Abstract
We investigated tissue responses to endoskeleton stent grafts for saccular abdominal aortic aneurysms (AAAs) in canines. Saccular AAAs were made with Dacron patch in 8 dogs, and were excluded by endoskeleton stent grafts composed of nitinol stent and expanded polytetrafluoroethylene graft. Animals were sacrificed at 2 months (Group 1; n = 3) or 6 months (Group 2; n = 5) after the placement, respectively. The aortas embedding stent grafts were excised en bloc for gross inspection and sliced at 5 to 8 mm intervals for histopathologic evaluation. Stent grafts were patent in all except a dog showing a thrombotic occlusion in Group 2. In the 7 dogs with patent lumen, the graft overhanging the saccular aneurysm was covered by thick or thin thrombi with no endothelial layer, and the graft over the aortic wall was completely covered by neointima with an endothelial layer. Transgraft cell migration was less active at an aneurysm than at adjacent normal aorta. In conclusion, endoskeleton stent grafts over saccular aneurysms show no endothelial coverage and poor transgraft cell migration in a canine model.
Stent grafts have been widely used for an arterial aneurysm or a pseudoaneurysm, traumatic arterial rupture, arterial occlusion and transjugular intrahepatic portosystemic shunt since Dotter introduced a stent graft (1-9). However, stent grafts are usually limited to the treatment of large arteries due to its thrombogenicity. Successful endovascular grafting requires endothelialization covering graft as soon as possible, which prevents grafts from subsequent thrombosis or excessive tissue formation. Theoretically, endothelial cells were believed to come from artery stems at anastomoses, perigraft capillaries or circulating cells (10). Migration of endothelial cells from anastomoses to graft was commonly observed in animal models, but its role has not shown a meaningful role in humans (11, 12). Some reports documented the role of perigraft capillaries or circulating cells in the endothelialization (13, 14).
The tissue reactions to stent grafts such as thrombosis or neointima were previously reported using normal vessels or fusiform aneurysm models (15-22). Though endothelial cells covered grafts in a normal artery or aorta (15-19), grafts over fusiform aneurysms did not show endothelial coverage (20-22). It is still unclear whether the endothelialization may be different between normal aorta and aneurysm in the same object. We made a saccular abdominal aortic aneurysm model which had both aneurysmal wall and normal aortic wall in the same axial plane in canine, and investigated tissue responses along the stent grafts after endovascular procedures.
Under a protocol approved by the Clinical Research Institute, 8 adult mongrel dogs of 20 to 25 kg body weight were used for this experiment. Each animal was anesthetized with an intramuscular injection of 10 mg/kg ketamine hydrochloride (Ketara; Yuhan Yanghaeng, Seoul, Korea) and xylazine hydrochloride (Rompun; Bayer Korea, Seoul, Korea). The animals were mechanically ventilated with enflurane gas (Arylane; Ilsung Pharmaceuticals Co., Seoul, Korea) after endotracheal intubation. Aneurysms were made with longitudinal incision of the abdominal aorta and attachment of Dacron patch after laparotomy. The size of Dacron patch was 2.0 × 2.0 cm to 2.6 × 2.6 cm. One week after the laparotomy, CT angiography with 3-dimensional reconstruction was performed to confirm luminal patency of the aneurysm and aorta, and to measure the aneurysm size (Fig. 1A). Mean length, depth, and width of the aneurysms were 21 ± 4 mm, 11 ± 1 mm, and 14 ± 2 mm, respectively.
A stent graft was prepared with a self-expandable stent (Niti-S M-type; Taewoong Medical, Gimpo, Korea) and expanded polytetrafluoroethylene (ePTFE) graft (ImpraR, C. R. Bard, Ariz., 10 to 40 micron internodal distance) in an endoskeleton type (Fig. 1B, C). The stent was made of 2 nitinol wires which were wound on the mandrel through a series of interlacing and interlocking patterns, and spiral mesh was created around the circumference of the mandrel. Both ends of the wire were bended at the center of the stent. Both stent tips were coiled with platinum wire for better visualization under fluoroscopy. The stent was covered with ePTFE graft with bare areas at both ends, and the stent and graft were sutured with Prolene 6-0 (EthiconR).
