Abstract
The presence of heparan sulfate proteoglycan (HSPG) in anionic sites in the lamina rara interna of glomerular basement membrane suggests that the proteoglycan may be deposited by the glomerular endothelial cells (GEndo). We have previously demonstrated that bovine GEndo in vitro synthesize perlecan, a species of glomerular basement membrane HSPG. In this study we examined whether high glucose medium regulates the GEndo metabolism of glycopeptides including perlecan. Metabolic labeling of glycoconjugates with 35S-SO4, sequential ion exchange and Sepharose CL-4B chromatography of labeled glycoconjugates, and northern analysis were performed. Incubation of GEndo for 8 to 14 weeks (but not for 1-2 weeks) in medium containing 30 mM glucose resulted in nearly 50% reduction in the synthesis of cell layer and medium 35SO4-labeled low anionic glycoproteins and proteoglycans, including that of basement membrane HSPG (Kav 0.42) compared to GEndo grown in 5 mM glucose medium; no changes in anionic charge density or hydrodynamic size of proteoglycans were noted. Northern analysis demonstrated that the mRNA abundance of perlecan was reduced by 47% in cells incubated with 30 mM glucose. Our data suggest that high glucose medium reduces the GEndo synthesis of perlecan by regulating its gene expression. Reduced synthesis of perlecan by GEndo may contribute to proteinuria seen in diabetic nephropathy.
Pathologic changes in the glomerular extracellular matrix lead to abnormal protein excretion as well as to impairment of glomerular filtration in diabetic nephropathy. Alterations in the diabetic glomerular basement membrane include increase in its thickness and decrease in the density of anionic sites (1). The latter corresponds to reduction in glomerular basement membrane content of heparan sulfate proteoglycan (HSPG), a highly anionic molecule which regulates the chargeand size-selective aspects of glomerular permselectivity (2-4). Ultrastructural studies have demonstrated aggregation of HSPG into anionic sites in both the lamina rara externa and lamina rara interna of glomerular basement membrane (1). Previous reports have shown that glomerular epithelial cells contribute to HSPG present in glomerular basement membrane (5-7). Incubation of glomerular epithelial cells in medium containing high glucose concentraion results in inhibition of synthesis of basement membrane HSPG, perlecan (8) or agrin (9). High glucose medium induced reduction in synthesis of perlecan was found to be mediated by regulation of gene expression of perlecan core protein (10), suggesting a role for glomerular epithelial cells in pathogenesis of proteinuria in diabetic nephropathy.
Perlecan is an important species of HSPG present in the basal laminae including the glomerular basement membrane (11). It is a modular proteoglycan consisting of a large core protein and heparan sulfate glycosaminoglycan (GAG) side chains. Presence of HSPG in the subendothelial zone of the glomerular basement membrane (lamina rara interna) (1) suggests that glomerular endothelial cells (GEndo) may be the source of the proteoglycan. Studies on bovine GEndo have shown that the cells synthesize perlecan and release it into the medium (12). The anionic site density in subendothelial lamina rara interna of glomerular basement membrane is reduced in diabetic nephropathy (1). This suggests that altered metabolic activity of glomerular endothelial cells may be involved in reduction of proteoglycans in the subendothelial aspect of the diabetic glomerulus. Accordingly, we examined whether high glucose medium regulates the GEndo metabolism of basement membrane HSPG, perlecan.
Bovine GEndo were grown and characterized as previously described (12). Cells were grown to confluence and medium changed to either (a) control medium: RPMI medium containing 5 mM glucose, 0.66 unit/mL insulin, 10% fetal bovine serum, 15 mM HEPES, 100 µg/mL streptomycin, 100 U/mL penicillin, or, (b) high glucose medium: RPMI medium containing 30 mM glucose, no added insulin, but with all other additives as for control medium. These conditions were designed to reflect features of type I diabetes, i.e., hyperglycemia and insulin lack. The cells were incubated in an atmosphere of 5% CO2 at 37℃ in control, or high glucose medium for 7 or 14 days for "short term" incubation. In some experiments employing "long term" incubation, GEndo were either grown to confluence and maintained in 5 mM or 30 mM glucose medium, or, were passed every 10-14 days for 5 to 6 times and maintained in the respective media for up to 2 to 3.5 months. The media were changed every 3-4 days. In some experiments cells were grown in medium containing 5 mM glucose and 25 mM mannitol to serve as osmotic control for 30 mM glucose.
