Abstract
Under conditions of Na+ channel hyperactivation with aconitine, the changes in action potential duration (APD) and the restitution characteristics have not been well defined in the context of aconitine-induced arrhythmogenesis. Optical mapping of voltage using RH237 was performed with eight extracted rabbit hearts that were perfused using the Langendorff system. The characteristics of APD restitution were assessed using the steady-state pacing protocol at baseline and 0.1 µM aconitine concentration. In addition, pseudo-ECG was analyzed at baseline, and with 0.1 and 1.0 µM of aconitine infusion respectively. Triggered activity was not shown in dose of 0.1 µM aconitine but overtly presented in 1.0 µM of aconitine. The slopes of the dynamic APD restitution curves were significantly steeper with 0.1 µM of aconitine than at baseline. With aconitine administration, the cycle length of initiation of APD alternans was significantly longer than at baseline (287.5 ± 9.6 vs 247.5 ± 15.0 msec, P = 0.016). The functional reentry following regional conduction block appears with the progression of APD alternans. Ventricular fibrillation is induced reproducibly at pacing cycle length showing a 2:1 conduction block. Low-dose aconitine produces arrhythmogenesis at an increasing restitution slope with APD alternans as well as regional conduction block that proceeds to functional reentry.
Aconitine, an alkaloid compound obtained from the plant Aconitum napellus, has well established arrhythmogenic effects on the heart. The arrhythmogenic effects of aconitine include various ventricular rhythm disorders such as premature ventricular contraction (PVC), ventricular tachycardia (VT), torsades de pointes (TdP), and ventricular fibrillation (VF) as well as supraventricular rhythm disorders, in a dose-dependent manner (1). Experimentally, aconitine-induced arrhythmias are considered to represent triggered activity of early as well as delayed activity afterdepolarization (2).
At the cellular level, aconitine has been shown to bind to Na+ channels and prolongs their open state favoring entry of a large quantity of Na+ into the cytosol; this is accompanied by Ca2+ overload via sequential activation of an electrogenic Na+-Ca2+ exchange system or L-type Ca2+ channels, and eventually induces triggered activity (3, 4). The repetitive discharges from a focal myocardial region, caused by enhanced triggered activity, have been considered the mechanism associated with aconitine-induced cardiac arrhythmias. However, in addition to the repetitive focal discharges caused by enhanced triggered activity, a single or a few PVCs observed after aconitine administration do not explain degeneration to more serious ventricular arrhythmias such as TdP and VF. Therefore, reentry and breakup of wave propagation might be involved more significantly in aconitine-induced arrhythmogenesis than previously thought. However, the role of action potential duration (APD) restitution has not been well studied in aconitine-induced arrhythmogenesis. Therefore, the purpose of the present study was to evaluate the role of APD restitution as a mechanism of degeneration into more serious ventricular rhythm disorders, induced by the administration of aconitine.
The animal experiments were reviewed and approved by the institutional animal care and use committee of the Yeungnam University Medical Center (YUMC-AEC2010-023).
Adult white rabbits (3.2-4.8 kg) were injected intravenously with heparin and anesthetized with ketamine and xylazine. After the heart was quickly removed, the ascending aorta was then immediately cannulated to a Langendorff apparatus and perfused with warm (36℃) oxygenated (95% O2, 5% CO2) Tyrode's solution (pH 7.4) at a rate of 30-40 mL/min to maintain a perfusion pressure at about 60 mmH2O. The composition of Tyrode's solution (in mM/L) was: NaCl 125, KCl 4.5, NaH2PO4 1.8, NaHCO3 24, CaCl2 1.8, MgCl2 0.5, dextrose 5.5, and albumin 50 mg/L in deionized water.
A pseudo-electrocardiogram (pseudo-ECG) was recorded by three electrodes placed widely apart with one pole on the right atrium and another pole on the left ventricle (LV), or with one pole on the right ventricle and the other on the LV; the last one was used for grounding. The pseudo-ECG was filtered using a low pass filter at 100 Hz and a high pass filter at 1-10 Hz, and digitized with MP100WSW (BIOPAC Systems, Inc., Goleta, CA, USA).
An optical mapping system was used for this study that included a charge coupled device camera (CA-D1-0128T, Dalsa Inc., Ontario, Canada). The voltage fluorescence signals were acquired through a 710 nm long-pass filter at 1.33 msec/frame. A voltage sensitive dye RH237 (Di-4-ANNEPS, Sigma-Aldrich®, St. Louis, MO, USA) was added to the perfusate (10-20 µL of 1 mg/mL solution dissolved in dimethyl sulfoxide (DMSO, Sigma-Aldrich) with an LED light source.
