Abstract
As one of trials on neuroprotection after spinal cord injury, we used pregabalin. After spinal cord injury (SCI) in rats using contusion model, we observed the effect of pregabalin compared to that of the control and the methylprednisolone treated rats. We observed locomotor improvement of paralyzed hindlimb and body weight changes for clinical evaluation and caspase-3, bcl-2, and p38 MAPK expressions using western blotting. On histopathological analysis, we also evaluated reactive proliferation of glial cells. We were able to observe pregabalin's effectiveness as a neuroprotector after SCI in terms of the clinical indicators and the laboratory findings. The caspase-3 and phosphorylated p38 MAPK expressions of the pregabalin group were lower than those of the control group (statistically significant with caspase-3). Bcl-2 showed no significant difference between the control group and the treated groups. On the histopathological analysis, pregabalin treatment demonstrated less proliferation of the microglia and astrocytes. With this animal study, we were able to demonstrate reproducible results of pregabalin's neuroprotection effect. Diminished production of caspase-3 and phosphorylated p38 MAPK and as well as decreased proliferation of astrocytes were seen with the administration of pregabalin. This influence on spinal cord injury might be a possible approach for achieving neuroprotection following central nervous system trauma including spinal cord injury.
Numerous efforts to overcome the neurologic deficits after spinal cord injury have been attempted, and have advanced our understandings of the pathophysiologic mechanisms after spinal cord injury (SCI) (1-4). However, few clinical trials have been completed. Contemporary research on spinal cord injury has been performed in two ways. One is neuro-protection that is focused on limiting or ameliorating the secondary pathophysiologic mechanisms. The other is neuro-regeneration that is focused on overcoming an established SCI such as gliosis. We have focused on excitotoxicity as one of these efforts to reduce the secondary mechanism of SCI. Excitatory neurotransmitters such as glutamate and asparate rapidly increase and then fall following SCI. Presynaptic release, disrupted neurons, axons and/or glial cells, and especially astrocytes and oligodendrocytes, have been suggested as sources of glutamate in the gray matter and white matter. These over-expressed excitatory amino acids can subsequently cause apoptosis of neurons and glia cells, and especially oligodendrocytes (2, 5-8). Alteration of the intracellular sodium and calcium, which is mediated by the N-methyl-D-asparate (NMDA) and α-amino-3-hydroxy-5-methylisoxazole-4-propionate-kainate (AMPA-kainate) receptors subsequently produces cytotoxic edema, acidosis and activation of a number of enzymes, including phospholipase, protease and nuclease. These enzymes subsequently elicit the destruction of cellular structures and they initiate apoptosis (9).
Pregabalin is a drug that is used for controlling neurogenic pain in various clinical conditions, including diabetic neuropathy, neuralgia and complex regional pain syndrome. Its chemical structure is similar to that of gamma-aminobutyric acid (GABA). However, it does not act like GABA and it does not bind to the GABA receptors. It is known that it binds at the α2δ subunit of the voltage-controlled calcium channels. Its potent binding at this site reduces Ca2+ influx at the presynaptic nerve endings and therefore, it reduces the release of several neurotransmitters, including glutamate and noradrenalin (10-12). In vivo and in vitro studies have demonstrated the effect of pregabalin on the release of glutamate (13). However, most of these studies were designed for assessing the effect on neuropathic pain and no experimental trials have been completed in traumatic SCI. Thus, we designed an experiment to study the effect of pregabalin on traumatic SCI and hypothesized that pregabalin would act as a neuroprotector after spinal cord injury. In the previous study, we demonstrated that pregabalin could improve neurologic outcomes, reduce the apoptosis of neurons and oligodendrocytes and reduce the expression of activated microglia histologically (14). In the present study, we tried to elucidate the reproducibility of the previous results with respect to SCI and examined the anti-apoptotic and anti-inflammatory effects of pregabalin.