At the end of either short or long term incubation, confluent layers of GEndo grown in 12-well dishes were fed fresh control or high glucose medium and labeled for 24 hr with 200 µCi/mL of 35S-SO4 (ICN Biochemicals, Aurora, OH, U.S.A.; specific activity 43 mCi/mg S, carrier free). Kasinath (12) has previously shown that 35S-SO4 incorporation into macromolecules by the GEndo is linear up to 48 hr. Following labeling, the medium and cell layer were extracted as previously described (12). Guanidine HCl was added to the medium at a concentration of 4 M along with the following protease inhibitors: 1 mM phenylmethylsulfonylfluoride, 100 mM 6-aminohexanoic acid and 10 mM benzamidine HCl. Cell layers were extracted overnight at 4℃ in 4 M guanidine HCl containing 2% (3-[3-cholamidopropyl]-dimethylammonio)-1-propane sulfate) (CHAPS) and protease inhibitors. Medium and layer extracts were stored at -20℃ until further analysis. Protein content of the cell monolayers in parallel wells (n=4 in each experiment) incubated in the respective media was estimated by using a bicinchonic acid reagent kit (Pierce, Rockford, IL, U.S.A.), with bovine serum albumin as standard.
The 35S-labeled macromolecules were separated from unincorporated free label by Sephadex G-25 chromatography using pre-packed PD:10 columns (Pharmacia, Peepack, NJ, U.S.A.). The elution buffer consisted of 6 M urea, 0.15 M NaCl, 0.05 M sodium acetate, protease inhibitors (as above), and, 0.5% CHAPS, pH 5.8 (12). Aliquots of fractions were counted in liquid scintillation medium (Aquassure, NEN Research, Boston, MA, U.S.A.), and the excluded fractions were pooled for analysis.
35S-labeled proteoglycans were separated from low anionic labeled macromolecules by DEAE-Sephacel chromatography. Equal amounts (cpm) of pooled 35S-labeled cell or medium macromolecules from each incubation condition were applied to a DEAE-Sephacel column of 2 mL bed volume, and eluted in 1 mL fractions along a linear NaCl gradient employing 6 M urea buffer (see above) containing 0.15 M NaCl and 1.5 M NaCl as start and limit buffers, respectively. Protease inhibitors were included in both buffers. Gradient development was monitored by measurement of conductivity of fractions using CDM-80 instrument (Radiometer, Copenhagen, Denmark). The fractions that elute at greater than 0.3 M NaCl were pooled as proteoglycans (14).
Hydrodynamic size characteristics of proteoglycans were studied by Sepharose CL-4B chromatography. Equal amounts (cpm) of cell or medium labeled proteoglycans from each condition of incubation were eluted in 1 mL fractions from a 100×0.65-cm column of Sepharose CL-4B under dissociated conditions employing 4 M guanidine-HCl, 50 mM Tris-HCl, pH 7.4, 0.5% Triton X-100 buffer containing protease inhibitors. Aliquots of fractions were counted and fractions corresponding to the peaks were pooled. The hydrodynamic size of proteoglycans was calculated as Kav=Ve-Vo/Vt-Vo, were Vo is the void volume as determined by blue dextran elution, Vt is the total volume as determined by elution of free 3H-glucosamine, and Ve is the elution volume of labeled proteoglycan.
Proteoglycans in each peak of Sepharose CL-4B chromatography were divided into three aliquots, and subjected to chondroitinase-ABC or nitrous acid digestion as previously described (8, 12); the third untreated aliquot served as a control. These conditions of have been shown to take enzymatic digestions to completion (8, 12, 13). Following GAG digestion gel chromatography was performed using a G:50 Sephadex column with PBS as the elution buffer and relative proportions of chondroitin/dermatan sulfate and heparan sulfate GAGs were determined.
Total RNA was extracted from GEndo incubated with 5 mM glucose, 30 mM glucose, or 5 mM glucose +25 mM mannitol medium (10, 15). RNA samples were quantified by spectrophotometry and their integrity confirmed by electrophoresis on 1% agarose gels. Equal amount of total RNA (15 µg) from GEndo incubated with the respective media were fractionated on a 1% agarose, 2.2 M formaldehyde gel, transferred to Nytran membrane using a TurboBlotter system (Schleicher and Schuell, Keene, NH, U.S.A.), and cross-linked by ultraviolet light. The membrane was prehybridized in a buffer containing 50% formamide, 5×SSC, denatured salmon sperm DNA 150 µg/mL, sodium pyrophosphate-EDTA, at 42℃ with 1-2×106 cpm/mL of RPD-1, a partial cDNA for rat perlecan (15). The blot was washed two times in 2×SSC, 0.1% SDS for 15 min at 45℃ and once in 0.2×SSC, 0.1% SDS for 15 min at 50℃. The blot was autoradiographed on an X-omat film using intensifying screens. The membrane was stripped by boiling in 1% SDS, 10 mM EDTA, 10 mM Tris buffer for 10 min and reprobed with a cDNA for GAPDH, as described above. The intensity of bands was measured by laser densitometric scanning (Hoefer Scientific Instruments, San Francisco, CA, U.S.A.).
Following 24 hr of labeling in control medium approximately 47% of the total 35S-labeled macromolecules appeared in the medium, the rest being retained in the cell layer. The relative distribution of labeled macromolecules between the medium and the cell layer compartments was unaffected by incubation in high glucose medium (Table 1).
