Abstract
The purpose of this study was to demonstrate the cellular localization of cyclooxygenase-2 (COX-2) and caveolin-3 (Cav-3) in primarily cultured rat chondrocytes. In normal rat chondrocytes, we observed relatively high levels of Cav-3 and a very low level of COX-2 mRNA and protein. Upon treating the chondrocytes with 5 µM of CdCl2 (Cd) for 6 hr, the expressions of COX-2 mRNA and protein were increased with the decreased Cav-3 mRNA and protein expressions. The detergent insoluble caveolae-rich membranous fractions that were isolated from the rat chondrocytes and treated with Cd contained the both proteins of both COX-2 and Cav-3 in a same fraction. The immuno-precipitation experiments showed complex formation between the COX-2 and Cav-3 in the rat chondrocytes. Purified COX-2 with glutathione S-transferase-fused COX-2 also showed complex formation with Cav-3. Confocal and electron microscopy also demonstrated the co-localization of COX-2 and Cav-3 in the plasma membrane. The results from our current study show that COX-2 and Cav-3 are co-localized in the caveolae of the plasma membrane, and they form a protein-protein complex. The co-localization of COX-2 with Cav-3 in the caveolae suggests that the caveolins might play an important role for regulating the function of COX-2.
Cyclooxygenase (also be known as prostaglandin endoperoxidase or prostaglandin G/H synthase) is the rate-limiting enzyme for the production of prostaglandins (PGs) and thromboxanes from free arachidonic acid (1), which is liberated from the membrane-bound phospholipids when the cell is injured or there is receptor activation. Two isoforms of cyclooxygenase (COX) have been identified, COX-1 and COX-2 (2-4). COX-1 is believed to be responsible for the synthesis of prostaglandins during homeostasis and it is constitutively expressed at low levels (5). In contrast, the COX-2 gene is an immediate-early response gene that is inducible by many stimuli (1, 6).
Caveolins (Cav) are integral membrane proteins present in the caveola, the small flask-shaped and detergent insoluble invaginations in the plasma membrane, and they have been implicated to function in the vesicular transport processes and for the transduction of receptor generated signals (7). Recent studies have shown that many signal proteins, enzymes and receptors are localized in the caveolae by being anchored there through the caveolins (8). In 2001, Liou and coworkers (9) have reported that COX-2 was co-localized with Cav-1 in human fibroblasts. In addition, we have reported COX-1 was also co-localized with Cav-1 and Cav-2 in human embryonic kidney (HEK 293) cells (10). Many investigators have reported that Cav-1 and Cav-2 bind to some proteins and regulate their functions. With respect to Cav-3, there is a little information on the protein-protein interaction of Cav-3. Cav-3 was shown to be specifically expressed in muscle and it was also detected in brain astroglial cells (11). In 1999, Schwab and co-workers (12) reported that rat chondrocytes cultured from the femoral and tibial articular surfaces of knee joints of newborn rat also expressed Cav-3. COX-2 has been related with inflammation that was induced by many causes. COX-2 inhibitors have used to decrease the pain of arthritis patients. In this connection, we hypothesize that Cav-3 may also be related with the function of COX-2.
The purpose of this study, was to investigate the cellular localization of the inducible form of COX-2 and Cav-3 in cultured rat chondrocytes as a first step to demonstrating the relationship between COX-2 and Cav-3.
Cartilage of normal appearance was aseptically prepared from the femoral and tibial articular surfaces of knee joints of newborn rats. Catilage pieces were digested enzymatically according to the slightly modified method of Kiepe (13). Briefly, hyaluronidase (0.5%), trypsin (0.25%) and collagenase (0.2%) were treated for 2 hr. After washing and centrifugation, cells were resuspended in Ham's F12/Dulbecco's Modified Eagle's Medium with 10% fetal bovine serum, 50 µg/mL gentamicin. The cells were grown at 37℃ with 5% CO2 in air. In order to identify chondrocytes, the expression of type II collagen and aggrecan mRNAs and proteins as a chondrogenic markers were determined by RT-PCR and Western blot analysis. For cell culture, used chemicals and reagents were purchased from Gibco BRL (Grand Island, NY, U.S.A.) and Sigma-Aldrich (St. Louis, MO, U.S.A.). Used primer sequences (TaKaRa Korea Biomedical Co., Seoul, Korea) are shown in Table 1.