Caspase-3 is activated during apoptosis of many types of CNS cells, and its activation appears to be an important event in apoptosis in the central nervous system (15). In contrast, bcl-2 proteins regulate the release of cytochrome C from mitochondria, the subsequent activation of caspase and ensuing apoptotic cell death. Bcl-2 and bcl-XL are representative anti-apoptotic proteins in the family of bcl proteins (6, 15, 16). We examined the expressions of caspase-3 and bcl-2 to assess the anti-apoptotic effect of pregabalin. Secondarily, in order to investigate the anti-inflammatory effects of pregabalin, we examined p38 mitogen-activated protein kinase (p38 MAPK) expression. p38 MAPK is a member of the MAPK family, and is known to be a potent regulator of the expression of inflammatory factors such as TNF-alpha, interleukin (IL)-1, IL-6 etc. (17-19). Finally, we histopathologically investigated the effect of pregabalin on the activation of glial cells, especially astrocytes and microglia. As the previous studies have reported, the proliferation of astrocytes and microglia is one of the key features in the secondary injury mechanism, and is related to the gliosis that is known to be a major impediment of neuro-regeneration.
A total of 50 adult male Sprague-Dawley rats (body weights; 250-300 g each) were used in this study. They were kept under standardized conditions (4 rat/cage, 20℃-24℃, 45%-65% humidity, a 12 hr of daily light) and given free access to food and water throughout the study. Rats were randomly assigned to one of the following four groups before operation; the control group (Group I, n = 15, SCI alone), the sham operated group (Group II, n = 5, laminectomy only without SCI), the methylprednisolone (MP) treated group (Group III, n = 15, SCI followed by intraperitoneal injection of methylprednisolone), and the pregabalin (GP) treated group (Group IV, n = 15, SCI followed by intraperitoneal injection of pregabalin).
The rats were anesthetized with ketamine (50 mg/kg) and rompun (2 mg/kg, intraperitoneal). Their backs were shaved and then sterilized with antiseptic betadine. Lamincetomies were performed T9 after exposure of the paravertebral muscles from T8-10. All the spinal contusions were induced by 25 g-cm contusion using the Multicenter Animal Spinal Cord Injury Study (MASCIS) impactor (drop from 2.5 cm height with a rod weighing 10 g). The 25 g-cm lesion was chosen to evaluate the neuroprotection effect of the experimental trials in severe SCI and the rats would have insufficient motor recovery to ambulate over several weeks. Postoperatively, 5 mg gentamycin was administrated intramuscularly. The postoperative care procedures involved providing the rat with drinking water in the cage as well as both softened rat chow and regular pellets placed directly in the cage. The rat's bladders were emptied manually twice a day during this experiment. All of surgical interventions and the presurgical and postsurgical animal care were provided in accordance with the Laboratory Animal Welfare Act and the Guidelines and Policies for Rodent Survival Surgery, as provided by the Animal Studies Committee of the Catholic University of Korea (IACUC approval No. 2009-0086-01).
In group III, 30 mg/kg MP was administered intraperitoneally at 30 min, 12, 24, and 48 hr after the contusion. In group IV, 30 mg/kg pregabalin (Pfizer Pharmaceuticals, New York, NY, USA) was administered intraperitoneally at 30 min, 12, 24, and 48 hr after contusion. We followed the previous experiment dosage of pregabalin. It is similar to the clinical dosage that is commonly used for neuropathic pain (40-80 mg/kg/d).
A motor function scale described by Gale et al. (20) was used to evaluate the rats' motor function. The animals were allowed to move freely in an open field. An observer, who was blinded to the treatments the rats received, observed the rats for at least 1 min each and recorded movements in the hip, knee and ankle joints daily. All rats in the experimental groups were observed until sacrifice (postoperative 7 days).
All the rats were assessed using a second behavioral task, the inclined plane, which tests an animal's ability to maintain its position on a board raised in 5° increments and so it can be used as an index of hind limb strength. The maximum angle at which a rat is able to maintain its position for at least 5 sec constitutes the inclined plane score. The rats were tested on the postoperative 7th day before sacrifice.
On postoperative day 7, all the rats were killed via deep anesthesia using intraperitoneal injections of ketamine and rompun. For immunoflurorescence staining, 2 rats in the sham group and 5 rats in the other groups were randomly selected and then transcardiac perfusion was done for these rats with 100 mL of buffered saline followed by 500 mL of 4% paraformaldehyde (pH 7.4). We obtained a 1.5 cm segment of perfused spinal cord that encompassed the contusion site. The specimens were fixed in cold 4% paraformaldehyde overnight, incubated in 30% sucrose solution at room temperature for 4 hr, and then stored at -70℃. All remaining rats (3 rats in the sham and 10 rats in the other groups) were used for protein analysis. As soon as the rats were sacrificed, 3 cm spinal cord segments centered on the epicenter of the injury were dissected, removed quickly and stored at -80℃.