Following long term incubation (8 to 14 weeks) in high glucose medium, the net synthesis of 35S-labeled macromolecules was reduced by approximately 53% and 50% (p<0.05) in both the medium and cell layer compartments, respectively. Incubation in 30 mM glucose medium for 7 or 14 days did not affect incorporation of 35S-SO4 into macromolecules (data not shown). Careful attention was paid to possible untoward effects of prolonged incubation periods on GEndo. Incorporation of 35S-SO4 into macromolecules by GEndo incubated in control medium was quantitatively similar to our previous observations with GEndo (12), suggesting that the cells were metabolically active despite prolonged incubation. Protein contents of cell layers did not differ significantly between control and 30 mM glucose treated cell layers at the end of incubation periods, i.e., 399±33.6 µg/well (control, 30 mM glucose) vs. 445±38.5 µg/well (30 mM glucose, Table 1). Finally, morphological examination at the end of prolonged incubation did not reveal any difference in appearance between cells grown in control or the high glucose media, the cells was not noted on careful examination of the monolayers every three days for the duration of incubation. The profile of proteoglycans on molecular sieve chromatography was similar to that reported in pure populations of GEndo (12), further confirming absence of emergence of new cells with a different proteoglycan profile. There was no evidence of excessive cell detachment in cell layers incubated with 30 mM glucose. These observations show that prolonged incubation with 30 mM glucose did not affect the viability of GEndo compared to cell layers incubated for the same duration with medium containing 5 mM glucose (Fig. 1).
On ion exchange chromatography, 35S-labeled macromolecules in both medium and cell layer of cells incubated with 5 mM glucose resolved into 2 peaks. Peak A appeared at a molarity close to that of start buffer (0.15 M NaC1) whereas peak B eluted at a molarity greater than 0.3 M NaC1 (Fig. 2, 3).
Proteoglycans accounted for the majority of labeled macromolecules in both medium and cell layer compartments. The relative distribution of labeled macromolecules into peaks A and B was not altered in GEndo incubated with 30 mM glucose. However, high glucose medium induced a significant 53.5% reduction in the synthesis of 35S-labeled low anionic macromolecules, i.e., glycoproteins (peak A) in cell layer compartment; although a 47% decrease was seen in the synthesis of labeled glycoproteins in the medium, it did not achieve statistical significance (Table 1).
A significant reduction in the synthesis of 35S-labeled proteoglycans was evident when cells were incubated with 30 mM glucose (Table 1) which was estimated at 56% for the medium (31,900±2,270 vs. 14,070±4,000, p<0.01) and at 48% for the cell layer compartment (37,940±4,600 vs. 19,700±5,710, p<0.05). Furthermore, high glucose medium did not alter the anionic charge density of proteoglycans in either the medium or the cell layer, as indicated by elution of peak B at similar molarity of NaC1 (Fig. 2, 3).
On molecular sieve chromatography, medium proteoglycans from GEndo incubated with 5 mM glucose resolved into 2 peaks of Kav 0.18 and 0.42 (Fig. 4). Peak M-I (Kav 0.18) formed 36% of all medium proteoglycans whereas peak M-II (Kav 0.42) accounted for the remainder (Table 2). GAG analysis showed that the majority of proteoglycans in peaks M-I and M-II was made of chondroitin sulfate proteoglycan (76% and 59%, respectively) the remainder consisting of heparan sulfate. The HSPG in peak M-II has the hydrodynamic size of bovine glomerular basement membrane HSPG (Kav 0.44) (16). On incubation with 30 mM glucose, there was no alteration in either the hydrodynamic size of the proteoglycan peaks M-I and M-II, or, their relative distribution (Fig. 4). However, high glucose medium caused a reduction in the synthesis of proteoglycans in peak M-I by 58% (11,430±460 vs. 4,850±1,090, p<0.005) (Table 2). Similarly, a 55% reduction was seen in the synthesis of peak M-II, proteoglycans (20,470±1,820 vs. 9,220±2,910, p<0.05) when GEndo were incubated with 30 mM glucose medium (Table 2). GAG analysis showed that heparan sulfate accounted for 30% and 50% of peaks M-I and M-II respectively. The reduction in synthesis of HSPG in peak M-II that resembles glomerular basement membrane HSPG, perlecan, was estimated at 45%.
Cell layer proteoglycans of GEndo incubated with control medium also resolved into two distinct peaks of Kav 0.24 and 0.67, respectively (Fig. 5). Peak C-I of Kav 0.24 accounted for 18% of all cell layer proteoglycans (Table 2). GAG analysis showed that heparan sulfate and chondroitin/dermatan sulfate accounted for 54% and 46% of GAGs in peak C-I (Kav 0.24), respectively, whereas peak C-II was composed mostly of chondroitin/dermatan sulfate (73%). Incubation with high glucose medium did not alter the hydrodynamic size or the relative proportion of the proteoglycan peaks (Fig. 5). There was a reduction of approximately 26% in the synthesis of peak C-I proteoglycans, which did not reach statistical significance (Table 2). High glucose medium decreased the synthesis of peak C-II proteoglycans significantly by 53% (31,550±5,260 vs. 14,970±4,720, p<0.05) (Table 2). Incubation with high glucose medium did not change the GAG constituent profile of either peak.