The size of stent grafts was determined on the basis of CT angiography performed a week after aneurysm preparation. Diameter of stent grafts was 12 mm and length was 60 mm with proximal and distal bare portions of 10 mm.
Stent grafts were inserted a month after aneurysm preparation. A 10-Fr femoral sheath was inserted through the right common carotid artery. Heparin (100 units/kg) was injected intravenously to prevent procedure-related thrombosis before endovascular procedures. Through the sheath, the pre-loaded stent graft system was introduced and the stent graft was placed to exclude the saccular aneurysm. Angiography was performed to confirm complete exclusion of the aneurysm immediately after stent graft placement.
At 2 and 6 months after stent grafting, conventional and CT angiography were performed to observe luminal patency of the stent graft. The animals were euthanized with an overdose of thiopental sodium (Pentothal; Choong Wae Pharmacy, Seoul, Korea) after follow-up angiography; 3 animals at 2 months (Group 1), and 5 animals at 6 months (Group 2) after stent grafting.
Ten thousand units of heparin were administered intravenously just before euthanizing the animals to prevent thrombosis while harvesting the aortic specimens. Abdominal aorta with a stent graft was removed en bloc, flushed with heparinized saline and fixed with 10% formalin solution. The fixed aorta was cut longitudinally at the opposite side of aneurysm for visual inspection of luminal patency and coverage. Inner surface of the aorta was pictured with a digital camera, and the surface area over the stent graft and the glossy white area without thrombi were calculated using a digital image analysis tool, Image Lab (MCM DESIGN, Hillerød, Denmark, version 2.2.4).
After gross inspection, the aorta was transected at 5 to 8 mm intervals after manual removal of stent wires, which gave 12 to 20 sections per aorta. The transected slices were processed for hematoxylin-eosin staining, Masson trichrome staining for collagen, elastic fiber staining, Factor VIII-related antigen immunohistochemical staining for endothelial cells, and smooth muscle actin immunohistochemical staining.
We divided the aorta into 6 aortic segments; proximal normal aortic segment with bare stent (PS), proximal normal aortic segment with stent graft (PG), aneurysmal side of the aortic segment of saccular aneurysm (AN) and anti-aneurysmal side of the aortic segment of saccular aneurysm (A-AN), distal normal aortic segment with stent graft (DG), and distal normal aortic segment with bare stent (DS) (Fig. 2).
We calculated the maximum distance between the center of the stent wire and the inner surface of the graft at AN and A-AN to evaluate the distance between the stent and the graft. Single-layered surface cells with brownish cytoplasm on Factor VIII-related antigen immunohistochemical staining were defined to be endothelial cells (23).
Thrombi in the excluded aneurysm were compared with luminal thrombi at AN in terms of organization degree using hematoxylin-eosin, Masson trichrome and elastic fiber staining. On 400-times magnified images, the organization of thrombi were graded as following: Grade 1, no collagen and no fibrocytes; Grade 2, collagen and less than 50 fibrocytes; Grade 3, collagen and 50 to 300 fibrocytes; and Grade 4, collagen and over 300 fibrocytes.
Transgraft cell migration was evaluated in all aortic segments covered by ePTFE graft. Spindle cells with brownish cytoplasm in the graft were considered to be cells with myoid differentiation on smooth muscle actin immunohistochemical staining. On 400-times magnified images, transgraft cell migration was graded as following: Grade 1, no collagen and no spindle cells; Grade 2, collagen and less than 10 spindle cells; Grade 3, collagen and 10 to 50 spindle cells; Grade 4, collagen and over 50 spindle cells.
Wilcoxon sign ranked test was performed for statistical analyses of the distance between stent and graft at AN vs A-AN using SPSS (version 12.0, IBM Corp., NY, USA). Mixed model was used to compare organization degrees of thrombi in an excluded aneurysm vs at AN, and transgraft cell migration at each aortic segment covered by ePTFE. P value of 0.05 or less was used to determine statistical significance.