The tissue blocks were cut into 10 µm sections transversely to the long axis of the spinal cord. The sections were mounted on gelatin-coated slides. The mounted sections were then rinsed three times 10 min at a time with phosphate buffered saline (PBS), followed by blocking with 0.15% Triton X-100 and 5% normal horse serum (NHS) in 0.1 mM PBS for 30 min. The sections were next exposed to mouse monoclonal antibody GFAP (Calbiochem, Darmstadt, Germany), which is a specific astrocyte marker, at a dilution 1:100 in 0.1M PBS +0.15% Triton X-100 +1% NHS. The next day, the sections were incubated with a dilution of the secondary antibody, FITC conjugated anti-mouse IgG (Jackson ImmunoResearch, West Grove, PA, USA) for fluorescence. After 4 hr exposure, the slides were rinsed with 0.1 mM PBS five times, covered and then stored in a freezer. The slides were then thawed and photographed with a confocal analysis system (Olympus Microscope Confocal/Image Analysis system, Tokyo, Japan). The fluorescent light green cells were counted in a 75 × 250 µm area centered at the epicenter of the injured spinal cord. The other immunofluorescence staining was performed for identifying the microglia by using the monoclonal antibody OX-42 (1:500, Calbiochem), which labels the complement type 3 receptor on the microglia and macrophages. FITC was also used for fluorescence.
Morphometric analysis of the positively fluorescent cells was performed under high-power magnification (× 200) in a blinded fashion. On each slide, six fields were randomly selected and in order to eliminate bias from hemorrhage and necrosis, cells were counted at the peripheral margin of the cystic lesions. The mean numbers of each specimen were recorded and the mean numbers of each group were compared. To exclude the false positive counting of immunofluorescent cells, the cells which were coincident with DAPI were considered as positive cells.
The spinal cord samples were homogenized on ice in RIPA buffer (150 mM NaCl, 50 mM Tris-HCl [pH 7.4], 2 mM EDTA, 1% NP-40, 10 mM NaF, 1 mM Na3VO4, 10 mM sodium pyrophosphate, 1 mM PMSF, 10 mg/mL aprotinin, 10 mg/mL leupeptin, and 0.1 mg/mL soybean inhibitor). The lysate was centrifuged at 15,000 rpm for 20 min at 4℃. Proteins were separated by SDS-polyacrylamide gel electrophoresis and transferred to polyvinylidene difluoride membrane (Hybond-P, Amersham Pharmacia Biotech, Buckinghamshire, UK). The membrane was blocked with 5% fat-free dry milk for 1 hr in Tris-buffered saline (0.1% Tween-20, 20 mM Tris-HCl, 137 mM NaCl, pH 7.4) and then it was incubated overnight at 4℃ with the primary antibodies. The antibodies used for immunoblotting were as follows: anti-rabbit activated caspase-3 antibody (1:1,000; Cell Signaling Technology, Danvers, MA, USA), anti-rabbit phosphorylated p-38 MAPK (1:1,000; Cell Signaling Technology), anti-rabbit p-38 MAPK (1: 1,000; Cell Signaling Technology) and anti-mouse Bcl-2 (1:1,000; Santa Cruz Biotechnology, Santa Cruz, CA, USA). After the membranes were washed, they were incubated with secondary peroxidase-conjugated anti-rabbit or anti-mouse antibodies (Amersham Pharmacia Biotech) that were diluted 1:2,000 in Tris-buffered saline with 0.01% Tween 20. An antibody detection system (ECL, Amersham Pharmacia Biotech) was used and the membranes were exposed to radiography film. The protein band intensities were quantified with a VDS densitometer (Amersham Pharmacia Biotech).
The time course of functional recovery, as measured by the Gale rating score, was recorded (Fig. 1). The results of the "motor function score" evaluation are presented as a mean value with SEM (Table 1). The mean motor function scores at post-injury day 7 were 1.92 ± 0.2, 6 ± 0, 2.0 ± 0.3, and 3.17 ± 0.2 in group I to IV respectively. On the statistical analysis, the mean motor function scores of each group had a statistically significant difference (P = 0.01), which was tested for with a one-way ANOVA. The recovery of hindlimb function in the GP group was superior to that of the control and the MP groups (P = 0.01).