As there was a reduction in synthesis of HSPG that resembles GBM HSPG, perlecan, we examined whether regulation of perlecan gene expression could be involved. For northern analysis, we employed RPD-1, a partial cDNA probe for rat perlecan, which shares a high degree of homology with mouse and human perlecan (15). As shown in Fig. 6, bovine GEndo constitutively express perlecan transcript which is approximately 12 kb in size (10). Following incubation with 30 mM glucose for 12 weeks, there was a demonstrable reduction in the mRNA abundance of perlecan. This was estimated to be 47% following correction for RNA loading as assessed by hybridization with the probe for GAPDH. In contrast to the high glucose medium, incubation for identical duration with 5 mM glucose with 25 mM mannitol, employed as an osmotic control for 30 mM glucose, caused a 1.5-fold increase in the mRNA abundance of perlecan in GEndo.
These data demonstrate that prolonged incubation of bovine GEndo with high glucose medium results in a decrease in the synthesis of sulfated glycoproteins and proteoglycans, including perlecan, a glomerular basement membrane HSPG. The synthesis of perlecan is regulated at the level of gene expression. Metabolic effects of high glucose are selective and does not due to an osmotic effect, as equimolar mannitol failed to decrease the mRNA expression of perlecan.
It is generally thought that glomerular epithelial cells are responsible for synthesis of glomerular basement membrane in adult rats (5). Studies employing antibodies against laminin have shown that both glomerular epithelial and endothelial cells are involved in matrix synthesis in the developing rat glomerulus; however, in the adult rat, glomerular epithelial cells become the predominant source for laminin deposited in the basement membrane (17). Endothelial cells are metabolically active cells that are known to contribute to synthesis of extracellular matrix. The proximity of the anionic sites in lamina rara interna to glomerular endothelial cells suggests that GEndo could deposit HSPG in this location (1). Reduction in HSPG in the diabetic glomerulus has been shown to involve its distribution in lamina rara interna (1). Our in vitro observations support that GEndo contribute to reduction of glomerular basement membrane content of perlecan in diabetic nephropathy. The mechanism underlying decreased synthesis involves reduced gene expression although augmented rate of degradation of perlecan cannot be excluded.
There are interesting similarities and differences in the regulation of metabolism of 35S-labeled glycoconjugates between the GEndo and glomerular epithelial cells when incubated in high glucose medium. Following incubation of glomerular epithelial cells for 8 days in medium containing 30 mM glucose, there was an increase in the synthesis of sulfated glycoproteins and proteoglycans present in medium and cell layer (8). The increased synthesis of 35S-labeled glycoproteins in glomerular epithelial cell may contribute to glomerular hypertrophic changes that predominantly involve extracellular matrix. In contrast, high glucose medium caused a selective and significant reduction in synthesis of glomerular basement membrane HSPG by glomerular epithelial cells (8). In comparison with the glomerular epithelial cells, the response of GEndo to high glucose medium was strikingly different. There was a generalized reduction in the synthesis of 35S-labeled glycoproteins and proteoglycans, including the medium HSPG species that resembles perlecan, a glomerular basement membrane HSPG. Additionally, prolonged incubation was needed for reduction in basement membrane HSPG synthesis by GEndo whereas shorter incubation one and eight days was sufficient with glomerular epithelial cells (10). These observations suggest that while the reduction in HSPG content in basement membrane, reported to occur as early as 2 weeks in diabetic nephropathy (18), may be due to changes in glomerular epithelial cell synthesis, the more sustained reduction observed at later stages may involve decrease in the synthesis of HSPG by both epithelial and endothelial cells. It is possible that prolonged incubation in high glucose leads to the formation of advanced glycated end-products (AGE) or, at least, Amadori end-products. Voziyan et al. (19) demonstrated that incubation of BSA with 30 mM glucose at 37℃ resulted in significant increase in the rate of accumulation of Amadori intermediate and the kinetics was characterized by t1/2-9 days. Therefore, GEndo maintained in 5 mM or 30 mM glucose medium for up to 2 to 3.5 months might produce significant amount of Amadori intermediate and AGE. Thus, the observed changes may not be due to direct glucose effects. We recently showed that both high glucose and AGE have reducing effects on the production of perlecan core protein by glomerular epithelial cell in vitro (20). These effects seemed to be additive.
