Abstract
The aim of this study was to investigate the role of kinesin superfamily member 15 (KIF15) in nasopharyngeal carcinogenesis (NPC) and explore its underlying mechanisms. We employed various assays, including the CCK-8 assay, flow cytometry, the Transwell and scratch assay, Western blotting, and nude mice transplantation tumor, to investigate the impact of KIF15 on NPC. Our findings demonstrate that KIF15 plays a critical role in the proliferation, apoptosis, migration, and invasion of NPC cells. Furthermore, we discovered that silencing KIF15 inhibits cell proliferation, migration, and invasion while promoting apoptosis, and that KIF15's effect on NPC cell growth is mediated through the PI3K/AKT and P53 signaling pathways. Additionally, we showed that KIF15 promotes nasopharyngeal cancer cell growth in vivo. Our study sheds light on the significance of KIF15 in NPC by revealing that KIF15 knockdown inhibits NPC cell growth through the regulation of AKT-related signaling pathways. These findings suggest that KIF15 represents a promising therapeutic target for the prevention and treatment of NPC.
Nasopharyngeal carcinoma (NPC), a malignant epithelial tumor, typically arises in the top and lateral walls of the nasopharyngeal cavity and can invade surrounding tissues. It is a prevalent malignancy in China and exhibits a geographically uneven distribution [1]. Specifically, 92% of new cases occur in economically less developed countries, with at least twice as many new cases occurring in East or Southeast Asia [2]. The World Health Organization classifies NPC into three types based on pathological staging: type I keratinizing squamous carcinoma, type II non-keratinizing squamous carcinoma, and type II non-keratinizing squamous carcinoma, further categorized into differentiated and undifferentiated carcinomas [3]. Early-stage NPC is typically treated with radiotherapy alone, whereas patients with intermediate to advanced stages often receive a combination of radiotherapy and chemotherapy [4]. The incidence rate of NPC is lower in women than in men, although the incidence and mortality rates of NPC increase progressively with age worldwide [5,6]. Recent studies have revealed that targeted therapies can be designed at the cellular and molecular level to specifically target oncogenic sites. These drugs demonstrate high specificity, minimal side effects, and no adverse effects on normal tissue cells surrounding the tumor [7]. However, the intricate signaling pathways that mediate NPC's pathogenesis have not been fully elucidated. As such, exploring the pathological mechanisms underlying nasopharyngeal cancer development and discovering new interventions and therapeutic targets remains of considerable clinical importance and relevance.
The kinesin superfamily (KIFs) is a group of proteins that plays a critical role in transport and cytokinesis in eukaryotic cells. To date, 45 kinesin family members in 14 isoforms have been identified [8,9]. KIFs consist of a set of microtubule-dependent motor proteins that share a highly conserved motor structural domain. This domain provides binding sites for microtubules and facilitates protein and organelle transport in a microtubule-dependent manner. During mitosis and cytoplasmic division, KIFs play an essential role [10]. KIF15 is a member of the kinesin-12 subfamily and is also referred to as kinesin 12. It is involved in several cellular processes, including spindle assembly, plasma membrane transport, and cell division [11]. Studies have shown that KIF15 is overexpressed in tumor cells and regulates cell proliferation, apoptosis, and invasion/migration. Specifically, KIF15, in conjunction with KIF11, promotes the formation of the bipolar spindle, which suggests that KIF15 is crucial for cell proliferation [12,13]. KIF15 is currently found to be highly expressed in breast, liver, and pancreatic cancers. Silencing KIF15 inhibits the growth of these tumor cells and is a potential target for tumor prevention and treatment [14-16]. However, the relationship between KIF15 and NPC has not been investigated, and the molecular mechanisms by which KIF15 regulates the proliferation, migration, and invasion of NPC cells remain unknown. Therefore, this study examines the effects of KIF15 silencing on the proliferation, apoptosis, migration, and invasion of NPC cells.