For purification of the GST-COX-2 and -Cav-3 fusion protein, the coding region of COX-2 and Cav-1 cDNA were amplified by polymerase chain reaction and cloned into SalI and NotI sites for COX-2 and BamHI and EcoRI sites for Cav-1 of the GST expression vector pGEX4T-3 and -1, respectively. The GST fusion proteins were expressed in transformed Escherichia coli induced by isopropyl-1-thio-β-D-galactopyranoside and purified by affinity chromatography using GST purification modules (Amersham Pharmacia Biotech, Birkinghamshire, U.K.). Immunoprecipitation experiments using respective antibodies were performed using modified method of the previous report (9).
Total RNA was extracted with TRI Reagent (Sigma-Aldrich) according to the manufacturer's instructions and 500 ng total RNA was reverse transcribed with 5 U of AMV reverse transcriptase XL for 30 min. The cDNAs were used as templates for PCR and the conditions for the PCR were as follows; one cycle of 1 min at 94℃, 35 cycles each for 30 sec at 94℃, 30 sec at 55℃ and 90 sec at 72℃, and a final cycle of 10 min at 72℃. The products (15 µL) were separated by a 1% agarose gel electrophoresis and stained with ethidium bromide. Used reagents for RT-PCR were bought from TaKaRa.
The primarily cultured rat chondrocytes were washed with cold phosphate-buffered saline (PBS, pH 7.4), harvested and homogenized in 9 volumes of 0.3 M sucrose, 0.26 unit/mL aprotinin, 0.1 mM phenylmethylsulfonyl fluoride, 10 µg/mL leupeptin and 10 µg/mL trypsin inhibitor, with 10 strokes of a motor-driven Teflon/glass homogenizer. The homogenate was centrifuged for 10 min at 8,000 rpm, and the supernatant centrifuged for 20 min at 8,000 rpm. The supernatant was centrifuged for 40 min at 45,000 rpm, and the membrane pellet was re-suspended in 0.25 M sucrose, 100 mM KCl, 5 mM MgCl2, and 50 mM Tris (pH 7.4). Aliquots were heated at 100℃ for 10 min in sample buffer and subjected to 10% SDS-polyacrylamide gel electrophoresis. The separated proteins were transferred electrophoretically to a Hybond-P polyvinylidene difluoride transfer membrane (Amersham Pharmacia Biotech). This was blocked with 5% non-fat dried milk and incubated with polyclonal goat anti-human COX-2 antibody (1:500, Santa Cruz Biotechn, Santa Cruz, CA, U.S.A.) or monoclonal mouse anti-rat Cav-3 antibody (1:200, Transduction Lab., Lexington, MS, U.S.A.), polyclonal rabbit anti-mouse aggrecan (1:1,000, CHEMICON, Temecula, CA, U.S.A.) or monoclonal mouse anti-human collagen type 2 (1:1,000, CHEMICON) for 1 hr at room temperature followed by horseradish peroxidase-conjugated secondary antibodies (1: 3,000, Jackson Immunoresearch, West Grove, PA, U.S.A.) for 1 hr at room temperature. After washing, the membrane was visualized by the ECL kit (Amersham Pharmacia Biotech).
