Abstract
Purpose
To establish a pH measurement system for a mouse tumor study using a clinical scanner, to develop the 1H and 31P radio frequency (RF) coil system and to test pH accuracy with phantoms.
Materials and Methods
The 1H and the 31P surface coils were designed to acquire signals from mouse tumors. Two coils were positioned orthogonally for geometric decoupling. The pH values of various pH phantoms were calculated using the 1H decoupled 31P MR spectrum with the Henderson-Hasselbalch equation. The calculated pH value was compared to that of a pH meter.
Results
The mutual coil coupling was shown in a standard S12. Coil coupling (S12) were -73.0 and -62.3 dB respectively. The signal-to-noise ratio (SNR) obtained from the homogeneous phantom 1H image was greater than 300. The high resolution in vivo mice images were acquired using a 31P-decoupled 1H coil. The pH values calculated from the 1H-decoupled 31P spectrum correlated well with the values measured by pH meter (R2=0.97).
Many tumor studies have been performed with high magnetic field animal magnetic resonance (MR) scanners using magnetic resonance imaging (MRI) or magnetic resonance spectroscopy (MRS) of 1H and other nuclei such as 31P, 13C, and 19F (1, 2, 3, 4, 5, 6). These MRI/MRS provide some information on tumor microenvironment. MRS is especially useful in studying metabolism but can also be utilized to characterize tumor metabolism, pH, hypoxia, drug delivery, treatment efficacy, and apoptosis (1).
Alterations in tissue pH underlie many pathological processes. The capability to image tissue pH in a clinic could offer new ways of detecting disease and response to treatment (7). A change in tumor microenvironment pH compared to that in normal tissue is a well-recognized phenomenon. The slightly acidic pH in tumor microenvironments has become an important aspect in designing anti-tumor therapy (4, 8). Moreover, pH can have a major impact on successful metastasis, which involves several pH-sensitive steps (9, 10).
Several methods have been proposed for measuring tissue pH including MRS and MRI. Some of these methods exploit endogenous MR resonance, while others require the administration of exogenous agents (7). In human cancers, 31P MRS has been used to endogenously measure pH (11). We propose small animal RF coils to be used in a tumor study with both 1H MRI and 31P MRS. And this 1H-31P coil system for the clinical MR scanner, which is a more popular and convenient than the animal MR scanner.
Technically, proton-decoupling is a necessary requirement to improve low sensitivity 31P MRS (9). The accuracy of the pH values could be increased by the proton-decoupled 31P spectrum. Some reports have suggested arranging coil fields in an orthogonal fashion (12, 13) in order to avoid substantial coil-flux coupling. Bottomley et al. (12) used figure-8 type geometry ('butterfly design') for the 1H decoupling coil, where the proton field was orthogonal to the 13C field. Merkele et al. (13) described a similar design, which was known as a co-planar dual-loop surface coil, consisting of a center-fed loop producing counter-rotating currents in half-loops, thus creating a magnetic field orthogonal to the 13C plane. Although these designs provided good decoupling between the 1H coil and 13C or 31P coil, the proton coil produced a parallel magnetic field intensity that decreased very quickly with distance from the coil plane; therefore, if the region of interest were located at a greater distance from the coil, this design would not provide sufficient proton performance. A newly designed half-volume coil using a lower-frequency coil a significant distance from the protons had been reported to experience blocking field problems (14). This half-volume coil provided -20 dB isolation between the 13C coil and 1H coils (14). Recently, a birdcage-type coil with two independent, separated RF channels was reported by Jeon et al. for 19F imaging (15).
In this study, we sought to confirm properties of the 1H-31P coil system by measuring and comparing pH values using a 31P MRS and a pH meter.
Magnetic resonance is widely used for tumor imaging and spectroscopy. Proton MR imaging was acquired using receive-only coils, whereas the phosphorus MR spectrum was acquired using transmit-and-receive coils.