The stent grafts were successfully deployed in all animals, and the angiography performed just after endovascular stent grafting demonstrated complete exclusion of the aneurysms from the aorta. Conventional and CT angiography at 2 months after stent grafting demonstrated persistent exclusion of the aneurysms, and luminal patency of stent grafts in all animals. There was a mural thrombus showing a crescentic low attenuation on CT (Fig. 3) along the inner surface of the stent graft overhanging saccular aneurysm neck (AN) in 5 animals. The 6-month follow-up angiography showed aortic occlusion (n = 1), more thickening (n = 1) and no significant interval change of mural thrombi (n = 1), and no mural thrombi (n = 2).
Thrombotic occlusion of the aorta was observed in an animal of Group 2. The other 7 animals showed patent aortic lumens which were covered by glossy white neointima or brownish thrombi. Glossy white neointima covered 92% ± 5% of inner surface of the stent grafts except at AN. In contrast, the inner surface of the stent grafts at AN was covered with brownish thick or thin thrombi in all 7 dogs (Fig. 4), and the stent wires were floating in the aortic lumen above the brownish thin thrombi (n = 3).
The distance between the stent and the graft was 1.15 ± 0.64 mm and 0.10 ± 0.02 mm at AN and A-AN, respectively (P < 0.001). The graft was in close contact with the stent at A-AN, whereas the graft was bulged out toward the saccular aneurysm and separated from the stent at AN.
The stent graft was covered by endothelial cells at all the aortic segments except AN (Fig. 5). The stent graft at AN was partially covered with a layer of cells which were not stained for Factor VIII-related antigen and could not be regarded as true endothelial cells.
Most thrombi were organized well in the excluded aneurysms, and 78% of the thrombi were rated as grade 4. Grade 1, 2, and 3 thrombi were 1%, 1%, and 20%, respectively. In contrast, thrombi at AN were relatively fresh and 44% of the thrombi was rated as grade 1 (Fig. 6). Grade 2, 3, and 4 thrombi were 26%, 13%, and 17%, respectively. There was a significant difference in the organization degree between thrombi in the excluded aneurysms and at AN (P = 0.0024).
Transgraft cell migration was active at the normal aortic segments (PG, A-AN, and DG), whereas it was inactive at AN (P < 0.001) (Fig. 7). Some spindle cells in the graft were positively stained for smooth muscle actin, but others were not. It suggests that myoid differentiated cells coexisted with fibroid differentiated cells in the graft.
Here, endovascular repair for a saccular aneurysm using endoskeleton stent grafts showed a regional difference in endothelial cell coverage on the graft between the normal aortic segments and aneurysm segment. At the aortic segments (PS, PG, A-AN, DG, DS) where the stent graft was in close contact with the normal aortic wall, the stent graft was completely covered by glossy white intima with a layer of endothelium even at 2-month follow-up. In contrast, the stent graft at the aneurysm segment (AN) was covered by brownish thrombi without any endothelial cells up to 6 months after stent grafting, and only a layer of cells with no Factor VIII-related antigen partially covered the thrombi. These results are well correlated with previous studies about tissue reaction to stent grafts placed in normal vessels or fusiform aneurysm models (15-22).
No endothelialization at the aneurysm segment seems to lead poor organization of thrombi, which might resulted from continuous recirculation between blood flow and thrombi. Successful endovascular grafting can be expected, when early endothelialization of graft prevents thrombosis. Zhang et al. (10) reported the possibility of permeable prosthetic graft to help organization of thrombosis. Subcellular substances such as cytokines and growth factors generated in perigraft tissues could reach thrombi in the lumen and recruit endothelial progenitor cells. Endothelial cell coverage by fallout mechanism also could be based on the transmural transfer of the substances. In this study, we did not patch any autograft over the Dacron which can enhance the subcellular substances, so the poor transmural transfer of the substances might link to no endothelialization. In addition, immature endothelial cells shown at the aneurysm segment might be affected partly by poor transmural transfer of the substances.
