Abstract
Several reports have described aberrant methylation in various types of human cancers. However, the interpretation of methylation frequency in various human cancers has some limitations because of the different materials and methods used for methylation analysis. To gain an insight into the role of DNA hypermethylation in human cancers and allow direct comparison of tissue specific methylation, we generated methylation profiles in 328 human cancers, including 24 breast, 48 colon, 61 stomach, 48 liver, 37 larynx, 24 lung, 40 prostate, and 46 uterine cervical cancer samples by analyzing CpG island hypermethylation of 13 genes using methylation-specific PCR. The mean numbers of methylated genes were 6.5, 4.4, 3.6, 3.4, 3.1, 3.1, 3.1, and 2.1 in gastric, liver, prostate, larynx, colon, lung, uterine cervix, and in breast cancer samples, respectively. The number of genes that were methylated at a frequency of more than 40% in each tumor type ranged from nine (stomach) to one (breast). Generally genes frequently methylated in a specific cancer type differed from those methylated in other cancer types. The findings indicate that aberrant CpG island hypermethylation is a frequent finding in human cancers of various tissue types, and each tissue type has its own distinct methylation pattern.
Cytosine is methylated in the context of CpG dinucleotides, and most CpGs are methylated except for those on CpG islands. CpG islands are DNA segments of 0.5 to 2.5 kb in size, which are rich in cytosine-guanine dinucleotides and are often located in the promoter or 5' exonal sequences of genes. About half of human genes harbor CpG islands in their promoter sequences and these CpG islands are normally protected from methylation (1-3). Although the cause is unclear, promoter CpG islands can be methylated in relation to cancerization. Methylated CpG islands are bound with methylated cytosine binding proteins, which recruit histone deacetylase (4, 5). Moreover, histone modifications, such as, deacetylation and methylation, cause chromatin to adopt a closed structure, which cannot be accessed by transcription factors, which results in gene silencing (6, 7).
Hypermethylation of promoter CpG islands is an important mechanism of gene inactivation for tumorigenesis and acts as an alternative to gene mutations like point mutations or intragenic deletions (8, 9). Hypermethylation of promoter CpG islands in tumor suppressor genes or tumor-related genes is a common finding in human cancers, regardless of tissue type. However, tissue-to-tissue variations exist in CpG island methylation with regard to the type of gene methylated, the methylation frequency of a specific gene, and the overall methylation extent (10, 11). Esteller et al. (11) analyzed 15 major tumor types for the methylation statuses of 12 genes and demonstrated that the profiles of CpG island methylation for genes is cancer type-dependent, and thus, CpG island methylation provides tumor-type and gene-specific profiles. However, in this study, all 12 genes were not studied in each types of cancer. In particular, information available about methylation profiles in gastric cancer and hepatocellular carcinoma, which are common in Eastern Asian countries (12), is limited by the small number of cases included and genes studied, and furthermore, uterine cervical cancer was not included in the study.
In the present study, we analyzed 8 tissue types of human cancer, which are prevalent in Korean patients, namely, breast cancer, colorectal cancer, gastric cancer, liver cancer, lung cancer, laryngeal cancer, prostate cancer, and uterine cervical cancer, for the methylation status of 13 genes (APC, COX-2, DAP-kinase, E-cadherin, GSTP1, hMLH1, MGMT, p14, p16, RASSF1A, RUNX3, THBS1, and TIMP-3). These genes were selected because they have important roles in tumor suppression, cell-to-cell attachment, cell cycle, DNA repair and protection, apoptosis, and metastasis, and also because they have been previously demonstrated to be inactivated by CpG island hypermethylation in cancer cell lines or primary tumor tissues. The aim of this study was to determine the methylation profiles of the major types of human cancers to better understand the role of CpG island hypermethylation in human carcinogenesis.
Archival tissue samples of primary tumors of 8 types were studied, i.e., of gastric cancer (adenocarcinoma, n=61), colon cancer (adenocarcinoma, n=48), lung cancer (adenocarcinoma, n=24), laryngeal cancer (squamous cell carcinoma, n=37), breast cancer (invasive ductal carcinoma, n=24), hepatocellular carcinoma (n=48), uterine cervical cancer (squamous cell carcinoma, n=46), and prostate carcinoma (adenocarcinoma, n=40). After microscopic examinations of hematoxylin and eosin-stained slides, tumoral portions with the ratio of tumor cells to normal cells more than 30:70 were marked with a pen, and the corresponding portions of the archival blocks were punched out. Punched tissues were cut with scissors into tiny pieces, dewaxed in xylene, and then treated in ethanol. DNA was extracted using proteinase K and phenol/chloroform/isoamylalcohol.