The anterior epicardial surface of the LV was used in eight rabbits for the experiments. The APD was measured from the onset of the QRS complex (time zero) to a 90% reduction of the fluorescence signal amplitude. Stimulus artifact was used as time zero for measuring the paced beats.
The hearts were perfused with Tyrode's solution for at least 15 min before any experimental intervention. Cytochalasin D of 5 µM (Sigma-Aldrich) was used as an electromechanical uncoupler. Electrical stimuli (2 msec pulse duration, twice the diastolic threshold current) were delivered through a bipolar electrode at the LV apex. Mapping was performed both during sinus rhythm and during LV pacing. The steady-state pacing cycle length started at 300 msec and was progressively shortened until loss of capture occurred. Following the above pacing protocol, extrastimuli (S2) were delivered that gradually shortened the coupling intervals to the point of capture loss, preceded by basal incremental pacing (S1; 300 msec, 8 beats). Aconitine was dissolved in normal saline before it was added to the Tyrode's solution to achieve a concentration of 0.1 and 1.0 µM. After collecting baseline data, aconitine was administrated sequentially. The pacing and mapping protocols were repeated at these concentrations.
APD90 was used for the statistical analyses. Paired t-test was used for the numerical values and those were presented as the mean ± SD. Because it was not possible at times to differentiate the U wave from the T wave, on the pseudo-ECG, the QT interval was measured from the onset of the QRS to the end of the T-U wave. A repeated measurement method using a general linear model analysis was used to compare the means of the QT intervals, and APD90. A P value of less than 0.05 was considered to indicate statistical significance.
The appearance of triggered activity was found to be dependent on the concentration of aconitine in the Langendorff perfusate. In the absence of electrical stimulation, an intrinsic narrow QRS rhythm continued to be observed with a concentration of 0.1 µM of aconitine without producing triggered activity, as at baseline. After completing the experiments described above, the concentration of the perfusate was increased tenfold to 1.0 µM aconitine; this resulted in aconitine-induced triggered activity.
The present study was performed using a 0.1 µM concentration of aconitine in order to assess the reentry and breakup of wave propagation caused by the effects of aconitine on the myocardium while minimizing aconitine-induced triggered activity.
After administration of 0.1 µM aconitine, the heart rate was gradually accelerated with a prolonged QT interval compared to baseline. However, aconitine-induced triggered activity was not observed in the ventricular myocardium over 30 min during the experiments. The aconitine-induced triggered activity was observed after 1.0 µM of aconitine was administered (Fig. 1). At first, there was a more prolonged QT interval with a hump, which was considered an early afterdepolarization (EAD), and then isolated PVCs were observed. The documented rhythm gradually progressed to ventricular bigeminy and VT. VT was accelerated with T-wave alternans and then degenerated to polymorphic VT such as TdP and VF. Similar sequences of rhythm development were observed in all eight preparations after administration of aconitine.
Aconitine prolonged the APD as well as the QT interval (Table 1). During steady-state pacing (incremental pacing), the cycle length of initiation of APD alternans and 2:1 conduction block were longer with aconitine administration compared to the baseline. In addition, the capture failure (block) of a single extrastimulus (S2), followed by 300 msec incremental pacing, occurred with longer S1-S2 intervals after the administration of aconitine.
The left panel of Fig. 2 shows that APD alternans occurred with the gradual shortening of the pacing cycle length and finally progressed to a merging of a short APD over a long APD, as a pair. The right panel, with 0.1 µM aconitine, shows that these phenomena appeared earlier, that is at a longer cycle length, and demonstrated a 2:1 block. With the cycle lengthening presenting with a 2:1 block, the steady-state pacing easily induced VF; whereas at baseline APD alternans was present without 2:1 block at that cycle length.
Optical mapping was performed on the anterior epicardial surface of the left ventricle of the extracted rabbit hearts. The patterns of wave propagation are illustrated in Fig. 3 after administration of 1 µM aconitine; the apex of the left ventricle was paced with the cycle length showing an intermittent 2:1 block.
The upper panel of Fig. 3 shows the paced wavefront propagated sequentially from the apex to the base (also from point "a" to point "b") while the next wave failed to propagate (2:1 block). However, the middle panel demonstrates the regional conduction block of the wavefront toward point "a" after a few incidental 1:1 conductions of paced beats; whereas the wavefront toward point "b" propagated to the base and turned around to progress toward point "a" initiating the conduction of fibrillations. Therefore, the regional conduction block was responsible for VF that could be reproducibly induced around the pacing cycle length with a 2:1 block.