The human NPC cell lines CNE-2, C666-1, SUNE-1, HONE-1, and 5-8F were acquired from the Shanghai Cell Institute at the Chinese Academy of Sciences. Gibco BRL provided trypsin, fetal bovine serum (FBS), and DMEM medium, while PBS reagents were purchased from Servicebio Technology Co. Dimethyl sulfoxide was sourced from MP Biomedicals, and isopropyl alcohol, methanol, and ethanol were acquired from Fuyu Fine Chemical Co. RIPA lysate was obtained from Beyotime Biotechnology Co., while the Cell Counting Kit-8 (CCK-8) was procured from Dojindo Chemical Research Institute. Lastly, the ECL chemiluminescence reagent was purchased from Thermo Scientific, Inc. siRNAs for p53 and negative control were purchased from Invitrogen. The siRNA sequences of p53 and negative control are list in Table 1.
The NPC cell lines (CNE-2, C666-1, 5-8F, SUNE-1, and HONE-1) were cultured in high-glucose DMEM medium containing 10% FBS at 37°C with 5% CO2 in a humidified environment. The cells were routinely sub-cultured every 2–3 days to maintain optimal cell growth conditions. Cells in the logarithmic growth phase were used for subsequent experiments and seeded onto culture plates accordingly.
To prepare for lentivirus-mediated knockdown of KIF15, 5 × 103 293T cells in logarithmic growth phase were inoculated into 6-well plates and cultured at 37°C with 5% CO2 saturation humidity until the cell density reached 50%–80%. Recombinant lentiviral vectors carrying the PLKO.1-KIF15-shRNA interference plasmid (experimental group) or PLKO.1-scramble-shRNA plasmid (negative control group) were transfected into the 293T cells with helper plasmids psPAX2 and pMD2.G using a lentiviral packaging instrument (The shRNA sequences of KIF15 and scramble are list in Table 2). The viral supernatant was collected after 24 and 48 h of transfection, centrifuged, and filtered through a 0.45 μm filter. The resulting solution was stored at –80°C for subsequent experiments. To enhance the lentiviral infection efficiency, polybrene was added to the NPC cell lines CNE-2 and 5-8F prior to lentiviral infection. After 48 h of infection, cells were screened with puromycin-containing medium. Stably transfected KIF15-shRNA/CNE-2, ScrshRNA/CNE-2, KIF15-shRNA/5-8F, and ScrshRNA/5-8F cell lines were filtered and amplified in culture for subsequent experiments.
After NPC cell treatment, trizol cell lysis solution was added to the collected cells for RNA extraction. The extracted RNA was quantified and transcribed into cDNA for use as a template in real-time fluorescence quantitative PCR. The reaction conditions consisted of 50 cycles at 95°C for 10 sec, 95°C for 5 sec and 60°C for 30 sec, followed by 1 h at 60°C, and 61 cycles at 65°C for 30 sec. Each group was set up with three replicate wells, and GAPDH served as the internal reference for mRNA expression analysis using the 2-△△Ct method. Primer sequences were designed using Primer Premier 5.0 software and synthesized by Invitrogen. The primer sequences are listed in Table 3.
Rabbit anti-α-tubulin antibody (#2144S), rabbit anti-cleaved-caspase 3 antibody (#9661S), rabbit anti-p-AKT antibody (#4060S) and rabbit anti-AKT antibody (#9272S), rabbit anti-P53 antibody (#2527S), rabbit anti-MMP2 antibody (#40994S) and rabbit anti-MMP9 antibody (#3852S) were purchased from Cell Signaling Technology. Rabbit anti-KIF15 antibody (ab272615) were purchased from Abcam. After completion of treatment of the NPC cell lines, the medium was discarded, and the total cell protein was extracted by adding RIPA lysate and quantitatively dispensed. The total protein was subjected to SDS-PAGE gel electrophoresis, and after transferring the membrane, it was blocked at room temperature for 2 h. The primary antibodies were added sequentially, diluted in proportion, and incubated overnight at 4°C, followed by addition of the corresponding secondary antibody dilutions the next day. The expression levels of the target protein were detected by chemiluminescence, and the developed bands were analyzed in grayscale using ImageJ software.
The CNE-2 and 5-8F cell lines in the logarithmic growth stage were seeded in 96-well plates at a density of 1 × 104 cells per well and incubated for 1, 3, 5, and 7 days, respectively. At the end of each incubation period, 10 μl of CCK-8 reagent was added to each well and incubated for an additional hour. The ELISA's wavelength was set at 450 nm, the absorbance value was corrected and zeroed using the cell culture solution, and the OD value of each well was measured.