In a conventional surgery using interpositional PTFE graft, both graft ends of 1 to 1.5 cm anchored to the aorta were endothelialized, but the rest middle graft was not (24). Ombrellaro et al. (25) reported that endothelialization in Palmaz balloon-expandable stent graft using ePTFE was significantly better than those in interpositional PTFE graft. They hypothesized that placing a porous vascular conduit in contact with a high concentration of endothelial cells would enhance the potential for graft healing. The native artery in close contact with stent grafts can represent a suitable endothelial cell source and mediate covering the porous prosthetic grafts with endothelial cells (26). Here, we could not find any difference in endothelialization between the bare (PS, DS) and covered (PG, A-AN, DG) portions of the stent graft. Virmani et al. (18) also reported the same result in the study with a bare stent and an endoskeleton type of stent graft. At the aneurysm segment (AN), there seemed to be no endothelial cell source such as native artery to initiate endothelialization.
The wide internodal distance of ePTFE used for this study might facilitate transgraft cell migration. The stent graft completely covered by endothelial cells (aortic segments) had prominent spindle cells and collagen suggesting active transgraft cell migration. In contrast, transgraft cell migration at the aneurysm segment where there was no endothelialization was much less active. The gap between aneurysm wall and stent graft might be a barrier against transgraft cell migration, and aneurysmal wall made of Dacron could be a poor source of the cells.
The type of stent grafts could be another reason for absence of endothelialization at AN. In endoskeleton stent grafts used for this study, stent and graft were not tightly attached at the aneurysm segment, because the graft was sutured to the stent only at its both ends, and the graft was free floating over the stent. Therefore, the graft can blow out toward the aneurysm sac, and the gap between the stent and graft can be detected as a small sac collecting contrast material in conventional and CT angiography. This might cause graft to move continuously in the pulsatile aortic blood flow and make a turbulence flow between the stent and graft. It might induce thrombosis and a poor patency rate shown in endoprosthesis where the graft material is unsupported or loosely attached to the stent (27, 28).
Dacron patch can be another reason for absence of endothelialization. The patch is a good material for creating the aneurysm of intended size and shape. However, it does not allow natural expansion of an aneurysm that can be found in a true aneurysm, and it could have different hemodynamic features after endovascular grafting and prevent endothelization over the stent graft. Other aneurysm models using non-prosthetic material such as allograft or autograft might produce different results. Further investigations with different types of stent grafts, graft materials and aneurysm models are warranted to investigate factors affecting endovascular graft healing, and underlying biological mechanisms.
In conclusion, stent grafts over saccular aneurysms in a canine model show no endothelial coverage and transgraft cell migration. In the aspect of endothelialization created along the graft, our saccular aneurysm model shows same results as previous normal artery or fusiform aneurysm models. The difference in endothelialization between normal aorta and aneurysm is reproduced in the same object.
Figures and Tables
Fig. 1
An aneurysm model and an endoskeleton stent graft. (A) An abdominal aortic aneurysm is shown in CT angiography a week after laparotomy. (B, C) A self-expandable nitinol stent (B) is covered with ePTFE (C).
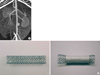
Fig. 2
Schematic division of the aorta. The aortic specimen with a stent graft was artificially divided into 6 segments for the histologic evaluation; proximal normal aortic segment with bare stent (PS), proximal normal aortic segment with stent graft (PG), aneurysmal side of the aortic segment of saccular aneurysm (AN) and anti-aneurysmal side of the aortic segment of saccular aneurysm (A-AN), distal normal aortic segment with stent graft (DG), and distal normal aortic segment with bare stent (DS). S, stent; SG, stent graft; Ao, aorta; P, proximal; D, distal.
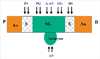
Fig. 3
Follow-up imaging after endovascular grafting. Thick tissue formation (arrow) and crescentic low attenuation at AN are demonstrated in conventional (A) and CT (B) angiography 6 months after grafting.
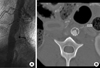
Fig. 4
Morphometric analysis of neointima. There is a lack of glossy white neointimal coverage at AN (A). Glossy white neointimal coverage is demonstrated in 91% of the inner surface of the stent graft where there is a layer of endothelium on microscopic inspection (B).