Caveolae-rich membrane fractions from the Cd (5 µM for 6 hr)-treated rat chondrocytes were fractionated using modified sucrose gradient ultracentrifugation (9, 14). In brief, chondrocytes were homogenized in 2 mL of lysis buffer (10 mM Tris-HCl, pH 7.5, 150 mM NaCl, 5 mM EDTA, 1 mM phenylmethylsulfonyl fluoride, 10 µg/mL leupeptin, 10 µg/mL trypsin inhibitor, and 60 mM OG, octyl β-D-glucopyranoside, Sigma-Aldrich) followed by sonication. The homogenate was brought to 40% sucrose by addition of an equal volume of 80% sucrose and loaded in an ultracentrifuge tube. A discontinuous sucrose gradient was layered on top of the sample by placing 4 mL of 30% and 4 mL of 5% sucrose, respectively. After centrifugation at 200,000×g for 16-20 hr at 4℃, the twelve 1-ml fractions from the top to the bottom were collected. Each fraction was analyzed by Western blot for its COX-2 and Cav-3 using specific antibodies, respectively.
The lysates of Cd-treated rat chondrocytes were incubated with antibodies specific for Cav-3, COX-2 or a pre-immune control IgG at a final concentration of 4 µg/mL for 4 hr at 4℃. Protein-A-Sepharose was then added for 2 hr at 4℃. Thereafter, the samples were centrifuged and the pellets were washed 4 times with PIPA buffer (150 mM NaCl, 1 mM EDTA, 60 mM OG, 1% NP-40, 0.05% SDS, and 10 mM Tris; pH 8.0) and then prepared for the Western blotting analysis by boiling in the sample buffer to determine whether interactive binding has occurred between COX-2 and Cav-3.
The cultured rat chondrocytes on cover slips were fixed with 95% ice-cold ethanol for 10 min at room temperature and permeabilized by 0.1% Triton X-100 solution in PBS (10 min, room temperature). The nonspecific binding sites were blocked with 10% goat serum in PBS for 30 min in room temperature. Polyclonal COX-2 antibody (1:200) and monoclonal Cav-3 antibody (1:50) were treated to the fixed cells for 1 hr in room temperature. Secondary antibodies without prior antibody treatment were also included as controls. After washing, cells were double stained with FITC- and Texas Red-conjugated secondary antibodies for 30 min. After washing, samples were examined at the appropriate wavelengths under a Bio-Rad MRC 1000 confocal microscope (Hercules, CA, U.S.A.). More than 50 cells have been inspected per experiment and the photos of cells with typical morphology and staining are presented.
For the immunogold EM study of Cd-treated rat chondrocytes, cells that had been grown on a culture dish were rinsed with PBS followed by fixation in PBS containing 0.1% glutaraldehyde, 4% paraformaldehyde and 3.5% sucrose for 1 hr at 4℃. The cells were then scraped from the culture dish and centrifuged to obtain the cell pellet that was later embedded in 1% agar in PBS. The cell blocks were then washed three times in PBS followed by postfixation with 1% osmium tetroxide on ice for 2 hr, washed three times, and stained the block with 0.5% uranyl acetate, all in PBS. The cells were then embedded in Epon 812 after dehydration in an ethanol series. Ultrathin sections were collected on Formvar/carbon-coated nickel grids, which were then floated on drops of freshly prepared 3% sodium metaperiodate for 30 min. The immunogold labeling procedure was modified from Spector et al. (15). After etching and washing, the grids were placed on 50-µL droplets of solution A (phosphate saline solution, pH 8.2, containing 4% normal goat serum, 1% bovine serum albumin, 0.1% Tween 20, 0.1% sodium azide) for 30 min. Grids were then incubated for 2 hr at room temperature in a humidified chamber on 50-µL droplets of the anti-human COX-2 and anti-rat Cav-3 antibodies appropriately diluted in solution B (solution A but with 1% normal goat serum) followed by rinses in solution B. The grids were reacted with the 10-nm gold-conjugated goat anti-mouse IgG or 15-nm gold-conjugated rabbit anti-goat IgG diluted in solution A. After washes in PBS and deionized water, the grids were stained with uranyl acetate (7 min) and lead citrate (2 min) and were viewed with a Hitachi H-7100 (Tokyo, Japan) electron microscope.
