Abstract
We performed an immunohistochemical study on the estrogen receptor alpha (ER-α) distribution in the cerebellum of a human neonate with multiple congenital anomalies, that had been acquired during autopsy. Although the exact pathology in the brain was not clearly elucidated in this study, an unidentified stressful condition might have induced the astrocytes into reactive states. In this immunohistochemical study on the neonatal cerebellum with multiple congenital anomalies, intense ER-α immunoreactivities (IRs) were localized mainly within the white matter even though ER-α IRs were known to be mainly localized in neurons. Double immunohistochemical staining showed that ER-α IR cells were reactive astrocytes, but not neurons. Interestingly, there were differences in the process length among the reactive astrocytes showing ER-α IRs. Our quantitative data confirmed that among the glial fibrillary acidic protein (GFAP)-expressing reactive astrocytes, the cells exhibiting intense ER-α IRs have much longer cytoplasmic processes and relatively weaker GFAP IRs. Taken together, the elongated processes of reactive astrocytes might be due to decreased expression of GFAP, which might be induced by elevated expression of ER-α even though the elucidation of the exact mechanism needs further studies.
Since estrogen plays its role through its binding to estrogen receptors (ERs), ER-α and ER-β, the morphological information on the distribution of ERs became imperative in understanding the effect of estrogen on various intracellular molecular dynamics. Among various organs exhibiting ER immunoreactivities (IRs), the central nervous system (CNS) was intensively investigated because estrogen was recently revealed to be crucial for neuroprotective functions (1-3). These novel functions of estrogen in the CNS were reported to be related to the presence of ERs within the cytoplasm (4-6), which were quite different from those of previously recognized ERs in the nuclei of cells (7-10). Among the neural cells showing possible estrogen-related neuroprotective effect, astrocytes were important candidates for the function because ER-α IRs were shown to be intensely localized within astrocytes of affected brain regions in some pathological states. ER-α was exclusively localized within astrocytes exhibiting glial fibrillary acidic protein (GFAP) or vimentin IRs, the markers for reactive astrocytes against various injuries to the brain (11). In this regard, reactive astrocytes could be a direct target for estrogens working through its binding to ERs within the cells. Taken together, ER-α IRs were mainly observed in neurons in normal state but ER-α IRs shifted into astrocytes in some pathological states.
However, we found out that ER-α IRs were expressed differentially even among GFAP-positive astrocytes, suggesting that the expression of ER-α IRs might affect the expression of GFAP and even the process elongation of astrocytes. The findings of this study also suggests that the expression pattern of ER-α IRs in reactive astrocytes under neuropathlogical conditions could be more complicated than have been suggested by previous studies.
The subject of the current case, human female neonate, was born at 41 weeks of gestation by cesarean section. After she died on the sixth postnatal day, autopsy was performed. The postmortem examination showed various anomalies of cardiovascular, digestive, and urogenital systems. The anomalies included patent ductus arteriosus, pyrolic stenosis of the stomach, exstrophy of the urinary bladder, urethral atresia, omphalocele-containing urinary bladder, vaginal atresia (complete obstruction), and anorectal agenesis with rectovesical fistulas. However, no specific morphological anomalies were detected in the brain by gross and microscopic examinations. The cerebellar tissue sample was taken and immersed overnight in the freshly prepared 4% paraformaldehyde (PFA)-containing phosphate-buffered saline (PBS). Subsequent tissue processing and immunohistochemical study were performed in accordance with our previous method (12). The primary antibodies used in the present study were rabbit anti-human ER-α antibody (1:1,000, Santa Cruz, CA, U.S.A.), mouse anti-GFAP antibody (1:500, Biogenix, CA, U.S.A.), mouse anti-OX42 antibody (MCA275G, SeroTec, U.K.), and mouse monoclonal anti-165 kDa neurofilament (1:500, Developmental Studies Hybridoma Bank, Iowa, U.S.A.) antibody. The secondary antibodies used for fluorescence microscope were alexa fluor 555, and alexa fluor 488 antibodies against rabbit and mouse (Molecular Probes, Eugene, OR). DAPI (1:5,000, Molecular Probes) was used for nucleus staining.
Intense ER-α IRs were observed mainly within the white matter than those in the gray matter including granular and molecular layers (Fig. 1A, B). To rule out the possibility that ER-α IRs were expressed by cerebellar neurons, double immunohistochemical staining was performed with anti-ER-α and anti-165 kDa neurofilament antibodies. Anti-165 kDa neurofilament IRs were not co-localized in the cell processes exhibiting ER-α IRs (Fig. 1C).
The types of ER-α-positive cells were also identified by a double immunohistochemical study with both anti ER-α and GFAP antibodies. The majority of the cells expressing ER-α IRs showed GFAP IRs (Fig. 2A to C). And there might be some differences in the process length among the reactive astrocytes showing both GFAP and ER-α IRs. The reactive astrocytes with intense ER-α IRs exhibited relatively long processes and relatively weak GFAP IRs (Fig. 3). To confirm this pattern with a statistical method, the reactive astrocytes exhibiting GFAP IRs were classified into two groups; the one with long processes (>20 µm of process length) and the other with short processes (below 20 µm of process length). The intensities of GFAP IRs and ER-α IRs were also differentially measured in perinuclear or process areas within the cells in each group. The signal intensities were measured in 100 different photographed fields using Image program (Scion Corporation, Frederick, MD, U.S.A.). All densitometric data were expressed as mean density, i.e. the average of the gray values of all pixels within the measured area (maximum level is 256). The acquired data were analyzed by Student t-test, and a p value less than 0.01 was deemed statistically significant. The final data were presented as means±S.D. In this quantitative study, we statistically confirmed that GFAP immunoreactive astrocytes with long processes expressed much intense ER-α IRs than those with short processes (Fig. 4). ER-α IR cells were not reactive for a microglia marker, OX-42 (Fig. 5).
