Res. 2009. 15:6018–6027.
17. Lopez-Novoa JM, Nieto MA. Inflammation and EMT: an alliance towards organ fibrosis and cancer progression. EMBO Mol Med. 2009. 1:303–314.
18. Apte RN, Dotan S, Elkabets M, White MR, Reich E, Carmi Y, Song X, Dvozkin T, Krelin Y, Voronov E. The involvement of IL-1 in tumorigenesis, tumor invasiveness, metastasis and tumor-host interactions. Cancer Metastasis Rev. 2006. 25:387–408.
19. Krelin Y, Voronov E, Dotan S, Elkabets M, Reich E, Fogel M, Huszar M, Iwakura Y, Segal S, Dinarello CA, et al. Interleukin-1beta-driven inflammation promotes the development and invasiveness of chemical carcinogen-induced tumors. Cancer Res. 2007. 67:1062–1071.
20. McNamara D, El-Omar EM. Helicobacter pylori infection and the pathogenesis of gastric cancer: a paradigm for host-bacterial interactions. Dig Liver Dis. 2008. 40:504–509.
21. Tu S, Bhagat G, Cui G, Takaishi S, Kurt-Jones EA, Rickman B, Betz KS, Penz-Oesterreicher M, Bjorkdahl O, Fox JG, et al. Overexpression of interleukin-1beta induces gastric inflammation and cancer and mobilizes myeloid-derived suppressor cells in mice. Cancer Cell. 2008. 14:408–419.
22. Song SH, Jong HS, Choi HH, Inoue H, Tanabe K, Kim NK, Bang YJ. Transcriptional silencing of cyclooxygenase-2 by hyper-methylation of the 5' CpG island in human gastric carcinoma cells. Cancer Res. 2001. 61:4628–4635.
23. Mann JR, Backlund MG, Buchanan FG, Holla VR, Shi Q, Daikoku T, Dey SK, DuBois RN. Repression of prostaglandin dehydrogenase by epidermal growth factor and snail increases prostaglandin E2 and promotes cancer progression. Cancer Res. 2006. 66:6649–6656.
24. Brouxhon S, Kyrkanides S, O'Banion MK, Johnson R, Pearce DA, Centola GM, Miller JN, McGrath KH, Erdle B, Scott G, et al. Sequential downregulation of E-cadherin with squamous cell carcinoma progression: Loss of E-cadherin via a prostaglandin E2-EP2-dependent posttranslational mechanism. Cancer Res. 2007. 67:7654–7664.
25. Noda M, Tatsumi Y, Tomizawa M, Takama T, Mitsufuji S, Sugihara H, Kashima K, Hattori T. Effects of etodolac, a selective cyclooxygenase-2 inhibitor, on the expression of E-cadherin-catenin complexes in gastrointestinal cell lines. J Gastroenterol. 2002. 37:896–904.
26. Ran JT, Zhou YN, Tang CW, Li HH, Li XW, Lu H. Short-term preoperative treatment of celecoxib, a selective cyclooxygenase-2 inhibitor, on E-cadherin expression in gastric carcinoma tissues. Ai Zheng. 2009. 28:361–365.
27. Becker KF, Atkinson MJ, Reich U, Becker I, Nekarda H, Siewert JR, Höfler H. E-cadherin gene mutations provide clues to diffuse type gastric carcinomas. Cancer Res. 1994. 54:3845–3852.
28. Chen HC, Chu RY, Hsu PN, Hsu PI, Lu JY, Lai KH, Tseng HH, Chou NH, Huang MS, Tseng CJ, et al. Loss of E-cadherin expression correlates with poor differentiation and invasion into adjacent organs in gastric adenocarcinomas. Cancer Lett. 2003. 201:97–106.
29. Kim TY, Jong HS, Jung Y, Kim TY, Kang GH, Bang YJ. DNA hypermethylation in gastric cancer. Aliment Pharmacol Ther. 2004. 20:S131–S142.