As the first step of the current study, we have characterized primarily cultured rat chondrocytes by using RT-PCR and Western blot analysis with employing type II collagen and aggrecan as the chondrogenic markers. Fig. 1A shows the light microscopic morphology of the primarily cultured rat chondrocytes. Fig. 1B, C shows the mRNA and protein expression of the chondrogenic marker. From these results, the cells used in the current study had the characteristics of chondrocytes.
We examined the expressions of COX-2 and Cav-3 mRNAs and proteins in the normal or Cd-treated rat chondrocytes. The results shown in Fig. 2 indicate that the expressions of Cav-3 mRNA and protein were observed in the un-stimulated rat chondrocytes. In the un-stimulated rat chondrocytes, the level of COX-2 mRNA and the protein expression were very weakly detected (Fig. 2). However, when the rat chondrocytes were stimulated with Cd (5 µM) for 6 hr, the expression of COX-2 mRNA and protein markedly increased to the maximal level in a dose- and time-dependent manner (data not shown). The expressions of Cav-3 mRNA and protein were decreased by the treatment with Cd.
Fig. 3 shows the results of the Western blot analysis using the antibodies for COX-2 or Cav-3 on the caveolae-enriched membrane fractions that were obtained from the sucrose density gradient centrifugations of the stimulator-treated rat chondrocytes. The COX-2 protein was observed in the fractions numbered 4 and 5. These membrane fractions also contained Cav-3 protein (Fig. 3).
To determine whether there is a direct binding between the COX-2 and Cav-3 in the detergent-resistant membrane fractions, lysates of the rat chondrocytes were stimulated with Cd (5 µM for 6 hr), and the purified GST fusion proteins were initially immuno-precipitated with the antibodies directed against COX-2 or Cav-3. Subsequently, the precipitates were electrophoresed by SDS-PAGE and the separated proteins were transferred to a membrane. The formation of a complex between the COX-2 and Cav-3 was analyzed by performing Western blot analysis with using the designated antibodies. The COX-2 or Cav-3 proteins did not precipitate with the control IgG (Fig. 4A, B, designated none). As shown in Fig. 4, the presence of the COX-2 and Cav-3 complex (Fig. 4A) was detected upon precipitation with COX-2 antibody. The presence of the COX-2 and Cav-3 complexes could also be detected following precipitation with the Cav-3 antibody (Fig. 4B). The purified GST fusion proteins also formed complexes with each other without forming a complex with the immunoprecipitates of the control IgG (data not shown). This result is consistent with their co-localization (Fig. 3), as well as the direct binding between COX-2 and Cav-3 in the caveolae-enriched membrane fractions.
To confirm the localization of COX-2 and Cav-3 in the rat chondrocytes treated with Cd (5 µM for 6 hr), the cells were observed with confocal and electron microscopes by separately staining with the respective antibodies of COX-2 and Cav-3 and with using fluorescence- or gold particle-conjugated secondary antibodies. The confocal microscopic results showed that the immuno-reactivity of COX-2 was detected in the cytoplasm and plasma membrane (Fig. 5A-b), and the immuno-reactivity of Cav-3 was detected at the plasma membrane of the cultured chondrocytes (Fig. 5A-a). The merged image (orange color) showed that at least some portion of COX-2 and Cav-3 were co-localized in the plasma membrane (Fig. 5A-c). No single effects of secondary antibodies were detected (Fig. 5A-d, e). The 15-nm gold particles for COX-2 were observed in all portions of the cells containing the caveolae structures. In contrast, the 10-nm gold particles for Cav-3 were observed only on the surface- or intracellular-caveolae structures (Fig. 5B).