The proton MR images and phosphorus spectrum were acquired using a 3.0 T GE Discovery MR750 scanner (General Electric Company, Milwaukee, USA).
The 1H and 31P RF coil planes were located orthogonally for geometric decoupling (Fig. 1). The two coils were tuned at 127.74 for protons and 51.75 MHz for phosphorus, matched to 50 Ω, and decoupled from each other. Electromagnetic coupling between two coils was measured using a network analyzer HP8753D (Hewlett Packard, USA).
For the receive-only proton coil, a single 25-mm loop coil etched on a copper PCB (Printed Circuit Board) was used. A non-magnetic PIN diode (MA4P4001, M/A-COM Technology Solution, Lowell, MA, USA) (16) was used to control decoupling. Passive decoupling through the use of a pair of crossed high-speed switching diodes was included as a safety precaution (Fig. 2).
The SNR was determined by the ratio of mean signal intensity to the standard deviation of background noise on phantom image.
The Fast Recovery Fast Spin Echo pulse sequence parameters for proton in vivo mouse images were TR/TE = 3500/89.3 or 88.4 ms, image matrix = 256 × 256, 2 NEX, FOV 12 × 12, slice thickness 4.0 mm. The animal experiment was conducted with the approval of the Association for Assessment and Accreditation of Laboratory Animal Care International.
The 25-mm, two-turn 31P RF coil loops were formed from copper wire, functioning as a transmit-and-receive coil. Impedance matching to 50 ohm was achieved using the balanced capacitive matching method (17).
The single voxel phosphorus spectrum was acquired using an Fid CSI (chemical Shift imaging) MNS (Multi-Nuclear Spectroscopy) pulse sequence (TR = 1500 ms, number of points 2048, total number of scans 128 or 256 (in vivo)). The spectrum analysis was performed with SAGE 7 (Spectroscopy Analysis, GE Healthcare) on an MR750 system.
Intracellular pH was calculated from resonance positioning of Pi with respect to phosphocreatine (PCr) using the following modification of the Henderson-Hasselbalch equation:
The observed Pi position relative to PCr at 0 ppm is represented by δ, and δA and δHA are the respective chemical shifts of the protonated and unprotonated forms of Pi (at 5.7 and 3.23 ppm). The pKa(6.77) is the logarithm of the equilibrium constant for the acid-base equilibrium between protonated and unprotonated forms of Pi (18, 19).
The 1H images and 31P MR spectrum were acquired from a nude mouse (about 23 g) in vivo.
The phosphorus phantom for pH measurement was filled with 100 mM Pi (Na2HPO4), 40 mM phosphocreatine (PCr), and 0.05 mM ethylenediaminetetraacetic acid (EDTA). The volume of each phantom was less than 5 ml. The pH values of the phantoms ranged from 6.4 to 7.6.
The mutual coil coupling between 1H-31P coil was shown in a standard S12 analyzer measurement, transmitting into the 31P coil and receiving with the 1H coil and vice versa. The resulting coupling between the 31P coil and 1H coil is shown in Table 1.
The spin-echo images of a homogeneous phantom are shown in Fig. 3. The SNR of axial images (Fig. 3a) was 300, and that of coronal images (Fig. 3c) was 460.
The high resolution in vivo images acquired with 1H receive-only coils are shown in Fig. 4. The single-voxel 31P MR spectrum was acquired with a calculated pH value of 7.24.
We acquired pH values from MR spectra with various pH phosphorus phantoms. Some of 31P MR spectra are shown in Fig. 5a. The position of the Pi peak relative to that of PCr at 0 ppm shifted depending on pH. With increased pH values, the Pi peak was more distant from the PCr peak at 0 ppm. The pH values were calculated using the Henderson-Hasselbalch equation (Eq. [1]).
The pH values of the same phantom were measured by pH meter (pH 2700, Eutech Instruments). These pH values are plotted in Fig. 5b, where the x-axis represents pH value by pH meter, and the y-axis values represents those calculated from 31P MRS. These values had about 97% correlation.