Bisulfite modification was performed as described previously (13). In brief, 5 µg of DNA was denatured by heating at 97℃ for 6 min and then cooled. After centrifugation, the DNA was added with 2 M NaOH and stored at room temperature for 15 min. The denatured DNA was then treated with 1 mM hydroquinone and 3.5 M sodium bisulfite and incubated for 16 hr at 55℃. Following purification using a purification kit (Genomed, Bad Oeynhausen, Germany), the DNA was treated with 3 M NaOH and precipitated with three volumes of 100% ethanol and a two-thirds volume of 7.5 M NH4Ac at -20℃. The precipitated DNA was then washed with 70% ethanol and dissolved in 10 mM Tris buffer. A panel of the 13 genes was analyzed for methylation status using MSP. The primer sequences of each gene, the annealing temperature and the number of cycles used, and references are given in Table 1. The 25 µL reaction volume contained a PCR mixture of; 1X PCR buffer (16.6 mM (NH4)2SO4/67 mM Tris/pH 8.8/6.7 mM MgCl2/10 mM β-mercaptoethanol), dNTPs (each at 1 mM), primers (10 pM each), and bisulfite-modified DNA (30-50 ng). The reactions were hot-started at 97℃ for 5 min prior to adding 0.75 units of Taq polymerase (Bioneer Co., Seoul, Korea). The PCR products (7 µL) were electrophoresed on 2.5% agarose gel and visualized after staining with ethidium bromide. Samples showing signals approximately equivalent to that of the size marker (3.5 ng) were scored as methylated. Samples that gave negative results in the PCR with specific primer sequences for the unmethylated forms of E-cadherin or p16 were excluded from the study. This was because the presence of unmethylated E-cadherin and p16 was considered to ensure adequate bisulfite modification and the integrity of the bisulfite-modified DNA in the samples. Normal peripheral blood lymphocytes, obtained from patients with no evidence of cancer (n=20), were used as a control group. The 13 genes were not methylated in the normal peripheral blood lymphocytes. For each MSP reaction, we used normal lymphocyte DNA treated with Sss1 methyl transferase and distilled water without template DNA as positive control and negative control, respectively.
All statistical calculations were made using the SPSS software (version 11.0 SPSS, Chicago, IL). Student's t-test was used to compare the numbers of methylated genes between different tissue types of cancers. The Mann-Whitney test was used to examine methylation concordances at multiple loci. The p-values of <0.05 were considered to be statistically significant.
We analyzed a total of 328 samples of human cancers encompassing eight different tissue types for 13 genes (APC, COX-2, DAP-kinase, E-cadherin, GSTP1, hMLH1, MGMT, p14, p16, RASSF1A, RUNX3, THBS1, and TIMP-3) using MSP. A representative example of MSP analysis is demonstrated in Fig. 1. The methylation frequencies of CpG islands for each gene in each tumor type are summarized in Table 2. The number of genes methylated per tumor type was 6.5, 4.4, 3.6, 3.4, 3.1, 3.1, 3.1, and 2.1 for stomach, liver, prostate, larynx, colorectal, lung, uterine cervix, and breast cancer, respectively. Gastric cancer showed the highest number of methylated genes, and the difference between the number of methylated genes in gastric cancer and the second most frequently methylated tumor, liver cancer, was statistically significant (p<0.001, two-tailed student t test).
As shown in Table 2, each tissue type and each gene showed its own specific methylation pattern. The number of genes that were methylated at a frequency of more than 40% in each tissue type ranged from nine (gastric cancer) to one (breast cancer), and genes frequently methylated in a specific tissue type differed from those frequently methylated in other tissue types. In gastric cancer, nine genes (APC, COX2, DAPK, E-cadherin, p14, p16, THBS1, and TIMP3) showed methylation frequencies of >44%, and the remainder (GSTP1, hMLH1, MGMT, and RASSF1A) were methylated in <24%. Prostate cancer demonstrated a contrasting methylation pattern; it showed methylation frequencies of <27% for the genes frequently methylated in gastric cancer (except for APC) and methylation frequency of >80% for GSTP1 and RASSF1A, which were less frequently methylated in gastric cancer.