A steady-state pacing was induced in all hearts (n = 8) to obtain dynamic APD restitution curves with a pacing cycle length from 300 msec to a 2:1 conduction block cycle length. The average for the minimum pacing cycle lengths where 1:1 conductions were achieved was significantly longer with aconitine administration than at baseline (246.0 ± 39.7 msec vs 194.0 ± 13.4 msec, P = 0.035) while each pacing interval was gradually shortened by 10 msec (Table 1). In addition, APD alternans occurred at a significantly longer average pacing cycle length with aconitine administration (287.5 ± 9.6 msec vs 247.5 ± 15.0 msec, P = 0.016). Discordant APD alternans was not observed in all cases after aconitine administration and was noted in two cases at baseline.
Fig. 4 is a representative example of the dynamic APD restitution curve, which is more predictive of persistent APD alternans and VF inducibility than standard restitution curves (5, 6). The left panel of Fig. 4 shows the results obtained from the baseline study. As the pacing cycle length was progressively shortened, the diastolic interval (DI) and APD decreased according to the pacing cycle length in a nonlinear pattern. The dynamic restitution curve shows a parabolic pattern: At longer DIs, the slope of the dynamic restitution curve was nearly flat, whereas the slope of the dynamic restitution curve was steeper with shorter DIs. The right panel of Fig. 4 shows the results obtained with aconitine administration. The dynamic restitution curve shows a very steep slope (> 1) throughout with no initial flat portion.
The results of the present study showed that reentry following a functional regional block of wave propagation might be a mechanism associated with aconitine-induced arrhythmogenesis, occurring before the overt manifestations of triggered activity, in other words, with low concentrations of added aconitine (0.1 µM). In addition, the regional block was noted to concur, with maximal APD alternans, just before 2:1 conduction block. Therefore, the progression of APD alternans is likely to play a pivotal role in causing a functional regional block of wave propagation.
Aconitine has been shown to prolong the QT interval and APD consistent with the results of this study. The type 2 receptor site on voltage-gated Na+ channels is a binding site for aconitine that increases Na+ channel permeability and shifts the action potential towards a more hyperpolarized state in voltage-clamp studies (7, 8). Thereby causing a persistent activation of the Na+ channels, as well as the prolongation of the QT interval and APD, which become more refractory to subsequent stimulation (1, 9). One explanation is that the 2:1 conduction block occurred at a longer pacing cycle length with aconitine compared to the baseline. The EAD associated with aconitine administration can be explained by an increased window for Na+ current and delayed inactivation of the Na+ channel. In addition, the prolongation of the QT interval results from increased Na+ influx and the accumulation in the cytosol leads to the appearance of EAD through augmented activation of the Na+-Ca2+ exchange system (10). A few recent works have suggested that aconitine blocks HERG and Kv1.5 potassium channels (11), and this compound has a time- and concentration-dependent blocking effect on the ultra-rapid delayed rectifier potassium channel (IKur) in H9c2 myogenic cells and in ventricular myocytes of neonatal rats (12). This action may contribute to APD prolongation along with appearance of EAD, and could be another mechanism underlying aconitine-induced arrhythmia. However, we can not specify the contribution of possible blocking effect on potassium channel by aconitine in aconitine-induced arrhythmia observed in this study.
In the present study, the QT interval was prolonged depending on the dose of aconitine used, and was sufficiently prolonged so that a hump was noted on the terminal portion of the QT interval with a high-dose of aconitine (1.0 µM). Isolated PVC's originated from the same location where the humps were observed. Therefore, the humps are thought to represent the EAD as triggered activity; this has been described in previous studies (13, 14). After several minutes, the PVC's spontaneously degenerated to VT with progressive acceleration of the cycle length, and finally to VF.
With a low-dose of aconitine (0.1 µM), a PVC or hump, indicating the development of triggered activity, were never observed on the pseudo-ECG, or spontaneous VF. However, the steady-state pacing could easily induce VF with a certain pacing cycle length showing an intermittent 2:1 conduction block; however, VF was never induced at baseline. Therefore, the induction mechanism associated with VF, with low-dose aconitine, is not likely to be dependent on the triggered activity well known to be associated with aconitine-induced arrhythmogenesis.