The mechanism by which high glucose medium decreases the GEndo synthesis of glomerular basement membrane HSPG involves regulation at the level of gene expression, analogous to our observations with glomerular epithelial cells (10). The mechanism by which high glucose medium affects the mRNA abundance of HSPG core protein is not known but may involve either reduced transcription and/or shortened half-life of mRNA transcripts. A glucose response element (GRE), with a consensus palindromic sequence CTCGTG, has been identified in the promoter of a variety of glucoseregulated genes including liver-type pyruvate kinase, fatty acid synthase and spot-14 (21-23). GRE is involved in upregulation of expression of the aforementioned genes, whereas glucose inhibits the gene expression of HSPG in GEndo. It is possible there are alternative sites of glucose regulation in the promoter of basement membrane HSPG. Further investigation is needed to identify intermediary steps and effectors involved in genetic regulation of basement membrane HSPG by glucose.
The significance of reduced synthesis of 35S-labeled glycoproteins by GEndo following incubation with high glucose medium is unclear and is in contrast to the increment seen with glomerular epithelial cells (8). Only a few of the sulfated glycoproteins in basement membrane have been identified and include entactin (nidogen) (24-26), laminin, and fibronectin (27). In addition, there are several uncharacterized sulfated glycoproteins in the range of 50-180 kDa (28, 29). Glomerular entactin content is increased in diabetic nephropathy (26). Recent observations from our laboratory have suggested that mesangial laminin content is increased in nephropathy in rats with streptozotocin-induced diabetes and db/db mice with spontaneous type II diabetes (30). Additional studies are needed to identify the sulfated glycoproteins synthesized by GEndo. It is possible that the content of some of the hitherto unidentified sulfated glycoproteins is reduced in the diabetic glomerular basement membrane.
The reduction of HSPG synthesis by GEndo could contribute to proteinuria in diabetic nephropathy. Vernier et al. (1) have demonstrated a significant linear relationship between urinary albumin excretion and heparan sulfate enriched anionic sites in lamina rara interna in diabetic nephropathy. The mechanisms underlying dysregulation in GEndo HSPG metabolism are unknown and could include derangements in polyol metabolism (31, 32), nonenzymatic glycation (33) or cytokines such as TGF-β (34). Our data suggest that GEndo in vitro are an appropriate model to further investigate mechanisms involved in dysregulation of basement membrane HSPG synthesis in diabetic nephropathy.
Figures and Tables
Fig. 1
Morphology of GEndo undergoing prolonged incubation in control medium containing 5 mM and 30 mM glucose.
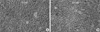
Fig. 2
Ion exchange chromatography of medium 35S-labeled macromolecules. Confluent layers of GEndo were incubated in medium containing (A) 5 mM glucose or (B) 30 mM glucose for 8 to 14 weeks and labeled with 200 µCi/mL of 35SO4 for 24 hr. Fractions containing proteoglycans in peaks B were pooled as indicated by bars.
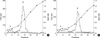
Fig. 3
Ion exchange chromatography of cell layer 35S-labeled macromolecules. GEndo were incubated in medium containing (A) 5 mM glucose or (B) 30 mM glucose for 8 to 14 weeks and labeled for 24 hr with 35SO4 as in Fig. 2. Fractions containing proteoglycans in peaks B were pooled as indicted by bars.
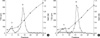
Fig. 4
Sepharose CL-4B chromatography of medium proteoglycans. Equal amounts (cpm) of 35S-labeled proteoglycans purified on ion exchange chromatography (peaks B in Fig. 1) were subjected to Sepharose CL-4B chromatography using 4 M guanidine-HCI buffer containing protease inhibitors. (A) medium proteoglycans from cells incubated with 5 mM glucose, (B) medium proteoglycans from cells incubated with 30 mM glucose. Peaks M-I and M-II were pooled as indicated by bars for further analysis.
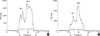
Fig. 5
Sepharose CL-4B chromatography of cell layer proteoglycans. Equal amounts (cpm) of 35S-labeled proteoglycans purified on ion exchange chromatography (peaks B in Fig. 2) were subjected to Sepharose CL-4B chromatography using 4 M guanidine-HCI buffer containing protease inhibitors. (A) cell layer proteoglycans from cells incubated with 30 mM glucose. Peaks C-I and C-II were pooled as indicated by bars for further analysis.
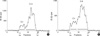
Fig. 6
Regulation of basement membrane HSPG, perlecan, mRNA abundance in GEndo by high glucose medium. Top panel shows bands of hybridization with RPD-1, a cDNA for perlecan, basement membrane HSPG. Lower panel shows hybridization bands with GAPDH probe.
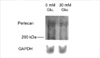
ACKNOWLEDGMENT
We thank Mrs. Carolyn Bryan for excellent secretarial assistance. This work was supported by grants from Veterans' Administration (BSK), and the American Diabetes Association (BSK), the NIH DK 33665 (HEA), Korean Ministry of Health and Welfare (HMP-98-M-5-0053), and Korea Science and Engineering Foundation (KOSEF, R01-2002-000-00251-0).
References
1. Vernier RL, Steffes MW, Sisson-Ross S, Mauer SM. Heparan sulfate proteoglycan in the glomerular basement membrane of type I diabetes mellitus. Kidney Int. 1992. 41:1070–1080.
2. Kanwar YS, Linker A, Farquhar MG. Increased permeability of the glomerular basement membrane to ferritin after removal of glycosaminoglycans (heparan sulfate) by enzyme digestion. J Cell Biol. 1980. 86:688–693.