The methylation frequencies of the 13 genes in all samples ranged from 53% to 6%, in the decreasing order of methylation frequency as follows; APC, DAPK, E-cadherin, RUNX3, RASSF1A, p14, p16, GSTP1, THBS1, COX2, TIMP3, MGMT, and hMLH1. APC, DAP-kinase, E-cadherin, p14, p16, and RASSF1A were methylated in all tissue types, but COX2, GSTP1, hMLH1, MGMT, RUNX3, THBS1, and TIMP3 were not. hMLH1 was methylated in gastric and colorectal cancers only, and GSTP1 was not methylated in laryngeal cancer or uterine cervical cancer (both of which are squamous cell carcinomas), but methylated in liver cancer and prostate cancer at frequencies of >40%. DAPK and RASSF1A showed contrasting methylation patterns; DAPK was methylated at frequencies of >40% in laryngeal, colorectal, gastric, and uterine cervical cancers but at frequencies of <40% in lung, breast, prostate, and liver cancers, whereas RASSF1A was methylated at frequencies of <40% in the former tissue types and at frequencies of >40% in the latter tissue types.
The number of genes methylated in each tissue type is summarized in Table 3. Concurrent methylation of more than five genes was observed in 601% of gastric cancers, 36% of hepatocellular carcinomas, 25% of prostate cancers, 17% of colorectal cancers, 17% of lung cancers, 8% of breast cancers, 8% of laryngeal cancers, and 4% of uterine cervical cancers. The distribution of the number of methylated genes was of the continuous pattern, not of bimodal pattern, in seven tissue types of human cancers except for gastric cancer.
To examine whether CpG island hypermethylation at a specific gene is coordinated with that of other genes, we determined and compared the number of other genes methylated when a specific gene was methylated or not. Methylation at each gene was associated with a higher number of methylated genes (Table 4), indicating the concordance of hypermethylation at multiple genes. The association was statistically significant, except for GSTP1 and RASSF1A.
CpG island hypermethylation is an important mechanism of inactivating tumor suppressor genes, and is found in virtually all tissue types of cancer. In the present study, we examined CpG island hypermethylation of 13 genes in eight human cancer tissue types, which included cancers prevalent in Korean patients, and were able to confirm that CpG island hypermethylation is a frequent event in all eight of these human cancers. However, the extent of aberrant CpG island hypermethylation differed within and between different cancer types. Our data shows that gastric cancer showed the highest number of methylated genes, followed by liver, prostate, larynx, colorectal, lung, uterine cervix, and breast cancer in descending order of the number of genes methylated per tumor type. In 7 cancers, except for gastric cancer, the distribution of the numbers of genes methylated formed a continuous pattern, from the least hypermethylated to the most hypermethylated cases, within the same tissue type, which contradicted the concept of CpG island methylator phenotype from which a bimodal pattern of distribution was expected (14). However, the concordant methylation of 12 gene CpG island loci, except for GSTP1, suggests the presence of a methylation defect, and supports the concept of the CpG island methylator phenotype. Whether a distribution is bimodal or continuous may depend on the selection of CpG island loci to define the CpG island methylator phenotype. Although reference CpG island loci have been proposed to define CpG island phenotype in colorectal cancer and gastric cancer (14, 15), no references have been set up in other tissue types. Studies to set up reference CpG island loci to define CpG island methylator phenotype for each tissue type are necessary.
In the present study, the average number of genes methylated was significantly higher in gastric cancer than in any other tissue types. This finding was consistent with the finding that the normal stomach shows a high frequency of age-related hypermethylation (16). We examined non-neoplastic tissues, including stomach, colon, liver, prostate, and uterine cervical tissues, for the methylation statuses of the same panel of genes (except APC) and found that the number of the genes methylated in the stomach exceeded those of the other normal tissue types (data not shown). The cause for this level of difference between tissue types is unclear, but it may be related to the accessibility of tissues to dietary factors or exogenous agents. N-methyl-N'-nitro-N-nitrosoguanidine, a well-known gastric carcinogen, has been demonstrated to induce CpG island hypermethylation of p16, and this hypermethylation increased with lesion progression along the multistep gastric carcinogenesis in the rat stomach (17). In addition, in vitro studies have demonstrated that the exposure to nickel causes CpG island hypermethylation (18, 19). Recent studies (20, 21) have shown that Helicobacter pylori infection is closely associated with aberrant CpG island hypermethylation in the normal stomach, which suggests that the high prevalence of Helicobacter pylori infection in the Korean population may contribute to the high frequency of aberrant methylation in the normal stomach.
