Abstract
Transplantation of marrow-derived mesenchymal stem cells (MSCs), expanded by culture in addition to whole bone marrow, has been shown to enhance engraftment of human hematopoietic stem cells (HSCs). Our hypothesis was that there might be an optimum ratio range that could enhance engraftment. We examined the percent donor chimerism according to the ratio of HSCs to MSCs in non-obese diabetic/severe combined immunodeficiency (NOD/SCID) mice. We tested a series of ratios of co-transplanted CD34+-selected bone marrow cells, and marrow-derived MSCs into sublethally irradiated NOD/SCID mice. In all experiments, 1×105 bone marrow derived human CD34+ cells were administered to each mouse and human MSCs from different donors were infused concomitantly. We repeated the procedure three times and evaluated engraftment with flow cytometry four weeks after each transplantation. Serial ratios of HSCs to MSCs were 1:0, 1:1, 1:2 and 1:4, in the first experiment, 1:0, 1:1, 1:2, 1:4 and 1:8 in the second and 1:0, 1:1, 1:4, 1:8 and 1:16 in the third. Cotransplantation of HSCs and MSCs enhanced engraftment as the dose of MSCs increased. Our results suggest that the optimal ratio of HSCs and MSCs for cotransplantation might be in the range of 1:8-1:16; whereas, an excessive dose of MSCs might decrease engraftment efficiency.
Mesenchymal stem cells (MSCs) are cells that have been demonstrated to have the ability to differentiate into distinct mesenchymal tissues such as bones, tendons, muscles, adipose tissues, cartilage and nerve tissues as well as to support hematopoiesis (1-5). MSCs have been labeled in a variety of ways since Dexter named them 'stromal cells' in the 1980's (6). Caplan designated the term mesenchymal stem cells in the 1990's (7), while others still refer to them as marrow stromal cells. The methods used for preparation of these cells have not yet been standardized; the differential potential of MSCs has not yet been fully determined due to the limitations of in vitro expansion. Since Noort et al. reported that MSCs promote engraftment of umbilical cord blood-derived CD34+ cells in non-obese diabetic/severe combined immunodeficiency (NOD/SCID) mice (8, 9) many clinical trials have been pursued to better understand their role in bone marrow transplantation (10). Unlike transplantation of hematopoietic stem cells (HSCs) only, the questions regarding in vitro expansion remain unanswered; in particular, questions regarding the optimal infusion buffer and whether to use thawed cells or fresh detached cells for cotransplantation of MSCs and HSCs remain unanswered. We studied the optimal ratio of HSCs to MSCs to facilitate engraftment, and tried to determine the appropriate ratio for enhancement of HSCs.
Human CD34+ cells were collected by bone marrow aspiration from 34 to 57 yr-old healthy volunteers (Table 1) under an Institutional Review Board approval protocol of Samsung Medical Center, Korea. CD34+ cells were isolated using the MACS sort system (Miltenyi Biotec GmbH, Germany). The purity of the CD34+-selected cells was more than 90%. MSCs were cultured as previously described (11). We used bone marrow derived MSCs that were not exceeded over 3 passages. MSCs were confirmed to be negative for hematopoietic markers by flow cytometry. For further clarification of MSCs, the following antibodies were used: CD14 (BD-Pharmingen, Palo Alto, CA, U.S.A.), CD29, CD34, CD44, CD45, CD51-61, CD90, CD105, CD106, CD166, HLA-DR, Stro-1, SH2-M2, SH3-M2 and SH4-M2.
NOD/SCID mice, purchased from Jackson Laboratories (MA, U.S.A.) were maintained in microisolator cages in specific pathogen free conditions. Eight-week old mice received sublethal total-body irradiation with 300 cGy from 137-Cs source within 24 hr before transplantation. In all experiments, 1×105 bone marrow derived human CD34+ cells were administered to each mouse and MSCs were used concomitantly. Bone marrow derived CD34+ cells and MSCs were injected in a final volume of 500 to 1,000 µL HBSS (Bio-Whittaker, Baltimore, MD, U.S.A.) per mouse and were infused via tail vein injection. Three experiments were performed repeatedly. Serial ratios of bone marrow derived CD34+ cells to MSCs were 1:0, 1:1, 1:2 and 1:4, in the first experiment (4 mice), 1:0, 1:1, 1:2, 1:4 and 1:8 in the second (10 mice) and 1:0, 1:1, 1:4, 1:8 and 1:16 in the third (15 mice).