3. Rosenzweig LJ, Kanwar YS. Removal of sulfated (heparan sulfate) and nonsulfated (hyaluronic acid) glycosaminoglycans results in increased permeability of the glomerular basement membrane to 125I-bovine serum albumin. Lab Invest. 1982. 47:177–184.
4. Kasinath BS, Kanwar YS. Rohrbach DH, Timpl R, editors. Glomerular Basement Membrane: Biology and Physiology. Molecular and Cellular Aspects of Basement Membrane. 1993. San Diego: Academic Press;89–106.


5. Stow JL, Sawada H, Farquhar MG. Basement membrane heparan sulfate proteoglycans are concentrated in the laminae rarae and in podocytes of the rat renal glomerulus. Proc Natl Acad Sci USA. 1985. 82:3296–3300.


6. Thomas GJ, Jenner L, Mason RM, Davies M. Human glomerular epithelial cell proteoglycans. Arch Biochem Biophys. 1990. 278:11–20.


7. Klein DJ, Oegema TR Jr, Fredeen TS, van der Woude F, Kim Y, Brown DM. Partial characterization of proteoglycans synthesized by human glomerular epithelial cells in culture. Arch Biochem Biophys. 1990. 277:389–401.


8. Kasinath BS, Block JA, Singh AK, Terhune WC, Maldonado R, Davalath S, Kallgren MJ, Wanna L. Regulation of rat glomerular epithelial cell proteoglycans by high glucose medium. Arch Biochem Biophys. 1994. 309:149–159.
9. Yard BA, Kahlert S, Engelleiter R, Resch S, Waldherr R, Groffen AJ, van den Heuvel LP, van der Born J, Berden JH, Kroger S, Hafner M, van dervWoude FJ. Decreased glomerular expression of agrin in diabetic nephropathy and podocytes, cultured in high glucose medium. Exp Nephrol. 2001. 9:214–222.