A unique profile of CpG island hypermethylation was observed in each tumor type, in which CpG island hypermethylations of APC, DAP-kinase, E-cadherin, p14, p16, and RASSF1A were shared but those of COX-2, GSTP1, hMLH1, MGMT, THBS1, and TIMP-3 were tissue-type specific. These unique profiles can be utilized to characterize the primary sites of metastatic adenocarcinomas in the liver or lung. Methylation analysis of metastatic tumors may complement immunohistochemical studies in terms of elucidating primary sites. The reason why some genes were hypermethylated and others remained unmethylated in specific tissue types is unclear. One explanation is that certain genes become hypermethylated preferentially because their hypermethylation confers a selective growth/survival advantage to a given cancer cell. However, this hypothesis cannot explain why MyoD1, which is normally not expressed in epithelial cells, is methylated in colon cancer cells (22).
In addition, CpG island hypermethylation can be utilized as a molecular marker to predict the response to chemotherapy. MGMT removes methyl-residues from O6-methylguanine, which otherwise would lead to the misreading of DNA polymerase and transversion mutations. If MGMT is inactivated due to CpG island hypermethylation, cancer cells become sensitive to alkylating chemotherapeutic agent. Better prognoses have been reported in cases of hematologic or CNS malignancies with MGMT hypermethylation compared to those with no MGMT hypermethylation (23, 24). In the present study, laryngeal cancer showed a high frequency of MGMT methylation (more than 50%), which suggests that the prognostic value of MGMT hypermethylation should be assessed in this tissue type.
Alterations of the transforming growth factor-β signaling pathway are common in human cancers. RUNX3 is a component of this pathway, and its inactivation by promoter CpG island hypermethylation has been described in various human cancers, including stomach (25), colon (26), liver (27, 28), lung (29), bile duct (30), and pancreatic cancer (31). In the present study, RUNX3 was methylated at frequencies >45% in lung, larynx, liver, and stomach cancers, but at frequencies of <30% in prostate, breast, and colon cancers. The relationship between RUNX3 methylation and clinical stage differs depending on tissue type, and a close relationship between RUNX3 methylation and an advanced clinical stage has been demonstrated in stomach (25, 31), lung (29), prostate (32), and urinary bladder cancers (33). However, no such association was found in liver cancer (28). In the present study, we found a significant difference of clinical stage between prostate cancers with and without RUNX3 methylation, but no such difference in other cancer types (data not shown). It might be possible that the lack of a close association in stomach and lung cancers was due to the limited number of the cases examined.
In conclusion, we analyzed the methylation status of 13 genes in 8 tissue types of human cancers and found that CpG island hypermethylation is a frequent event in the cancers examined and that each CpG island locus and each tissue type has its own gene-specific and tumor type-specific methylation pattern. CpG island hypermethylation was found most frequently in stomach and liver cancers and least frequently found in uterine cervical and breast cancers.
Figures and Tables
Fig. 1
Representative samples of MSP analysis for the methylated (M) and unmethylated (U) forms of six CpG island loci (APC, GSTP1, hMLH1, p16, RASSF1A, and RUNX3) in stomach cancer, colon cancer, and prostate cancer. The positive control was a placental DNA treated with Sss1 methylase before bisulfite modification, and the negative control was distilled water without template DNA. Marker 1 and 2, 100 bp DNA ladder of 14 ng and 3.5 ng, respectively; +, positive control; -, negative control.
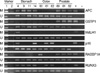
Table 1
Oligonucleotide sequences of primers (methylated or unmethylated forms) used for methylation specific PCR, their PCR product sizes and annealing temperatures
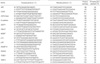
References
1. Larsen F, Gundersen G, Lopez R, Prydz H. CpG islands as gene markers in the human genome. Genomics. 1992. 13:1095–1107.