The results of the "inclined plane score" evaluation are presented as a mean value with SEM. The mean inclined plane angles were 28.7 ± 1.5°, 46 ± 1.0°, 27.7 ± 1.5°, and 31.3 ± 0.8° in group I to IV respectively. On the statistical analysis, the mean inclined plane scores of each group had a statistical significance (P = 0.01). Comparing the GP group with the MP group, there was also statistical significance (P = 0.01).
Most of the injured rats, except the sham operated rats, suffered from loss of body weight during the observation period. The mean changes for the control group and the MP group are larger than that for the GP group (P < 0.05, Table 1).
The relative optical densities for caspase-3 were 1.6 ± 0.16, 1.33 ± 0.02, 1.63 ± 0.2, and 0.97 ± 0.05 in group I-IV, respectively (Fig. 2A). The caspase-3 expression was significantly decreased in GP group compared to the control and MP groups (f = 3.97, P < 0.05, Bonferroni post hoc analysis, P < 0.05, n = 10). The relative optical densities for bcl-2 are 0.97 ± 0.27, 0.27 ± 0.04, 0.74 ± 0.17, and 0.8 ± 0.26 in group I-IV, respectively (Fig. 2B). However, it revealed no statistical difference between groups (f = 0.52, P = 0.67). The relative optical densities for phosphorylated p38 MAPK are 0.52 ± 0.12, 0.25 ± 0.01, 0.39 ± 0.08, and 0.4 ± 0.08 in group I-IV, respectively (Fig. 3). The control group tends to express higher levels phosphorylated p38 MAPK than the other groups. However, there was no statistically significant difference (f = 0.65, P = 0.58).
Except for the sham operated rats, the spinal cord of all the injured groups demonstrates the abundant activation of astrocytes (Fig. 4). The number of GFAP positive cells was 47.3 ± 2.5, 21.5 ± 3.7, 32.5 ± 5.2, and 29.3 ± 2.5 in group I-IV, respectively. The control group demonstrate a statistically significant increase in the number of the activated astrocytes compared to that of the other groups (P < 0.05). In most specimens, the activated astrocytes were located around the injured site and recruitment was also observed from the adjacent gray and white matter. The mean number of OX-42 positive cells in the × 200 magnified specimen was 21.2 ± 1.2, 5.3 ± 2.4, 18.6 ± 2.3, and 19.6 ± 1.5 in group I-IV, respectively. The injured group demonstrated a significantly elevated number of activated microglia. However, there was no statistical difference among the injured groups (Fig. 5).
Many diverse approaches for neuroprotection are being attempted as details of the mechanisms of secondary injury after SCI have been disclosed in the last few years. Through our previous study, we were able to verify the possibility of pregabalin as a neuroprotector after SCI (14). In that study, we only presented the anti-apoptotic and anti-inflammatory effects by histopathology. The present study used not only functional outcomes but also more detailed immunohistochemistry to demonstrate the feasibility of pregabalin on SCI. First, we analyzed the anti-apoptotic and anti-inflammatory effects by measuring the expression of caspase-3, bcl-2 and p38 MAPK. These molecules are known to be key players in the processes of apoptosis and inflammation (4, 15, 16, 21). Following spinal cord injury, neurons and glial cells undergo apoptosis; and the subsequent demyelination by apoptosis of oligodendrocyte is a key feature of secondary spinal cord injury. Previous studies have demonstrated that this phenomenon peaks at 48 hr and 7 days after SCI (1, 2, 15). And excitotoxicity is known to be one of the major pathomechanisms to elicit the apoptosis of the neuron and the supporting glial cells in the spinal cord injury. Presynaptic release, disrupted neurons, axons and/or glial cells, and especially astrocytes and oligodendrocytes, have been suggested as sources of glutamate in the gray matter and white matter. And subsequently these over-expressed excitotoxic neurotransmitters can induce the apoptosis by means of the direct damage of the mitochondria and the induction of apoptosis inducing enzymes including caspases. In this study, we observed a statistically significant reduction of caspase-3 expression in the pregabalin-treated group. The reduced expression of caspase-3 in the pregabalin group might be related to the pharmacologic action of this drug. Pregabalin may reduce extracellular glutamate concentration after SCI and this down-regulating effect of pregabalin was associated with rescuing cells from excitotoxicity. When we first designed this experiment, we decided to take the sample at the 7th day post-injury. This was based on the previous references that have demonstrated the apoptosis of neuronal damage after SCI persisted through the first week (2, 15). Although the present study demonstrated the statistical significant reduction of caspase-3 expression, we were not able to observe the significant differences in other proteins that are also related to apoptosis pathway. However, if we evaluate more of the acute stage samples (for example 24 hr after injury), we look forward to finding more statistical significant differences. We also measured phosphorylated p38 