It was well known that ER-α was mainly expressed by neurons in the brain without any neuropathological findings (13-15). On the other hand, we showed that much intense ER-α IRs were observed mainly within astrocytes of the white matter than neurons in the gray matter of the neonatal cerebellum with multiple congenital anomalies of the present case. Since ER-α was known to be localized within astrocytes in cases of excitotoxic injury or Alzheimer disease (11, 16), the current study could be well matched with previous reports. Therefore, even though the exact neuropathology of the current case was not clearly revealed in this study, the abnormal expression pattern of ER-α, the decreased intensities of neuronal ER-α IRs and increased intensities of ER-α IRs in astrocytes, could suggest that the neonatal brain might be under abnormal neurological conditions, which might finally induce the astrocytes into reactive states.
Though we could not be sure if estrogen directly affect the elongation of the astrocyte processes, it should be also considered that estrogen might inhibit GFAP transcription in astrocytes, which in turn down-regulate the proliferation of reactive astrocytes after injury (17).
As to the another possible cause of process elongation of ER-α-positive reactive astrocytes, the previous report on the effect of estrogen on promoting neurite sprouting under nerve growth factor (NGF) stimulation (18) deserves consideration. According to them, estrogen treatment markedly enhanced NGF-stimulated neurite outgrowth of PC12 cells that were stably transfected with the full-length rat ER-α gene (PCER). Since the estrogen effect on the neurite sprouting was thought to be correlated with the modulated expression of various cytoskeletal proteins (18), we could suggest the possibility that estrogen might also affect the cytoskeleton dynamics through ER-α during the process elongation of reactive astrocytes.
Although we could not clearly elucidate the cause of process elongation in ER-α IR astrocytes currently, the present study demonstrated for the first time that among reactive astrocytes under abnormal conditions, cells that express ER-α intensely had much longer processes, which might have been caused by the decrease in GFAP expression or inducing effect of estrogen on cytoskeleton dynamics. However, since our findings were from a single case under unknown pathological stimuli, our hypothesis should be considered as a tentative suggestion until the general mechanism related to the current changes could be further elucidated in the forthcoming animal experiments.
Figures and Tables
Fig. 1
(A) and (B) Estrogen receptor alpha (ER-α) immunoreactivities (IRs) in the cerebellum of a human neonate with multiple congenital anomalies. (B) is the magnified image of (A). W, white matter; Gr, granular layer; M, molecular layers. Yellow arrows indicate ER-α IR cells exhibiting extended processes around all directions. (C) Double immunohistochemical study using antineurofilament and anti-ER-α antibodies in the cerebellum of the human neonate with multiple congenital anomalies. Neurofilament IRs (green, indicated by white arrows); ER-α IRs (red); Nuclei stained with DAPI (blue). Scale Bars, 40 µm.
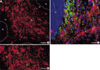
Fig. 2
Double immunohistochemical study on the cell type of estrogen receptor-alpha (ER-α) immunoreactivity (IR) cells in the cerebellum of a human neonate with multiple congenital anomalies. (A) for anti-glial fibrillary acidic protein (GFAP); (B) for anti-ER-α antibodies; (C) is the merged image of (A) and (B). Intensely ER-α IR astrocytes with longer processes were encircled by white dotted line. Astrocytes with weaker ER-α IRs were encircled by a yellow dotted line. Scale bars, 20 µm.
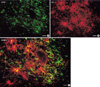
Fig. 3
Comparison of reactive astrocytes with short (A, B) and long processes (C, D). (A) and (C) for glial fibrillary acidic protein (GFAP) immunoreactivities (IRs). (B) and (D) for estrogen receptor alpha (ER-α) IRs. Scale bars, 20 µm.
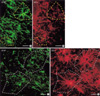
Fig. 4
Quantitative analysis on the relationship between the process length and the intensities of ER-α or GFAP IRs of reactive astrocytes. A p value of less than 0.01 was deemed statistically significant.
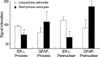
Fig. 5
Double immunohistochemical study using (A) OX-42 and (B) estrogen receptor alpha (ER-α) antibodies. (C) is the merged image of (A) and (B). (D) is merged image of (C) and DAPI stained image. ER-α immunoreactivity (IR) cells were not microglia because OX-42 IR microglia (indicated by yellow arrows) did not exhibit ER-α IRs. Scale Bars, 20 µm.
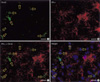
ACKNOWLEDGEMENTS
Mouse monoclonal anti-neurofilament 165 kDa antibody developed by Dr. Thomas M. Jessell and Jane Dodd was obtained from the Developmental Studies Hybridoma Bank established by the NICHD and maintained by the University of Iowa, Department of Biological Sciences, Iowa City, IA 52242.
References
1. McEwen BS. Clinical review 108: the molecular and neuroanatomical basis for estrogen effects in the central nervous system. J Clin Endocrinol Metab. 1999. 84:1790–1797.
2. Solum DT, Handa RJ. Localization of estrogen receptor alpha (ER alpha) in pyramidal neurons of the developing rat hippocampus. Brain Res Dev Brain Res. 2001. 128:165–175.
3. Manthey D, Behl C. From structural biochemistry to expression profiling: neuroprotective activities of estrogen. Neuroscience. 2006. 138:845–850.