The tumor microenvironment is significantly different from that of normal tissue and is known for its acidic properties. Tumor acidity has important consequences for therapy and cancer progression (10). MRI/MRS has been used as a common noninvasive modality for studying tumor microenvironments. Most animal tumor studies were performed with high magnetic field MR scanners. But the clinical MR scanner was more familiar and convenient than the animal scanner.
In this study, we attempted to establish a scanning system to measure pH using clinical MR scanner. To distinguish differences in tumor microenvironment, 31P MRS was used. The 1H images and 31P spectrum were acquired using an existing pulse sequence installed on clinical scanner.
For the animal study with a clinical MR scanner, a small RF coil was required to acquire the MR signal with appropriate SNR. Additionally, the proton-decoupled magnetic resonance spectroscopy (MRS) of 31P posed an issue for acquiring the appropriate 31P spectrum. We were able to solve this problem by using a geometric decoupling method. S.I. Babic et al. calculated the mutual inductance between two filamentary circular coils whose centers were not on the same axis (20, 21). If the angle between the two coil planes was 90°, the calculated mutual inductance was 0.
We developed a 1H receive-only coil and a 31P transmit-and-receive coil for an animal study using a clinical scanner. The coil planes were orthogonal to one another, allowing for -60 to -70 dB isolation between 31P coil and 1H coils (Table 1).
1H MR images were acquired with appropriate SNR (greater than 300). High-resolution images and 31P spectrum were obtained with 1H coils and 31P coils, respectively.
To test the 31P MR coil, we compared pH values calculated from the 31P MR spectrum and those directly measured by pH meter. The pH values measured using these two methods had a good correlation (about 97%) (Fig. 4b).
In this study, we developed an animal study system using a clinical scanner and measured pH using this system. If another nuclear surface coil could be used instead of the 31P coil, other metabolic information could be acquired. MRS of other nuclei, such as 31Na, could be acquired by using this simple two-surface coil system.
We developed a 1H and 31P surface coil system for an animal study using a clinical MR scanner. These two coil planes were orthogonally placed, allowing for sufficient decoupling of both coils. Accurate pH values were measured using this 1H-decoupled 31P MRS. This simple two-coil system is an alternative to other nuclear RF coils.
Figures and Tables
Fig. 1
The set-up of 1H and 31P RF coils. The two RF coil planes were located orthogonally for geometric decoupling (a). Photo of a 1H receive-only coil (b) and a 31P transmit-and-receive coil (c).
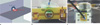
Fig. 2
The 1H receive-only coil scheme. Active decoupling consists of a PIN diode switching design and passive decoupling with crossed high-speed switching diodes and a detuning inductor.
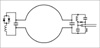
Fig. 3
The 1H spin-echo images of a homogeneous phantom: (a, b) axial images (TR/TE =167/15 ms, FOV 6 × 6 cm2, 256 × 256), (c, d) coronal images (TR/TE = 150/15 ms, FOV 8 × 8 cm2, 256 × 256). The SNR was measured at ROI (gray ellipse) in (a) and (c).
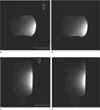
Fig. 4
The T2 weighted in vivo mouse images acquired from a 1H receive-only coil with a Fast Recovery Fast Spin-Echo pulse sequence. The parameters of these images were TR=3500 ms, TE=89.3 (coronal)/88.4 (axial) ms, thickness 4 mm, FOV 12 × 12 cm2, matrix 256 × 256 (coronal), 256 × 224 (axial) 2 NEX. One segment of the scale bar denotes 10 mm. The two white bars show the positions of the 1H and 31P RF coils.
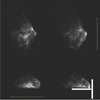
Acknowledgments
This work was supported by a National Research Foundation of Korea (NRF) grant funded by the Korean government, Ministry of Education and Science Technology (MEST) (2012R1A2A1A01011328).