2. Antequera F, Bird A. Number of CpG islands and genes in human and mouse. Proc Natl Acad Sci USA. 1993. 90:11995–11999.


3. Ponger L, Duret L, Mouchiroud D. Determinants of CpG islands: expression in early embryo and isochore structure. Genome Res. 2001. 11:1854–1860.


4. Nan X, Ng HH, Johnson CA, Laherty CD, Turner BM, Eisenman RN, Bird A. Transcriptio-nal repression by the methyl-CpG-binding protein MeCP2 involves a histone deacetylase complex. Nature. 1998. 393:386–389.


5. Jones PL, Veenstra GJ, Wade PA, Vermaak D, Kass SU, Landsberger N, Strouboulis J, Wolffe AP. Methylated DNA and MeCP2 recruit histone deacetylase to repress transcription. Nat Genet. 1998. 19:187–191.


6. Bird AP, Wolffe AP. Methylation-induced repression-belts, braces, and chromatin. Cell. 1999. 99:451–454.
7. Jones PA, Takai D. The role of DNA methylation in mammalian epigenetics. Science. 2001. 293:1068–1070.


8. Baylin SB, Herman JG, Graff JR, Vertino PM, Issa JP. Alterations in DNA methylation: A functional aspect of neoplasia. Adv Cancer Res. 1998. 72:141–196.
9. Jones PA, Laird PW. Cancer epigenetic comes of age. Nat Genet. 1999. 21:163–167.
10. Costello JF, Fruhwald MC, Smiraglia DJ, Rush LJ, Robertson GP, Gao X, Wright FA, Feramisco JD, Peltomaki P, Lang JC, Schuller DE, Yu L, Bloomfield CD, Caligiuri MA, Yates A, Nishikawa R, Su Huang H, Petrelli NJ, Zhang X, O'Dorisio MS, Held WA, Cavenee WK, Plass C. Aberrant CpG-island methylation has non-random and tumour-type-specific patterns. Nat Genet. 2000. 24:132–138.


11. Esteller M, Corn PG, Baylin SB, Herman JG. A gene hypermethylation profile of human cancer. Cancer Res. 2001. 61:3225–3229.
13. Herman JG, Graff JR, Myohanen S, Nelkin BD, Baylin SB. Methylation-specific PCR: a novel PCR assay for methylation status of CpG islands. Proc Natl Acad Sci USA. 1996. 93:9821–9826.


14. Toyota M, Ahuja N, Ohe-Toyota M, Herman JG, Baylin SB, Issa JP. CpG island methylator phenotype in colorectal cancer. Proc Natl Acad Sci USA. 1999. 96:8681–8686.


15. Toyota M, Ahuja N, Suzuki H, Itoh F, Ohe-Toyota M, Imai K, Baylin SB, Issa JP. Aberrant methylation in gastric cancer associated with the CpG island methylator phenotype. Cancer Res. 1999. 59:5438–5442.
16. Kang GH, Lee HJ, Hwang KS, Lee S, Kim JH, Kim JS. Aberrant CpG island hypermethylation of chronic gastritis, in relation to aging, gender, intestinal metaplasia, and chronic inflammation. Am J Pathol. 2003. 163:1551–1556.


17. Bai H, Gu L, Zhou J, Deng D. p16 hypermethylation during gastric carcinogenesis of Wistar rats by N-methyl-N'-nitro-N-nitrosoguanidine. Mutat Res. 2003. 535:73–78.


18. Lee YW, Klein CB, Kargacin B, Salnikow K, Kitahara J, Dowjat K, Zhitkovich A, Christie NT, Costa M. Carcinogenic nickel silences gene expression by chromatin condensation and DNA methylation: a new model for epigenetic carcinogens. Mol Cell Biol. 1995. 15:2547–2557.