MAPK. p38 MAPK has been reported to have inflammation-modulating function at the level of transcription and translation (18, 19, 22). In the central nervous system, the glia, and mainly microglia, upregulate the expression and activation of p38 MAPK in pathologic conditions, and the subsequent activation of other cytokines such as IL-1, IL-6, TNF-α, and COX-2 (17, 23, 24). In the present investigation, although not statistically significant, the pregabalin treated group showed lower expression of phosphorylated p38 MAPK than that of the control group. This finding is further supported by the histologic differences noted with regard to the activation of microglia. During histopathologic evaluation, we focused on the observation of reactive astrocytosis and pregabalin effects on it. Astrocytes have been shown to regulate of the extracellular level of glutamate in synapses. This scavenger action on overexpressed glutamate is exerted by excitatory amino acid transporters (EAATs) and glutamine synthetase (25, 26). In response to injury in the central nervous system, astrocytes change their appearance and they undergo a characteristic hypertrophy of their processes with the upregulation of intermediate filaments that are composed of glial fibrillary acid protein, vimentin and nestin. These changes are known as gliosis and they are a suitable reaction to overcome the pathologic condition (25). Astrocyte proliferation after injury has been known to have both detrimental and beneficial effects (26, 27). Astrocyte scarring has long been considered as a major impediment to the regeneration of damaged axons. Chondroitin sulfate glycoproteins are well known key molecules in inhibiting the regeneration of axons (28). However, beneficial effects of astrocytes have recently been established as well. Astrocyte protective actions are exerted by restoring altered homeostasis (the ionic and neurotransmitter balance) and re-establishing the anatomical blood-brain barrier (29). At later stages, astrocytes can aid in regeneration by production of a variety of cytokines such as TGF-β, glial cell line derived neurotrophic factor, β-FGF and VEGF (29, 30). Some of these growth factors can facilitate oligodendrocyte precursor migration, proliferation and differentiation (30). Taking these backgrounds of the astrocyte into account, we carefully hypothesize that pregabalin may reduce excitotoxicity and minimize the need for astrocytic clearance of glutamate during the acute pathologic condition of SCI. Because astrocytes can provide positive effects, however, it remains to be seen if the lower proliferation of astrocytes can provide long-term benefit.
In summary, we were able to get more promising results that might support the feasibility of pregabalin following SCI. Anti-inflammatory and anti-apoptotic effects of pregabalin were repeatedly demonstrated by the histopathological and biochemical methods. Further studies are needed to determine the safety, ideal dosages, methods of administration and defining long-term results. We are encouraged, however, that pregabalin might be another neuroprotective agent for the treatment of spinal cord injury.
Figures and Tables
Fig. 1
Motor scores for the each group. The rats in all the groups show a sequential recovery of hindlimb function. At the postoperative 7th day, the pregabalin treated group show a more significant recovery compared to that of the other groups. Horizontal line at motor score means the score in the sham-operated rats.
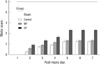
Fig. 2
(A) The effect of intraperitoneal pregabalin (30 mg/kg) on the expression of caspase-3 in contusive spinal cord injury. One-way ANOVA reveals significant differences between the groups for the expression of caspase-3 expression in the spinal cord tissue and the post hoc comparison (Bonferroni) test revealed that pregabalin treatment induced the least expression of caspase-3.This difference showed a statistical significance compared to the control group and the methylprednisolone treated group (P < 0.05, n = 10 for the experimental group, n = 3 for sham group). (B) The effect of intraperitoneal pregabalin (30 mg/kg) on the expression of bcl-2 in contusive spinal cord injury. One-way ANOVA test and post hoc analysis reveal no significant difference between the groups for the expression of bcl-2 expression in the spinal cord tissue (f = 0.52, P = 0.67, n = 10 for the experimental group, n = 3 for the sham group).
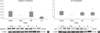
Fig. 3
The effect of intraperitoneal injection of pregabalin (30 mg/kg) on the expression of phosphorylated p38 MAPK in contusive spinal cord injury. The values were examined on post-injury day 7. The methylprednisolone and pregabalin treatment groups demonstrate a reduced expression of phosphorylated p38 MAPK as compared to that of the control group. However, ANOVA indicated no statistically significant difference (f = 0.65, P = 0.58, n = 10 for the experimental group, n = 3 for the sham group). The following Bonferroni post hoc analysis also indicated no statistical difference between the groups.