APD restitution is defined as the relationship of APD to its preceding DI over a range of cycle lengths (15, 16). In other words, the longer a preceding DI is, the more prolonged APD follows and the converse. The APD restitution curve roughly represents a single-exponential time course. The steepness of the slope of the APD restitution curve, which is measured as a ratio of the change of APD over the change of DI, was associated with APD alternans and maintenance of arrhythmias (17): When the slope of the APD restitution curve is > 1, APD alternans becomes exaggerated and can induce cardiac arrhythmia through increasing electrical inhomogeneity. In the present study, the slope of the APD restitution curve steepened after the administration of low-dose aconitine, which resulted in the appearance of APD alternans at longer pacing cycle lengths than at baseline. Similarly, fibrillatory conduction was easily observed at the longer pacing cycle lengths.
In the present study, the initiation of fibrillatory conduction (VF) showed a repetitive pattern that always began with a regional conduction block of short AP following maximal APD alternans. This phenomenon can be explained as during the progression of APD alternans, the subtle oscillation of APD in every long-short action potential pair (temporal and spatial heterogeneity of APD alternans) incidentally hinders the conduction of the next short AP, when the preceding long AP oscillated to slightly prolong the refractory period so that regional conduction block occurred. In addition, the heterogeneity of APD alternans is likely to be enhanced when the Na+ channels is affected by aconitine.
Discordant APD alternans, which is more susceptible to inducing VF than concordant APD alternans, was not observed during steady-state pacing with low-dose aconitine administration, whereas two were observed at baseline. The findings of previous studies (18, 19) show that once discordant APD alternans occurs, the dispersion of refractoriness (electrogenic heterogeneity) is significantly amplified to produce a favorable substrate for the initiation of reentry. While pacing from the same site, as in the experimental protocol of this study, the transition from concordant to discordant APD alternans requires that the pacing cycle length be short enough to engage conduction velocity restitution (18). Aconitine prolonged the APD, and thereby the pacing cycle length was not allowed to be short enough to induce discordant APD alternans in this study. Therefore, an increase in the restitution slope causing concordant APD alternans appears to have been the main cause of arrhythmogenesis, as well as regional conduction block.
With low-dose aconitine, where the triggered activity did not occur overtly, an increased heart rate is likely to contribute to arrhythmogenesis. In other words, even without considering high-dose aconitine intoxication by accidental ingestion or herbal prescription, low-dose aconitine that are considered to be safe in traditional herbal medicine might have harmful arrhythmogenic effects in a patient who develops atrial fibrillation with an uncontrolled rapid ventricular response.
The limitations of this study include that the APD recordings were only obtained from the epicardial surface; the transmural electrical gradient and excitation of endocardial Perkinje fibers were not assessed. The findings of this study showed that an increase in the APD restitution slope with regional conduction block was central to induction of VF with low-dose aconitine. Further study is needed using whole layers of heart that might reveal more detailed mechanisms of the arrhythmogenic effects of aconitine.
In conclusion, despite the engagement of triggered activity, low-dose aconitine can cause arrhythmogenesis by steepening of the restitution slope with APD alternans and regional conduction block that finally proceeds to functional reentry and break-up of wave propagation.
Figures and Tables
Fig. 1
Pseudo-ECG findings according to the changes of aconitine concentration. From baseline (A), the heart rates were gradually accelerated depending on the concentration of aconitine (B, C) with sequential prolongation of the QT interval. With 1.0 µM of aconitine (C), humps at the terminal portion of the T-waves (open arrow) were observed suggesting early afterdepolarization representing PVCs (D: closed arrow), which progressed to ventricular bigeminy (E) and VT (F: lined arrows point the alternans of T-wave). Finally VF was initiated (G).
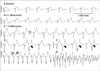
Fig. 2
Effects of aconitine on the changes of epicardial membrane potential. Administration of 0.1 µM aconitine (B) caused APD alternans at a longer pacing cycle length, with APD prolongation compared to the baseline study (A). The arrows indicate intermittent 2:1 conduction block induced VF (lower part of B).
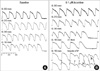
Fig. 3
The patterns of wave propagation during initiation of VF after administration of 1 µM aconitine. The panel (A) shows the paced wave propagated from the LV apex to the base (A to D). The panel (B) shows that the paced wave was not propagated toward the "a" direction (regional block) whereas the wave propagated toward the "b" direction initiated VF (E to I). The electrogram (C) shows the second paced wave (dotted arrow) failed to conduct totally while the forth wave succeeded and the sixth wave was conducted with the regional block C as shown in the middle panel.