References
1. Glunde K, Bhujwalla ZM. Metabolic tumor imaging using magnetic resonance spectroscopy. Semin Oncol. 2011; 38:26–41.
2. Stubbs M, Bhujwalla ZM, Tozer GM, et al. An assessment of 31P MRS as a method of measuring pH in rat tumours. NMR Biomed. 1992; 5:351–359.
3. Zhou R, Bansal N, Leeper DB, Glickson JD. Intracellular acidification of human melanoma xenografts by the respiratory inhibitor m-iodobenzylguanidine plus hyperglycemia: a 31P magnetic resonance spectroscopy study. Cancer Res. 2000; 60:3532–3236.
4. Gallagher FA, Kettunen MI, Brindle KM. Imaging pH with hyperpolarized 13C. NMR Biomed. 2011; 24:1006–1015.
5. Mason RP, Antich PP, Babcock EE, Gerberich JL, Nunnally RL. Perfluorocarbon imaging in vivo: a 19F MRI study in tumor-bearing mice. Magn Reson Imaging. 1989; 7:475–485.
6. McSheehy PM, Seymour MT, Ojugo AS, et al. A pharmacokinetic and pharmacodynamic study in vivo of human HT29 tumours using 19F and 31P magnetic resonance spectroscopy. Eur J Cancer. 1997; 33:2418–2427.
7. Gillies RJ, Raghunand N, Garcia-Martin ML, Gatenby RA. pH imaging. A review of pH measurement methods and applications in cancers. IEEE Eng Med Biol Mag. 2004; 23:57–56.
8. Iessi E, Marino ML, Lozupone F, Fais S, Milito AD. Tumor acidity and malignancy: novel aspects in the design of antitumor therapy. Cancer Ther. 2008; 6:55–66.
9. Hashim AI, Zhang X, Wojtkowiak JW, Martinez GV, Gillies RJ. Imaging pH and metastasis. NMR Biomed. 2011; 24:582–591.
10. Gillies RJ, Raghunand N, Karczmar GS, Bhujwalla ZM. MRI of the tumor microenvironment. J Magn Reson Imaging. 2002; 16:430–450.
11. Negendank W. Studies of human tumors by MRS: a review. NMR Biomed. 1992; 5:303–324.
12. Bottomley PA, Hardy CJ, Roemer PB, Mueller OM. Proton-decoupled, Overhauser-enhanced, spatially localized carbon-13 spectroscopy in humans. Magn Reson Med. 1989; 12:348–363.
13. Merkle H, Wei HR, Garwood M, Ugurbil K. B1-insensitive heteronuclear adiabatic polarization transfer for signal enhancement. J Magn Reson. 1992; 99:480–494.
14. Adriany G, Gruetter R. A half-volume coil for efficient proton decoupling in humans at 4 Tesla. J Magn Reson. 1997; 125:178–184.
15. WMIC 2013 P266 New Design of dual Tuned RF coil for Fluorine MR Molecular Imaging.
16. Barberi EA, Gati JS, Rutt BK, Menon RS. A transmit-only/receive-only (TORO) RF system for high-field MRI/MRS applications. Magn Reson Med. 2000; 43:284–289.
17. Cassidy PJ, Clarke K, Edwards DJ. Determining the tuning and matching requirements of RF coils using electromagnetic simulation and electric circuit analysis. Concepts Magn Reson Part B Magn Reson Eng. 2005; 25:27–41.
18. De Graaf RA. In vivo NMR Spectroscopy - Principles and Techniques. 2nd ed. John Wiley & Sons Ltd;2007. p. 80–82.
19. Larcombe-McDouall J, Buttell N, Harrison N, Wray S. In vivo pH and metabolite changes during a single contraction in rat uterine smooth muscle. J Physiol. 1999; 518:783–790.
20. Babic SI, Akyel C. Calculating mutual inductance between circular coils with inclined axes in air. IEEE Trans Magn. 2008; 44:1743–1750.
21. Grover FW. Inductance Calculations. New York: Dover;1964. p. 193–208.