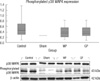
Fig. 4
Immunofluorescence detection of the activated astrocyte labeled with antibody GFAP. Abundant activation of astrocytes is observed in the most of the specimen of the injured groups. The control group demonstrate a higher number of activated astrocytes than that of the MP and the GP groups. The astrocytes are mostly located around the periphery of the injured site, and the astrocytes also show a tendency of recruitment from the surrounding gray and white matter (magnification × 200, scale bar 20 µm).
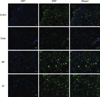
AUTHOR SUMMARY
Pregabalin as a Neuroprotector after Spinal Cord Injury in Rats: Biochemical Analysis and Effect on Glial Cells
Kee-Yong Ha, Eugene Carragee, Ivan Cheng, Soon-Eok Kwon, and Young-Hoon Kim
Pregabalin was used as a neuroprotective agent after spinal cord injury (SCI). Here we compared the effects of pregabalin with methylprednisolone. 50 rats were divided into four groups; the control group, the sham operated group, the methylprednisolone treated group, and the pregabalin-treated group. The pregabalin-treated group showed improved mean motor function score and the inclined plane score. Caspase-3 and phosphorylated p38MAPK expression of the pregabalin group were lower than that of the control group. Bcl-2 expression was not different between the control and the treated groups. In histopathological analysis, pregabalin treated group demonstrated less proliferation of microglia and astrocyte. The above data suggest neuroprotective effects of pregabalin.
References
1. Kwon BK, Tetzlaff W, Grauer JN, Beiner J, Vaccaro AR. Pathophysiology and pharmacologic treatment of acute spinal cord injury. Spine J. 2004. 4:451–464.
2. Dumont RJ, Okonkwo DO, Verma S, Hurlbert RJ, Boulos PT, Ellegala DB, Dumont AS. Acute spinal cord injury, Part I: pathophysiologic mechanisms. Clin Neuropharmacol. 2001. 24:254–264.
3. Fehlings MG, Baptiste DC. Current status of clinical trials for acute spinal cord injury. Injury. 2005. 36:Suppl 2. B113–B122.
4. Rowland JW, Hawryluk GW, Kwon B, Fehlings MG. Current status of acute spinal cord injury pathophysiology and emerging therapies: promise on the horizon. Neurosurg Focus. 2008. 25:E2.
5. Zhang Y, Bhavnani BR. Glutamate-induced apoptosis in primary cortical neurons is inhibited by equine estrogens via down-regulation of caspase-3 and prevention of mitochondrial cytochrome c release. BMC Neurosci. 2005. 6:13.
6. Xu GY, Liu S, Hughes MG, McAdoo DJ. Glutamate-induced losses of oligodendrocytes and neurons and activation of caspase-3 in the rat spinal cord. Neuroscience. 2008. 153:1034–1047.
7. Globus MY, Alonso O, Dietrich WD, Busto R, Ginsberg MD. Glutamate release and free radical production following brain injury: effects of posttraumatic hypothermia. J Neurochem. 1995. 65:1704–1711.
8. Park E, Velumian AA, Fehlings MG. The role of excitotoxicity in secondary mechanisms of spinal cord injury: a review with an emphasis on the implications for white matter degeneration. J Neurotrauma. 2004. 21:754–774.
9. Káradóttir R, Cavelier P, Bergersen LH, Attwell D. NMDA receptors are expressed in oligodendrocytes and activated in ischaemia. Nature. 2005. 438:1162–1166.
10. Joshi I, Taylor CP. Pregabalin action at a model synapse: binding to presynaptic calcium channel alpha2-delta subunit reduces neurotransmission in mice. Eur J Pharmacol. 2006. 553:82–88.
11. Tassone DM, Boyce E, Guyer J, Nuzum D. Pregabalin: a novel gamma-aminobutyric acid analogue in the treatment of neuropathic pain, partial-onset seizures, and anxiety disorders. Clin Ther. 2007. 29:26–48.
12. Fehrenbacher JC, Taylor CP, Vasko MR. Pregabalin and gabapentin reduce release of substance P and CGRP from rat spinal tissues only after inflammation or activation of protein kinase C. Pain. 2003. 105:133–141.