4. Lee E, Shin DH, Cha CI, Lee YS. Immunohistochemical study on the distribution of estrogen receptor-alpha in the hippocampus of the normal aged rat. J Korean Geriatr Soc. 2002. 6:233–238.
5. Kalesnykas G, Roschier U, Puolivali J, Wang J, Miettinen R. The effect of aging on the subcellular distribution of estrogen receptor-alpha in the cholinergic neurons of transgenic and wild-type mice. Eur J Neurosci. 2005. 21:1437–1442.


6. Kalita K, Szymczak S, Kaczmarek L. Non-nuclear estrogen receptor beta and alpha in the hippocampus of male and female rats. Hippocampus. 2005. 15:404–412.
7. Perrot-Applanat M, Logeat F, Groyer-Picard MT, Milgrom E. Immunocytochemical study of mammalian progesterone receptor using monoclonal antibodies. Endocrinology. 1985. 116:1473–1484.


8. Loy R, Gerlach JL, McEwen BS. Autoradiographic localization of estradiol-binding neurons in the rat hippocampal formation and entorhinal cortex. Brain Res. 1988. 467:245–251.


9. Liposits Z, Kallo I, Coen CW, Paull WK, Flerko B. Ultrastructural analysis of estrogen receptor immunoreactive neurons in the medial preoptic area of the female rat brain. Histochemistry. 1990. 93:233–239.


10. Perez SE, Chen EY, Mufson EJ. Distribution of estrogen receptor alpha and beta immunoreactive profiles in the postnatal rat brain. Brain Res Dev Brain Res. 2003. 145:117–139.
11. Garcia-Ovejero D, Veiga S, Garcia-Segura LM, Doncarlos LL. Glial expression of estrogen and androgen receptors after rat brain injury. J Comp Neurol. 2002. 450:256–271.
12. Shin DH, Lim HS, Cho SK, Lee HY, Lee HW, Lee KH, Chung YH, Cho SS, Ik Cha C, Hwang DH. Immunocytochemical localization of neuronal and inducible nitric oxide synthase in the retina of zebrafish, Brachydanio rerio. Neurosci Lett. 2000. 292:220–222.


13. Simerly RB, Chang C, Muramatsu M, Swanson LW. Distribution of androgen and estrogen receptor mRNA-containing cells in the rat brain: an in situ hybridization study. J Comp Neurol. 1990. 294:76–95.


14. Donahue JE, Stopa EG, Chorsky RL, King JC, Schipper HM, Tobet SA, Blaustein JD, Reichlin S. Cells containing immunoreactive estrogen receptor-alpha in the human basal forebrain. Brain Res. 2000. 856:142–151.
15. Milner TA, McEwen BS, Hayashi S, Li CJ, Reagan LP, Alves SE. Ultrastructural evidence that hippocampal alpha estrogen receptors are located at extranuclear sites. J Comp Neurol. 2001. 429:355–371.


16. Lu YP, Zeng M, Hu XY, Xu H, Swaab DF, Ravid R, Zhou JN. Estrogen receptor alpha-immunoreactive astrocytes are increased in the hippocampus in Alzheimer's disease. Exp Neurol. 2003. 183:482–488.
17. Stone DJ, Song Y, Anderson CP, Krohn KK, Finch CE, Rozovsky I. Bidirectional transcription regulation of glial fibrillary acidic protein by estradiol in vivo and in vitro. Endocrinology. 1998. 139:3202–3209.
18. Gollapudi L, Oblinger MM. Estrogen effects on neurite outgrowth and cytoskeletal gene expression in ERalpha-transfected PC12 cell lines. Exp Neurol. 2001. 171:308–316.