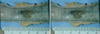
Fig. 5
Endothelialization of neointima. A layer of cells covers neointima in A-AN. The cells are strongly reactive to Factor VIII-related antigen and look brownish, which means they are endothelia (Factor VIII-related antigen immunohistochemical staining, original magnification × 400).
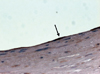
Fig. 6
Organization of thrombi. Relatively fresh thrombi (arrow) are seen along the inner surface of the stent graft in AN while organized thrombi (asterisk) are seen in the excluded saccular aneurysm (Masson trichrome stain, original magnification × 1).
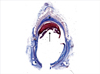
Fig. 7
Transgraft cell migration. (A) Cross section of the aorta at the level of aneurysm sac (Masson trichrome stain, original magnification × 1). (B, C) Magnified views of the red rectangular block in (A). There are lots of collagen and numerous spindle cells (arrows) in the graft. It was rated as Grade 4. (D, E) Magnified views of the black rectangular block in (A). There are little collagen and no spindle cell in the graft. It was rated as Grade 1.
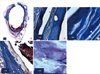
References
1. Dotter CT. Transluminally-placed coilspring endarterial tube grafts: Long-term patency in canine popliteal artery. Invest Radiol. 1969. 4:329–332.
2. May J. Abdominal aortic aneurysm: endovascular repair. Med J Aust. 2000. 173:340–341.
3. Castelli P, Caronno R, Piffaretti G, Tozzi M, Laganà D, Carrafiello G, Cuffari S, Bacuzzi A. Ruptured abdominal aortic aneurysm: endovascular treatment. Abdom Imaging. 2005. 30:263–269.
4. Hinchliffe RJ, Braithwaite BD. Ruptured abdominal aortic aneurysm: endovascular repair does not confer any long-term survival advantage over open repair. Vascular. 2007. 15:191–196.
5. Mestres G, Zarka ZA, García-Madrid C, Riambau V. Early abdominal aortic endografts: a decade follow-up results. Eur J Vasc Endovasc Surg. 2010. 40:722–728.
6. El-Sayed H, Ramlawi B. The current status of endovascular repair of thoracic aortic aneurysms (TEVAR). Methodist Debakey Cardiovasc J. 2011. 7:15–19.
7. Veraldi GF, Furlan F, Tasselli S, Tomasi I, Firpo M. Endovascular repair of intrathoracic left subclavian artery aneurysm with stent grafts: report of a case and review of the literature. Chir Ital. 2005. 57:355–359.
8. Lammer J. Femoropopliteal artery obstructions: from the balloon to the stent-graft. Cardiovasc Intervent Radiol. 2001. 24:73–83.
9. Haskal ZJ. Improved patency of transjugular intrahepatic portosystemic shunts in humans: creation and revision with PTFE stent-grafts. Radiology. 1999. 213:759–766.
10. Zhang Z, Briana S, Douville Y, Zhao H, Gilbert N. Transmural communication at a subcellular level may play a critical role in the fallout based-endothelialization of dacron vascular prostheses in canine. J Biomed Mater Res A. 2007. 81:877–887.
11. Poole-Warren LA, Schindhelm K, Graham AR, Slowiaczek PR, Noble KR. Performance of small diameter synthetic vascular prostheses with confluent autologous endothelial cell linings. J Biomed Mater Res. 1996. 30:221–229.
12. Guidoin R, Chakfé N, Maurel S, How T, Batt M, Marois M, Gosselin C. Expanded polytetrafluoroethylene arterial prostheses in humans: histopathological study of 298 surgically excised grafts. Biomaterials. 1993. 14:678–693.
13. Wu MH, Shi Q, Onuki Y, Kouchi Y, Sauvage LR. Histologic observation of continuity of transmural microvessels between the perigraft vessels and flow surface microostia in a porous vascular prosthesis. Ann Vasc Surg. 1996. 10:11–15.