Mice were sacrificed 4 weeks posttransplant and cells from the bone marrow were analyzed by flow cytometry. Cells were then incubated with PE-mouse anti-human CD45 (BD-Pharmingen, Palo Alto, CA, U.S.A.) for 30 min at 4℃, washed, resuspended in 1% paraformaldehyde in phosphate-buffered saline, and analyzed on a flow cytometry (FACS Vantage, Becton Dickinson, Palo Alto, CA, U.S.A.).
We isolated CD34+ HSCs from bone marrow using CD34+ cell directed kit (Miltenyi Biotec GmbH, Germany), and at the same time, isolated MSCs from CD34- population. The CD34+ cells infused had purities over 90% and CD34+/CD38- cells were in the range of 30±5-40±5% (data not shown) (Fig. 1).
FACS results showed that CD29, CD44, CD90, CD105, CD166, SH2, SH3, and SH4 were expressed in more than 90% of MSCs. Typical hematopoietic antigens (CD14, CD34 and CD45) as well as CD51-61, CD106 and HLA-DR known to be negative markers for MSCs (2, 12, 13) were hardly expressed. On the other hand, Stro-1, known to be related with homing to bone marrow (14), was expressed in 3.14% of MSCs (Fig. 2).
We observed that the engraftment efficiency after cotransplantation was improved as the MSCs dose was increased up to a certain level in all three independent experiments. The engraftment fold-increase of the 1:4 group was 3.99, 19.78 and 6.03 compared to the HSCs only group in experiment 1, 2 and 3, respectively (Table 2). The percent donor chimerism of the 1:16 group was inferior to that of the 1:8 ratio group (Fig. 3). The 1:4 and 1:8 ratio groups were associated with a higher percent donor chimerism than any other group studied (Fig. 4).
MSCs have recently been used experimentally in clinical trials of cardiovascular repair (15, 16), treatment of lung fibrosis (17), spinal cord injury (18) and bone and cartilage repair (4, 19) as a stem cell source. MSCs have been successfully used for therapeutic application when they are derived from bone marrow (20).
Generally, an accepted range of HSC dose for engraftment after bone marrow transplantation is 3-4×106 CD34+ cells/kg of recipient body weight for humans. Considering that a mouse weighs about 30 g on average, we postulated that 9-12×104 CD34+ cells should be a reasonable dose range for a mouse, and therefore infused 1×105 CD34+ cells.
Usually, the fluorescence intensity of CD34+ cells when conjugated with FITC is weaker than that when conjugated with PE using flow cytometry. Therefore, it is a matter of course that the percentage of CD34+ fraction may is different to some extent according to the conjugated materials even though the testing samples are exactly the same. Authors usually evaluate the purity of overall CD34+ cell fraction stained with PE according to the manufacturer's protocol of CD34 sort kit (Miltenyi Biotec, Germany). Fig. 1B is intended to show the percentage of CD34+/CD38- fraction which is known to be more primitive and have higher engraftment potential than CD34+/CD38+ fraction using PE-conjugated CD38 and FITC-conjugated CD34.
At present we do not know how long MSCs will maintain innate characteristics including the ability to differentiate and cytokine production; nor do we know what the best composition culture media should contain for maintaining MSCs. We used early MSCs not exceeding 3 passages based on the assumption that early passage cells would be more likely to have the innate characteristics of MSCs.