The results obtained in the present study demonstrate for the first time that some portion of the COX-2 protein is bound to Cav-3 protein and the bound complex is co-localized in the caveolae-enriched plasma membrane fractions of the primarily cultured rat chondrocytes. Several lines of evidences that were provided in the current study support this conclusion: 1) Western blot analysis of COX-2 indicated that it is present in the caveolae-enriched membrane fraction along with Cav-3, 2) the cross-over immuno-precipitation results show that the COX-2 protein formed a bound complex with the Cav-3 protein, and 3) with using confocal and electron microscopes, the immunocytochemistry images show that at least some portion of the COX-2 is co-localized with Cav-3 in the plasma membrane.
Articular joint degradation is a common feature of rheumatoid arthritis and osteoarthritis. Prostaglandins (PG) are important regulatory factors in normal cartilage metabolism and in the pathogenesis of osteoarthritis (OA) and inflammatory disorders (16, 17). The cyclooxygenase-2 dependent PGE2 was found to be the most abundant PG in the synovial fluid of rheumatoid arthritis and OA patients, and it has been demonstrated to mediate joint inflammation and the degradation of the cartilage matrix components (17-19). It is interesting that an alternative set of PGs, namely PGD2 and its metabolites, was recently proposed to have anti-inflammatory properties (20), and to act as a negative feedback loop for joint inflammation and cartilage catabolism (21). These observations increased the scientific interest in the possible differential effects of PGs on chondrogenesis with its potential implications for the treatment of cartilage pathologies. Chondrocytes are differentiated from mesenchymal cells during the embryo's development (22, 23). The phenotypes of differentiated chondrocytes in articular cartilage are characterized by the synthesis and maintenance of cartilage-specific extracellular matrix molecules, including type II collagen and the proteoglycans such as aggrecan. As shown in Fig. 1, the chondrocytes used in the current study well expressed type II collagen and aggrecan at the mRNA and protein levels.
Caveolae were originally described in the endothelial cells as flask-shaped vesicular invaginations in the plasma membrane, and they are rich in cholesterol, glycosphingolipids and sphingomyelin, and then are not solubilized by detergents. The membrane receptor associated signaling molecules (like the G-protein and the Src family kinases) or the membranous enzymes (such as endothelial nitric oxide synthase, heme oxygenase and cyclooxygenases) are anchored to the caveolae via the caveolins and the caveolae are implicated to function as the portals of signal transduction and the vesicular transport processes (24, 25). These membrane-associated signaling molecules are known to contain a common amino acid sequence motif (i.e., ψXψXXXXψ or ψXXXXψXXψ, in which ψ designates aromatic amino acids like Trp (W), Phe (F) or Tyr (Y) and X can be any amino acids) that recognizes the scaffolding domains that are present in the caveolins. Our Western blot analysis results for the caveolae-rich membrane fraction and the formation of immuno-precipitates by the respective antibodies strongly suggest that there might be protein-protein interaction between COX-2 and Cav-3.
COX-2 is a membrane-bound hemoglycoprotein that is mainly found in the endoplasmic reticulum. As the confocal and electron microscopy results have shown, most of the COX-2 is distributed in the intracellular portion (Fig. 5). However, some portion of the COX-2 is located in the plasma membrane in association with the caveolins. Caveolins are a family of integral membrane proteins that are contained in the caveolae. The caveolin family consists of Cav-1, Cav-2 and Cav-3 (26, 27). Normally, Cav-1 and Cav-2 are co-expressed and they form a hetero-oligomeric complex in many cell types (28, 29), but Cav-3 is known to be specific for muscle cells (30). With respect to the tissue distribution of Cav-3, there have been two current investigations that have demonstrated the expression of Cav-3 in other tissues. One of these is the brain astroglial cells and the other is the chondrocytes (11, 12). An unexpected interesting result from the current study was the expressional change of Cav-3. Except for very rare cases, the caveolin expression was not changed. Further study on the changes of Cav-3, according to Cd treatment, will be necessary.