14. Shi Q, Wu MH, Fujita Y, Ishida A, Wijelath ES, Hammond WP, Wechezak AR, Yu C, Storb RF, Sauvage LR. Genetic tracing of arterial graft flow surface endothelialization in allogeneic marrow transplanted dogs. Cardiovasc Surg. 1999. 7:98–105.
15. Dolmatch BL, Tio FO, Li XD, Dong YH. Patency and tissue response related to two types of polytetrafluoroethylene-covered stents in the dog. J Vasc Interv Radiol. 1996. 7:641–649.
16. Marty B, Leu AJ, Mucciolo A, von Segesser LK. Biologic fixation of polyester- versus polyurethane-covered stents in a porcine model. J Vasc Interv Radiol. 2002. 13:601–607.
17. Wilson EP, White RA, Kopchok GE, Donayre CE, de Virgilio C, Geselschap JH, Heilbron M. Deployment and healing of an ePTFE encapsulated stent endograft in the canine aorta. Ann Vasc Surg. 1997. 11:354–358.
18. Virmani R, Kolodgie FD, Dake MD, Silver JH, Jones RM, Jenkins M, Gillespie DL. Histopathologic evaluation of an expanded polytetrafluoro-ethylene-nitinol stent endoprosthesis in canine iliofemoral arteries. J Vasc Interv Radiol. 1999. 10:445–456.
19. Bashar AH, Kazui T, Terada H, Suzuki K, Washiyama N, Yamashita K, Baba S. Histological changes in canine aorta 1 year after stent-graft implantation: implications for the long-term stability of device anchoring zones. J Endovasc Ther. 2002. 9:320–332.
20. Guidoin R, Douville Y, Baslé MF, King M, Marinov GR, Traoré A, Zhang Z, Guillemot F, Dionne G, Sumanasinghe R, et al. Biocompatibility studies of the Anaconda stent-graft and observations of nitinol corrosion resistance. J Endovasc Ther. 2004. 11:385–403.
21. Palmaz JC, Tio FO, Laborde JC, Clem M, Rivera FJ, Murphy KD, Encarnacion CE. Use of stents covered with polytetrafluoroethylene in experimental abdominal aortic aneurysm. J Vasc Interv Radiol. 1995. 6:879–885.
22. Benson AE, Palmaz JC, Tio FO, Sprague EA, Encarnacion CE, Josephs SC. Polytetrafluoroethylene-encapsulated stent-grafts: use in experimental abdominal aortic aneurysm. J Vasc Interv Radiol. 1999. 10:605–612.
23. Little D, Said JW, Siegel RJ, Fealy M, Fishbein MC. Endothelial cell markers in vascular neoplasms: an immunohistochemical study comparing factor VIII-related antigen, blood group specific antigens, 6-keto-PGF1 alpha, and Ulex europaeus 1 lectin. J Pathol. 1986. 149:89–95.
24. Hanel KC, McCabe C, Abbott WM, Fallon J, Megerman J. Current PTFE grafts: a biomechanical, scanning electron, and light microscopic evaluation. Ann Surg. 1982. 195:456–463.
25. Ombrellaro MP, Stevens SL, Kerstetter K, Freeman MB, Goldman MH. Healing characteristics of intraarterial stented grafts: effect of intraluminal position on prosthetic graft healing. Surgery. 1996. 120:60–70.
26. Ombrellaro MP, Stevens SL, Sciarrotta J, Schaeffer D, Freeman MB, Goldman MH. Effect of intra-arterial environment on endothelialization and basement membrane organization in polytetrafluoroethylene grafts. Am J Surg. 1997. 174:29–32.
27. White R, Kopchok G, Zalewski M, Ayres B, Wilson E, de Virgilio C, Donayre C. Comparison of the deployment and healing of thin-walled expanded PTFE stented grafts and covered stents. Ann Vasc Surg. 1996. 10:336–346.
28. Dolmatch BL, Dong YH, Trerotola SO, Hunter DW, Brennecke LH, LaBounty R. Tissue response to covered Wallstents. J Vasc Interv Radiol. 1998. 9:471–478.