10. Kasinath BS, Grellier P, Choudhury GG, Abboud SL. Regulation of basement membrane heparan sulfate proteoglycan, perlecan, gene expression in glomerular epithelial cells by high glucose medium. J Cell Physiol. 1996. 167:131–136.


11. Iozzo RV, Murdoch AD. Proteoglycans of the extracellular environment: clues from the gene and protein side offer novel perspectives in molecular diversity and function. FASEB J. 1996. 10:598–614.


12. Kasinath BS. Glomerular endothelial cell proteoglycans: Regulation by TGFβ-1. Arch Biochem Biophys. 1993. 305:370–377.
13. Kasinath BS. Effect of insulin on high glucose medium induced changes in rat glomerular epithelial cell metabolism of glycoconjugates. Arch Biochem Biophys. 1995. 318:286–294.
14. Yanagishita M, Midura RJ, Hascall VC. Proteoglycans: Isolation and purification from tissue cultures. Methods Enzymol. 1987. 138:279–289.
15. Ko CW, Bhandari B, Yee J, Terhune WC, Maldonado R, Kasinath BS. Cyclic AMP regulates basement membrane heparan sulfate proteoglycan, perlecan, metabolism in rat glomerular epithelial cells. Mol Cell Biochem. 1996. 162:65–73.


16. Parthasarathy N, Spiro RG. Isolation and characterization of the heparan sulfate proteoglycan of the bovine glomerular basement membrane. J Biol Chem. 1984. 259:12749–12755.