For the molecular aspect of the protein-protein interaction between COX and the caveolins, Liou and co-workers have reported that COX-2 was co-localized and interacted with caveolin-1 (9). Our unpublished data showed that some portion of COX-2 was also co-localized in the plasma membrane to form a complex with Cav-2. In addition to COX-2, we have reported that COX-1 was also co-localized and interacted with Cav-1 and/or Cav-2. This suggests that caveolins have some regulatory effect on the functions of COX.
The COX system is known to be a microsomal enzyme system that is mainly located in the endoplasmic reticulum. The reasons for this un-expected co-localization of COX-2 in the caveolae by its binding to Cav-3 are not clear. One possibility is the regulation of COX-2 retention in the endoplasmic reticulum by a post-translational mechanism, and this idea needs further study. Further investigations on the regulatory characteristics of the caveolins in cells, such as the sorting mechanism of COX-2 to the caveolae, and on the difference between the microsomal and plasma membranous COX activity, as well as the changes of microsomal COX activity upon binding to exogenously added caveolins, may provide us with some important clues to the physiology and pathology of COX-2. In addition, it is necessary to clarify the mechanism of the regulatory effect of Cav-3 on COX-2.
Figures and Tables
Fig. 1
Morphology and characterization of primarily cultured rat chondrocytes. (A) Light microscopy of confluently grown chondrocytes. (B) Messenger RNA expression of type II collagen and aggrecan in the cultured rat chondrocytes. Total RNA (500 ng) isolated from rat chondrocytes was reverse transcribed and polymerase chain reaction was performed (35 cycles). PCR products were separated using 1% agarose gel and stained with ethidium bromide. (C) Isolated membrane protein (30 µg/lane) was separated by electrophoresis and presence of each type II collagen and aggrecan was detected with respective type II collagen and aggrecan antibodies. Data was shown typical result from 3 independent experiments. M, size marker; C, type II collagen; A, aggrecan.
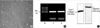
Fig. 2
Expression of COX-2 and Cav-3 mRNA and protein in primarily cultured rat chondrocytes. Cells were changed with medium containing 0.5% FBS in order to exclude serum effect prior to 12 hr of Cd treatment. Then, cells were treated with 5 µM of CdCl2 for 6 hr. After treatment, cells were collected with Tri Reagent for RT-PCR and with lysis buffer for Western blot analysis. The conditions of RT-PCR and Western blotting were same as those of Fig. 1. The COX-2 and Cav-3 antibodies (in Box). Data was shown typical result from 3 independent experiments. ctl; control, Cd; cadmium treated.
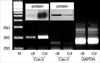
Fig. 3
Western blot analysis of COX-2 and Cav-3 in caveolae-rich membrane fraction. Fractions numbered 4 and 5 are the detergent resistant caveolae-rich fractions obtained by sucrose density gradient centrifugations and the fractions numbered 9 to 12 are the detergent soluble membrane fractions. Fractions obtained from sucrose density gradient centrifugation of detergent solubilized rat chondrocytes were subjected to Western blot analysis with respective antibodies. The result was revealed from 2 separate experiments.
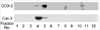
Fig. 4
Complex formation between COX-2 and Cav-3 in the rat chondrocytes. Cell lysates and purified GST fusion proteins (incubated for 2 hr at 37℃ were immunoprecipitated initially with antibodies of COX-2 or Cav-3 and the respective immunoprecipitated (IP) proteins (15 µg) were loaded onto each lane of a 10% SDS-polyacrylamide gel. After separation of the proteins by electrophoresis and transferring the separated proteins to a membrane, the membrane was immunoblotted (IB) using the indicated respective antibodies. Cell lysates were immunoprecipitated initially with COX-2 antibody (A) or Cav-3 antibody (B). The immunoprecipitational result for GST fusion proteins was shown in (C).