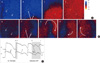
AUTHOR SUMMARY
Role of the Alternans of Action Potential Duration and Aconitine-Induced Arrhythmias in Isolated Rabbit Hearts
Byung-Chun Jung, Sang-Hee Lee, Yong-Keun Cho, Hyoung-Seob Park, Yoon-Nyun Kim, Young-Soo Lee and Dong-Gu Shin
Aconitine, a component of herbal medicine, reportedly induce ventricular arrhythmia at high concentration. Our present study shows that, with low-dose aconitine, an increased heart rate is likely to contribute to arrhythmogenesis: in other words, low-dose amounts that are recommended to be safe in traditional herbal medicine might have harmful arrhythmogenic effects when a patient develops atrial fibrillation with an uncontrolled rapid ventricular response.
References
1. Friese J, Gleitz J, Gutser UT, Heubach JF, Matthiesen T, Wilffert B, Selve N. Aconitum sp. alkaloids: the modulation of voltage-dependent Na+ channels, toxicity and antinociceptive properties. Eur J Pharmacol. 1997. 337:165–174.
2. Kimura I, Makino M, Takamura Y, Islam MA, Kimura M. Positive chronotropic and inotropic effects of higenamine and its enhancing action on the aconitine-induced tachyarrhythmia in isolated murine atria. Jpn J Pharmacol. 1994. 66:75–80.
3. Catterall WA. Structure and function of voltage-sensitive ion channels. Science. 1988. 242:50–61.
4. Chiang CE, Roden DM. The long QT syndromes: genetic basis and clinical implications. J Am Coll Cardiol. 2000. 36:1–12.
5. Koller ML, Riccio ML, Gilmour RF Jr. Dynamic restitution of action potential duration during electrical alternans and ventricular fibrillation. Am J Physiol. 1998. 275:H1635–H1642.
6. Banville I, Chattipakorn N, Gray RA. Restitution dynamics during pacing and arrhythmias in isolated pig hearts. J Cardiovasc Electrophysiol. 2004. 15:455–463.
7. Wright SN. Comparison of aconitine-modified human heart (hH1) and rat skeletal (µ1) muscle Na+ channels: an important role for external Na+ ions. J Physiol. 2002. 538:759–771.
8. Chan TY. Aconite poisoning. Clin Toxicol. 2009. 47:279–285.
9. Arita J, Xue XY, Aye NN, Fukuyama K, Wakui Y, Niitsu K, Maruno M, Siying C, Hashimoto K. Antiarrhythmic effects of an aconitine-like compound, TJN-505, on canine arrhythmia models. Eur J Pharmacol. 1996. 318:333–340.
10. Nattel S, Khairy P, Schram G. Arrhythmogenic ionic remodeling: adaptive responses with maladaptive consequences. Trends Cardiovasc Med. 2001. 11:295–301.
11. Li Y, Tu D, Xiao H, Du Y, Zou A, Liao Y, Dong S. Aconitine blocks HERG and Kv1.5 potassium channels. J Ethnopharmacol. 2010. 131:187–195.
12. Wang YJ, Chen BS, Lin MW, Lin AA, Peng H, Sung RJ, Wu SN. Time-dependent block of ultrarapid-delayed rectifier K+ currents by aconitine, a potent cardiotoxin, in heart-derived H9c2 myoblasts and in neonatal rat ventricular myocytes. Toxicol Sci. 2008. 106:454–463.
13. Cranefield PF. Action potentials, afterpotentials, and arrhythmias. Circ Res. 1977. 41:415–423.
14. Adaniya H, Hayami H, Hiraoka M, Sawanobori T. Effects of magnesium on polymorphic ventricular tachycardias induced by aconitine. J Cardiovasc Pharmacol. 1994. 24:721–729.
15. Nolasco JB, Dahlen RW. A graphic method for the study of alternation in cardiac action potentials. J Appl Physiol. 1968. 25:191–196.
16. Elharrar V, Surawicz B. Cycle length effect on restitution of action potential duration in dog cardiac fibers. Am J Physiol. 1983. 244:H782–H792.
17. Garfinkel A, Chen PS, Walter DO, Karagueuzian HS, Kogan B, Evans SJ, Karpoukhin M, Hwang C, Uchida T, Gotoh M, Nwasokwa O, Sager P, Weiss JN. Quasiperiodicity and chaos in cardiac fibrillation. J Clin Invest. 1997. 99:305–314.
18. Qu Z, Garfinkel A, Chen PS, Weiss JN. Mechanisms of discordant alternans and induction of reentry in simulated cardiac tissue. Circulation. 2000. 102:1664–1670.
19. Wilson LD, Rosenbaum DS. Mechanisms of arrhythmogenic cardiac alternans. Europace. 2007. 9:vi77–vi82.