19. Sutherland JE, Peng W, Zhang QW, Costa M. The histone deacetylases inhibitor A reduces nickel-induced gene silencing in yeast and mammalian cells. Mutat Res. 2001. 479:225–233.
20. Maekita T, Nakazawa K, Mihara M, Nakajima T, Yanaoka K, Iguchi M, Arii K, Kaneda A, Tsukamoto T, Tatematsu M, Tamura G, Saito D, Sugimura T, Ichinose M, Ushijima T. High levels of aberrant DNA methylation in Helicobacter pylori-infected gastric mucosae and its possible association with gastric cancer risk. Clin Cancer Res. 2006. 12:989–995.


21. Maekita T, Nakazawa K, Mihara M, Nakajima T, Yanaoka K, Iguchi M, Arii K, Kaneda A, Tsukamoto T, Tatematsu M, Tamura G, Saito D, Sugimura T, Ichinose M, Ushijima T. High levels of aberrant DNA methylation in Helicobacter pylori-infected gastric mucosae and its possible association with gastric cancer risk. Clin Cancer Res. 2006. 12:989–995.


22. Ahuja N, Li Q, Mohan AL, Baylin SB, Issa JP. Aging and DNA methylation in colorectal mucosa and cancer. Cancer Res. 1998. 58:5489–5494.
23. Esteller M, Garcia-Foncillas J, Andion E, Goodman SN, Hidalgo OF, Vanaclocha V, Baylin SB, Herman JG. Inactivation of the DNA-repair gene MGMT and the clinical response of gliomas to alkylating agents. N Engl J Med. 2000. 343:1350–1354.
24. Esteller M, Gaidano G, Goodman SN, Zagonel V, Capello D, Botto B, Rossi D, Gloghini A, Vitolo U, Carbone A, Baylin SB, Herman JG. Hypermethylation of the DNA repair gene O(6)-methylguanine DNA methyltransferase and survival of patients with diffuse large B-cell lymphoma. J Natl Cancer Inst. 2002. 94:26–32.


25. Li QL, Ito K, Sakakura C, Fukamachi H, Inoue K, Chi XZ, Lee KY, Nomura S, Lee CW, Han SB, Kim HM, Kim WJ, Yamamoto H, Yamashita N, Yano T, Ikeda T, Itohara S, Inazawa J, Abe T, Hagiwara A, Yamagishi H, Ooe A, Kaneda A, Sugimura T, Ushijima T, Bae SC, Ito Y. Causal relationship between the loss of RUNX3 expression and gastric cancer. Cell. 2002. 109:113–124.


26. Ku JL, Kang SB, Shin YK, Kang HC, Hong SH, Kim IJ, Shin JH, Han IO, Park JG. Promoter hypermethylation downregulates RUNX3 gene expression in colorectal cancer cell lines. Oncogene. 2004. 23:6736–6742.


27. Mori T, Nomoto S, Koshikawa K, Fujii T, Sakai M, Nishikawa Y, Inoue S, Takeda S, Kaneko T, Nakao A. Decreased expression and frequent allelic inactivation of the RUNX3 gene at 1p36 in human hepatocellular carcinoma. Liver Int. 2005. 25:380–388.


28. Park WS, Cho YG, Kim CJ, Song JH, Lee YS, Kim SY, Nam SW, Lee SH, Yoo NJ, Lee JY. Hypermethylation of the RUNX3 gene in hepatocellular carcinoma. Exp Mol Med. 2005. 37:276–281.


29. Li QL, Kim HR, Kim WJ, Choi JK, Lee YH, Kim HM, Li LS, Kim H, Chang J, Ito Y, Youl Lee K, Bae SC. Transcriptional silencing of the RUNX3 gene by CpG hypermethylation is associated with lung cancer. Biochem Biophys Res Commun. 2004. 314:223–228.


30. Wada M, Yazumi S, Takaishi S, Hasegawa K, Sawada M, Tanaka H, Ida H, Sakakura C, Ito K, Ito Y, Chiba T. Frequent loss of RUNX3 gene expression in human bile duct and pancreatic cancer cell lines. Oncogene. 2004. 23:2401–2407.


31. Sakakura C, Hasegawa K, Miyagawa K, Nakashima S, Yoshikawa T, Kin S, Nakase Y, Yazumi S, Yamagishi H, Okanoue T, Chiba T, Hagiwara A. Possible involvement of RUNX3 silencing in the peritoneal metastases of gastric cancers. Clin Cancer Res. 2005. 11:6479–6488.