Fig. 5
Confocal and electron microscopy of rat chondrocytes immuno-cytochemically stained with COX-2 and caveolin antibodies. Cells were double stained with fluorescein isothiocyanate- and Texas Red-conjugated secondary antibodies after an initial treatment with either Cav-3 or COX-2 antibodies separately and then examined under a confocal microscope at their respective wavelengths. For each experiment, a least 50 cells were examined and the presented images represent typical staining pattern for the majority of examined cells. a, Cav-3; b, COX-2; c, merged image of a and b; d and e; stained only with secondary antibodies without the prior treatment of either Cav-3 or COX-2 antibodies (A). Cells were double stained with different size of gold particle-conjugated secondary antibodies after an initial treatment with either Cav-3 or COX-2 antibodies separately and then examined under an electron microscope. Arrow showed structure of caveolae. # and * represented COX-2 (15 nm particles) and Cav-3 (10 nm particles), respectively.
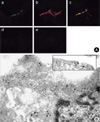
References
1. Nasrallah R, Hebert RL. Prostacyclin signaling in the kidney: implications for health and disease. Am J Physiol. 2005. 289:235–246.


2. Smith WL, Garavito RM, DeWitt DL. Prostaglandin endoperoxide H synthases (cyclooxygenase)-1 and -2. J Biol Chem. 1996. 271:33157–33160.
3. Rodrigues S, Bruyneel E, Rodrigue CM, Shahin E, Gespach C. Cyclooxygenase 2 and carcinogenesis. Bull Cancer. 2004. 91:S61–S76.
4. Park MK, Hwang SY, Kim JO, Kwack MH, Kim JC, Kim MK, Sung YK. NS398 inhibits the growth of Hep3B human hepatocellular carcinoma cells via caspase-independent apoptosis. Mol Cells. 2004. 17:45–50.
5. O'Neill GP, Ford-Hutchinson AW. Expression of mRNA for cyclooxygenase-2 and cyclooxygenase-2 in human tissues. FEBS Lett. 1993. 330:156–160.
6. Wu KK. Cyclooxygenase 2 induction: molecular mechanism and pathophysiologic roles. J Lab Clin Med. 1996. 128:242–245.


7. Williams TM, Lisanti MP. The caveolin genes: from cell biology to medicine. Ann Med. 2004. 36:584–595.


8. Liu P, Rudick M, Anderson RG. Multiple functions of caveolin-1. J Biol Chem. 2002. 277:41295–41298.


9. Liou JY, Deng WG, Gilroy DW, Shyue SK, Wu KK. Colocalization and interaction of cyclooxygenase-2 with caveolin-1 in human fibroblasts. J Biol Chem. 2001. 276:34975–34982.


10. Cha SH, Jung NH, Kim BR, Kim HW, Kwak JO. Evidence for cyclooxygenase-1 association with caveolin-1 and -2 in cultured human embryonic kidney (HEK 293) cells. IUBMB Life. 2004. 56:221–227.
11. Ikezu T, Ueda H, Trapp BD, Nishiyama K, Sha JF, Volonte D, Galbiati F, Byrd AL, Bassell G, Serizawa H, Lane WS, Lisanti MP, Okamoto T. Affinity-purification and characterization of caveolins from the brain: differential expression of caveolin-1, -2, and -3 in brain endothelial and astroglial cell types. Brain Res. 1998. 804:177–192.


12. Schwab W, Galbiati F, Volonte D, Hempel U, Wenzel KW, Funk RH, Lisanti MP, Kasper M. Characterisation of caveolins from cartilage: expression of caveolin-1, -2 and -3 in chondrocytes and in alginate cell culture of the rat tibia. Histochem Cell Biol. 1999. 112:41–49.


13. Kiepe D, Andress DL, Mohan S, Standker L, Ulinski T, Himmele R, Mehls O, Tonshoff B. Intact IGF-binding protein-4 and -5 and their respective fragments isolated from chronic renal failure serum differentially modulate IGF-1 actions in cultured growth plate chondrocytes. J Am Soc Nephrol. 2001. 12:2400–2410.