Cotransplantation of HSCs and MSCs enhanced engraftment as the dose of the MSCs were increased up until a 1:8 ratio. The percent donor chimerism in the 1:16 group, however, was lower than that of the 1:8 group even though it was still higher than the HSCs only group as shown in the experiment 3. These findings suggest that HSCs and MSCs have a threshold ratio of HSCs to MSCs for enhancing engraftment when cotransplanted into a NOD/SCID mouse. Our findings suggest that the optimal ratio of HSCs to MSCs in cotransplantation might be between 1:8 and 1:16 and we are conducting further experiments using these ratios. We confirmed MSCs engraftment enhancing ability in 3 experiments. Each experiment was performed with bone marrow-derived MSCs obtained from a different volunteer donor, and showed different enhancing abilities. For example, the mean fold-increase of the 1:4 ratio group was 3.99 compared with the HSCs only group in the experiment 1, while it was 7.21 in the experiment 2, and 1.21 in the experiment 3. The CD34+ cells were also derived from different donors; however, it is unlikely that the quality of MSCs is the sole determinant of engraftment efficiency. It is clear that the CD34+ cell dose has been shown to be strongly associated with engraftment kinetics regardless of individual differences (21, 22).
In addition, the proliferation rate and morphology of the 3 MSCs experiments differed when observed during cell expansion before cotransplantation (data not shown). Therefore, our findings suggest that the engraftment enhancing ability of MSCs might have considerable difference according to the MSC donor.
Our study demonstrated that MSCs could enhance engraftment in a dose-dependent manner up to a certain level in NOD/SCID mice. Future studies should help elucidate further mechanisms associated with engraftment such as secretion of specific MSC-cytokines that help enhance engraftment. For clinical application to human patients characteristics of MSCs including immunosuppression and HLA restriction require further explanation.
Figures and Tables
Fig. 1
Isolation of HSCs from bone marrow and results of FACS analyses. HSCs were isolated by MACS sort system from bone marrow. The purity of HSCs infused to NOD/SCID mice was more than 90%. (A) Isotypes were stained with FITC-anti-mouse IgG1 and PE-anti-mouse IgG1. (B) Dot plots show FITC-anti-human CD34 on x-axis and PE-anti-human CD38 on y-axis. (C) Isolated CD34+ cells were stained PE-anti-human CD34 only on y-axis.
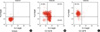
Fig. 2
Results of MSCs phenotypes by FACS analysis. MSCs that were used in this test were positive for CD29 (BD-Pharmingen, Palo Alto, CA, U.S.A.), CD44, CD90, CD105, CD166, SH2, SH3, and SH4, while negative for CD14, CD34, CD45, CD51-61, CD106 and HLA-DR. Stro-1 was weakly expressed. Blank peaks indicate the isotype of each cell and closed peaks represent expression of each marker.
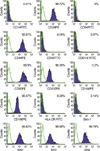
Fig. 3
MSCs enhanced human cell engraftment in bone marrow of NOD/SCID mice in a dose-dependent manner at 4 weeks posttransplant. (A) CD34+ only group (1.0×105 cells). (B) The ratio of CD34+ to MSCs was 1:1 (1.0×105 cells each). (C) The ratio of CD34+ to MSCs was 1:2 (1.0×105:2.0×105 cells). (D) The ratio of CD34+ to MSC was 1:4 (1.0×105:4.0×105 cells). (E) The ratio of CD34+ to MSC was 1:8 (1.0×105:8.0×105 cells). This is representative of 2 analyses in the experiment 2.
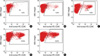
Fig. 4
Results of contransplantation. In all experiments, the engraftment in bone marrow was increased as the MSCs dose was increased. In experiment 3, the engraftment in the group of a 1:16 ratio is inferior to that of a 1:8 ratio. *Indicates significant statistical difference between the CD34+ only group and a cotransplanted group.
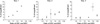
References
1. Majumdar MK, Thiede MA, Mosca JD, Moorman M, Gerson SL. Phenotypic and functional comparison of cultures of marrow-derived mesenchymal stem cells (MSCs) and stromal cells. J Cell Physiol. 1998. 176:57–66.