13. Million M, Wang L, Adelson DW, Roman F, Diop L, Taché Y. Pregabalin decreases visceral pain and prevents spinal neuronal activation in rats. Gut. 2007. 56:1482–1484.
14. Ha KY, Kim YH, Rhyu KW, Kwon SE. Pregabalin as a neuroprotector after spinal cord injury in rats. Eur Spine J. 2008. 17:864–872.
15. Keane RW, Kraydieh S, Lotocki G, Bethea JR, Krajewski S, Reed JC, Dietrich WD. Apoptotic and anti-apoptotic mechanisms following spinal cord injury. J Neuropathol Exp Neurol. 2001. 60:422–429.
16. Citron BA, Arnold PM, Haynes NG, Ameenuddin S, Farooque M, Santacruz K, Festoff BW. Neuroprotective effects of caspase-3 inhibition on functional recovery and tissue sparing after acute spinal cord injury. Spine (Phila Pa 1976). 2008. 33:2269–2277.
17. Horiuchi H, Ogata T, Morino T, Chuai M, Yamamoto H. Continuous intrathecal infusion of SB203580, a selective inhibitor of p38 mitogen-activated protein kinase, reduces the damage of hind-limb function after thoracic spinal cord injury in rat. Neurosci Res. 2003. 47:209–217.
18. Guo G, Bhat NR. p38alpha MAP kinase mediates hypoxia-induced motor neuron cell death: a potential target of minocycline's neuroprotective action. Neurochem Res. 2007. 32:2160–2166.
19. Genovese T, Esposito E, Mazzon E, Muià C, Di Paola R, Meli R, Bramanti P, Cuzzocrea S. Evidence for the role of mitogen-activated protein kinase signaling pathways in the development of spinal cord injury. J Pharmacol Exp Ther. 2008. 325:100–114.
20. Gale K, Kerasidis H, Wrathall JR. Spinal cord contusion in the rat; behavioral analysis of functional neurologic impairment. Exp Neurol. 1985. 88:123–134.
21. Austin JW, Fehlings MG. Molecular mechanisms of Fas-mediated cell death in oligodendrocytes. J Neurotrauma. 2008. 25:411–426.
22. Obata K, Yamanaka H, Kobayashi K, Dai Y, Mizushima T, Katsura H, Fukuoka T, Tokunaga A, Noguchi K. Role of mitogen-activated protein kinase activation in injured and intact primary afferent neurons for mechanical and heat hypersensitivity after spinal nerve ligation. J Neurosci. 2004. 24:10211–10222.
23. Nakahara S, Yone K, Sakou T, Wada S, Nagamine T, Niiyama T, Ichijo H. Induction of apoptosis signal regulating kinase 1 (ASK1) after spinal cord injury in rats: possible involvement of ASK1-JNK and -p38 pathways in neuronal apoptosis. J Neuropathol Exp Neurol. 1999. 58:442–450.
24. Kwak EK, Kim JW, Kang KS, Lee YH, Hua QH, Park TI, Park JY, Sohn YK. The role of inducible nitric oxide synthase following spinal cord injury in rat. J Korean Med Sci. 2005. 20:663–669.
25. Anderson CM, Swanson RA. Astrocyte glutamate transport: review of properties, regulation, and physiological functions. Glia. 2000. 32:1–14.
26. Williams A, Piaton G, Lubetzki C. Astrocytes--friends or foes in multiple sclerosis? Glia. 2007. 55:1300–1312.
27. Faulkner JR, Herrmann JE, Woo MJ, Tansey KE, Doan NB, Sofroniew MV. Reactive astrocytes protect tissue and preserve function after spinal cord injury. J Neurosci. 2004. 24:2143–2155.
28. Barritt AW, Davies M, Marchand F, Hartley R, Grist J, Yip P, McMahon SB, Bradbury EJ. Chondroitinase ABC promotes sprouting of intact and injured spinal systems after spinal cord injury. J Neurosci. 2006. 26:10856–10867.
29. Buffo A, Rolando C, Ceruti S. Astrocytes in the damaged brain: molecular and cellular insights into their reactive response and healing potential. Biochem Pharmacol. 2010. 79:77–89.
30. Rosenstein JM, Krum JM. New roles for VEGF in nervous tissue-beyond blood vessels. Exp Neurol. 2004. 187:246–253.