14. Ferretti A, Knijn A, Raggi C, Sargiacomo M. High-resolution proton NMR measures mobile lipids associated with triton-resistant membrane domains in haematopoietic K562 cells lacking or expression caveolin-1. Eur Biophys J. 2003. 32:83–95.
15. Spector DL, Fu XD, Maniatis T. Associations between distinct premRNA splicing components and the cell nucleus. EMBO J. 1991. 10:3467–3481.


16. Harris ED Jr. Rheumatoid arthritis. Pathophysiology and implications for therapy. N Engl J Med. 1990. 322:1277–1289.
17. Hardy MM, Seibert K, Manning PT, Currie MG, Woerner BM, Edwards D, Koki A, Tripp CS. Cyclooxygenase 2-dependent prostaglandin E2 modulates cartilage proteoglycan degradation in human osteoarthritis explants. Arthritis Rheum. 2002. 46:1789–1803.


18. Blanco FJ, Guitian R, Moreno J, de Toro FJ, Galdo F. Effect of anti-inflammatory drugs on COX-1 and COX-2 activity in human articular chondrocytes. J Rheumatol. 1999. 26:1366–1373.
19. Choi YA, Lee DJ, Lim HK, Jeong JH, Sonn JK, Kang SS, Baek SH. Interleukin-1β stimulates matrix metalloproteinase-2 expression via a prostaglandin E2-dependent mechanism in human chondrocytes. Exp Mol Med. 2004. 36:226–232.
20. Gilroy DW, Colville-Nash PR, Willis D, Chivers J, Paul-Clark MJ, Willoughby DA. Inducible cyclooxygenase may have anti-inflammatory properties. Nat Med. 1999. 5:698–701.


21. Fahmi H, Pelletier JP, Martel-Pelletier J. PPARgamma ligands as modulators of inflammatory and catabolic responses in arthritis. An overview. J Rheumatol. 2002. 29:3–14.
23. DeLise AM, Fischer L, Tuan RS. Cellular interactions and signaling in cartilage development. Osteoarthritis Cartilage. 2000. 8:309–334.


24. Li XA, Everson WV, Smart EJ. Caveolae, lipid rafts, and vascular disease. Trends Cardiovasc Med. 2005. 15:92–96.


25. Couet J, Li S, Okamoto T, Ikezu T, Lisanti MP. Identification of peptide and protein ligands for the caveolin-scaffolding domain. Implications for the interaction of caveolin with caveolae-associated proteins. J Biol Chem. 1997. 272:6525–6533.
27. Okamoto T, Schlegel A, Scherer PE, Lisanti MP. Caveolins, a family of scaffolding proteins for organizing "preassembled signaling complexes" at the plasma membrane. J Biol Chem. 1998. 273:5419–5422.


28. Scherer PE, Lewis RY, Volonte D, Engelman JA, Galbiati F, Couet J, Kohtz DS, Donselaar E, Perters P, Lisanti MP. Cell-type and tissue-specific expression of caveolin-2: Caveolins 1 and 2 co-localize and form a stable hetero-oligomeric complex in vivo. J Biol Chem. 1997. 272:29337–29346.
29. Li S, Galbiati F, Volonte D, Sargiacomo M, Engelman JA, Das K, Scherer PE, Lisanti MP. Mutational analysis of caveolin-induced vesicle formation. Expression of caveolin-1 recruits caveolin-2 to caveolae membranes. FEBS Lett. 1998. 434:127–134.
30. Song KS, Scherer PE, Tang Z, Okamoto T, Li S, Chafel M, Chu C, Kohtz DS, Lisanti MP. Expression of Caveolin-3 in Skeletal, Cardiac, and Smooth Muscle Cells. Caveolin-3 is a component of the sarcolemma and co-fractionates with dystrophin and dystrophin-associated glycoproteins. J Biol Chem. 1996. 271:15160–15165.