2. Pittenger MF, Mackay AM, Beck SC, Jaiswal RK, Douglas R, Mosca JD, Moorman MA, Simonetti DW, Craig S, Marshak DR. Multilineage potential of adult human mesenchymal stem cells. Science. 1999. 284:143–147.


3. Johnstone B, Hering TM, Caplan AI, Goldberg VM, Yoo JU. In vitro chondrogenesis of bone marrow-derived mesenchymal progenitor cells. Exp Cell Res. 1998. 10:265–272.
4. Bruder SP, Fink DJ, Caplan AI. Mesenchymal stem cells in bone development, bone repair, and skeletal regeneration therapy. J Cell Biochem. 1994. 56:283–294.
5. Gimble J, Guilak F. Adipose-derived adult stem cells: isolation, characterization, and differentiation potential. Cytotherapy. 2003. 5:362–369.


7. Caplan AI. Osteogenesis imperfecta, rehabilitation medicine, fundamental research and mesenchymal stem cells. Connect Tissue Res. 1995. 31:S9–S14.


8. Noort WA, Kruisselbrink AB, in't Anker PS, Kruger M, van Bezooijen RL, de Paus RA, Heemskerk MH, Lowik CW, Falkenburg JH, Willemze R, Fibbe WE. Mesenchymal stem cells promote engraftment of human umbilical cord blood-derived CD34(+) cells in NOD/SCID mice. Exp Hematol. 2002. 30:870–878.


9. Erices AA, Allers CI, Conget PA, Rojas CV, Minguell JJ. Human cord blood-derived mesenchymal stem cells home and survive in the marrow of immunodeficient mice after systemic infusion. Cell Transplant. 2003. 12:555–561.


10. Devine SM, Hoffman R. Role of mesenchymal stem cells in hematopoietic stem cell transplantation. Curr Opin Hematol. 2000. 7:358–363.


11. Kim DH, Yoo KH, Choi KS, Choi J, Choi SY, Yang SE, Yang YS, Im HJ, Kim KH, Jung HL, Sung KW, Koo HH. Gene expression profile of cytokine and growth factor during differentiation of bone marrow-derived mesenchymal stem cell. Cytokine. 2005. 31:119–126.


12. Haynesworth SE, Baber MA, Caplan AI. Cell surface antigens on human marrow-derived mesenchymal cells are detected by monoclonal antibodies. Bone. 1992. 13:69–80.


13. Prockop DJ. Marrow stromal cells as stem cells for nonhematopoietic tissues. Science. 1997. 276:71–74.


14. Bensidhoum M, Chapel A, Francois S, Demarquay C, Mazurier C, Fouillard L, Bouchet S, Bertho JM, Gourmelon P, Aigueperse J, Charbord P, Gorin NC, Thierry D, Lopez M. Homing of in vitro expanded Stro-1- or Stro-1+ human mesenchymal stem cells into the NOD/SCID mouse and their role in supporting human CD34 cell engraftment. Blood. 2004. 103:3313–3319.
15. Rafii S, Meeus S, Dias S, Hattori K, Heissig B, Shmelkov S, Rafii D, Lyden D. Contribution of marrow-derived progenitors to vascular and cardiac regeneration. Semin Cell Dev Biol. 2002. 13:61–67.


16. Shake JG, Gruder PJ, Baugartner WA, Senechal G, Meyers J, Redmond JM, Pittenger MF, Martin BJ. Mesenchymal stem cell implantation in a swine myocardial infarct model: engraftment and functional effects. Ann Thorac Surg. 2002. 73:1919–1925.


17. Ortiz LA, Gambelli F, McBride C, Gaupp D, Baddoo M, Kaminski N, Phinney DG. Mesenchymal stem cell engraftment in lung is enhanced in response to bleomycin exposure and ameliorates its fibrotic effects. Proc Natl Acad Sci USA. 2003. 100:8407–8411.


18. Hofstter CP, Schwarz EJ, Hess D, Widenfalk J, El Manira A, Prockop DJ, Olson L. Marrow stromal cells form guiding stands in the injured spinal cord and promote recovery. Proc Natl Acad Sci USA. 2001. 99:2199–2204.
19. De Kok IJ, Peter SJ, Archambault M, van den Bos C, Kadiyala S, Aukhil I, Cooper LF. Investigation of allogeneic mesenchymal stem cell-based alveolar bone formation: preliminary findings. Clin Oral Implants Res. 2003. 14:481–489.
20. Orlic D. Adult bone marrow stem cells regenerate myocardium in ischemic heart disease. Ann NY Acad Sci. 2003. 996:152–157.


21. Ketterer N, Salles G, Raba M, Espinouse D, Sonet A, Tremisi P, Dumontet C, Moullet I, Eljaafari-Corbin A, Neidhardt-Berard EM, Bouafia F, Coiffier B. High CD34+ cell counts decrease hematologic toxicity of autologous peripheral blood progenitor cell transplantation. Blood. 1998. 91:3148–3155.


22. Weaver CH, Hazelton B, Birch R, Palmer P, Allen C, West W. An analysis of engraftment kinetics as a function of CD34 content of peripheral blood progenitor cell collection in 692 patients after the administration of myeloblative chemotherapy. Blood. 1995. 86:3961–3969.