17. Abrahamson DR. Origin of the glomerular basement membrane visualized after in vivo labeling of laminin in newborn rat kidneys. J Cell Biol. 1981. 100:1988–2000.


18. Young BA, Johnson RJ, Alpers CE, Eng E, Gordon K, Floege J, Couser WG, Seidel K. Cellular events in the evolution of experimental diabetic nephropathy. Kidney Int. 1995. 47:935–944.


19. Voziyan PA, Khalifah RG, Thibaudeau C, Yildiz A, Jacob J, Serianni AS, Hudson BG. Modification of proteins in vitro by physiological levels of glucose: pyridoxamine inhibits conversion of Amadori intermediate to advanced glycation end-products through binding of redox metal ions. J Biol Chem. 2003. 278:46616–46624.
20. Ha T-S, Kim H-S. Effects of advanced glycation endproducts on rat glomerular epithelial cells: Roles of reactive oxygen species. Korean J Nephrol. 2003. 22:285–293.
21. Thompson KS, Towle HC. Localization of the carbohydrate response element of the rat L-type pyruvate kinase gene. J Biol Chem. 1991. 266:8679–8682.


22. Shin HM, Towle HC. Definition of carbohydrate response element of the rat S14 gene. Evidence for a common factor required for carbohydrate regulation of hepatic genes. J Biol Chem. 1992. 267:13222–13228.
23. Vaulont S, Kahn A. Transcriptional control of metabolic regulation genes by carbohydrates. FASEB J. 1994. 8:28–35.


24. Carlin B, Jaffe R, Bender B, Chung AE. Entactin, a novel basal lamina-associated glycoprotein. J Biol Chem. 1981. 256:5209–5214.
25. Timpl R, Dziadek M, Fujiwara S, Nowack H, Wick G. Nidogen: a new self-aggregating basement membrane protein. Eur J Biochem. 1983. 137:455–465.


26. Katz A, Fish AJ, Kleppel MM, Hagen SG, Michael AF, Butkowski RJ. Renal entactin (nidogen): isolation, characterization and tissue distribution. Kidney Int. 1991. 40:643–652.


27. Hogan BL, Taylor A, Kurkinen M, Couchman JR. Synthesis and localization of two sulphated glycoproteins associated with basement membranes and the extracellular matrix. J Cell Biol. 1982. 95:197–204.


28. Cohen MP, Wu VY, Surma ML. Non-collagen protein and proteoglycan in renal glomerular basement membrane. Biochem Biophys Acta. 1981. 678:322–328.


29. Lemkin MC, Farquhar MG. Sulfated and nonsulfated glycosaminoglycans and glycopeptides are synthesized by kidney in vivo and incorporated into glomerular basement membranes. Proc Natl Acad Sci USA. 1981. 78:1726–1730.


30. Ha T-S, Barnes JL, Stewart JL, Ko CW, Miner JH, Abrahamson DR, Sanes JR, Kasinath BS. Regulation of renal laminin in mice with type II diabetes. J Am Soc Nephrol. 1999. 10:1931–1939.


31. Tilton RG, Chang K, Pugliese G, Eades DM, Province MA, Sherman WR, Kilo C, Williamson JR. Prevention of hemodynamic and vascular albumin filtration changes in diabetic rats by aldose reductase inhibitors. Diabetes. 1989. 38:1258–1270.


32. Pugliese G, Tilton RG, Speedy A, Santarelli E, Eades DM, Province MA, Kilo C, Sherman WR, Williamson JR. Modulation of hemodynamic and vascular filtration changes in diabetic rats by dietary myo-inositol. Diabetes. 1990. 39:312–322.


33. Vlassara H, Bucala R, Striker L. Pathogenic effects of advanced glycosylation: biochemical, biological and clinical implications for diabetes and aging. Lab Invest. 1993. 70:138–151.
34. Ziyadeh FN, Sharma K, Ericksen M, Wolf G. Stimulation of collagen gene expression and protein synthesis in murine mesangial cells by high glucose is mediated by autocrine activation of transforming growth factor-β. J Clin Invest. 1994. 93:536–542.