After treatment, NPC cells were seeded in 6-well plates at a density of 2,000 cells/well, with 3 replicate wells set for each density. Next, 2 ml of DMEM medium containing 10% FBS was added to each well, and the plates were incubated in a 5% CO₂ incubator at 37°C for 7 days. Following incubation, the medium was discarded, and the cells were stained with 0.5% crystal violet staining solution prepared with 25% methanol. The stained cells were dried and photographed under a gel imaging system. Cell colonies consisting of more than 50 cells were counted using ImageJ software.
Apoptosis detection was performed using the Annexin V-FITC Apoptosis Detection Kit (BioVision) in combination with flow cytometry. This assay quantifies the externalization of cell membrane phosphatidylserine as a marker of apoptosis. A total of 1 × 105 cells were treated with serum-free medium for 24 h. Afterward, both floating and adherent cells were collected and washed with serum-containing medium. For each sample, a 1:50 dilution of Annexin V-FITC was prepared in binding buffer. Approximately 500 μl of the diluted solution was added to each tube, and the reaction was conducted at room temperature, protected from light, for 15 min. Following the reaction, the cells were analyzed using a BD FACSVerse flow cytometer and BD FASCuite software.
After completing the NPC cell treatment, they were seeded into the upper chamber of the Transwell at a density of 5 × 104 cells/well with a total of 200 μl of serum-free cell suspension per well. In the lower chamber, 600 μl of DMEM medium containing 10% FBS was added. The cells were incubated for 24 h, after which they were washed twice with PBS, and the liquid was aspirated. The Transwell chambers were fixed with 4% paraformaldehyde, followed by staining with 0.1% crystal violet staining solution for 15 min. After drying, the cells were inverted and photographed under a microscope, and the number of migrating cells in both groups was counted using ImageJ software.
Before the experiment, three equally spaced horizontal lines were marked at the bottom of a 6-well plate. After NPC cells were treated, they were seeded into the 6-well plates at a density of 3 × 105 cells/well, and 2 ml of DMEM medium containing 10% FBS was added to each well. The cells were then incubated until they reached confluence at the bottom of the plates. Using a sterile 200 μl pipette tip, scratches were made perpendicular to the black line mark on the back of the well plate with equal force. After scratching, the cells were rinsed gently with PBS and photographed under a microscope. Subsequently, the cells were incubated for 24 h, 36 h, and 48 h, and photographs were taken at each time point after removing the cells. Finally, PBS was aspirated, replaced with serum-free DMEM medium, and photographed under the microscope again.
Nude mice (BALB/c-nu, SPF grade) were obtained from Jinwei Biological Co. Stably transfected KIF15-shRNA/CNE-2 and ScrshRNA/CNE-2 cell lines were subcutaneously injected into the axilla of the right forelimb of each nude mouse using a disposable sterile syringe. The length and width of the tumors were measured and recorded on days 0, 2, 4, 6, 8, and 10 after injection. On day 10, the nude mice were imaged and observed for fluorescence using a live imager before being euthanized. The tumors were then removed, weighed, and the data were recorded.
Statistical parameters were calculated using ImageJ software, and statistical analysis was performed using SPSS 13.0 software. Data from each group were presented as mean ± SE, and one-way ANOVA was used to compare multiple groups. In all instances, differences were considered statistically significant when p < 0.05.
To elucidate the differences in expression of KIF15 between normal nasopharyngeal epithelial cells and nasopharyngeal cancer cell lines, we isolated mRNA and protein from normal nasopharyngeal epithelial cells (NP69) and nasopharyngeal cancer cell lines (C666-1, CNE-2, SUNE-1, HONE-1, and 5-8F) and examined KIF15 expression. Our results demonstrated that both mRNA and protein levels of KIF15 were higher in NPC cell lines compared to NP69 cells, with the most significant differences observed in CNE-2 and 5-8F (Fig. 1). These findings suggest that KIF15 may play a role in nasopharyngeal carcinogenesis and progression. In the following experiments, we will utilize CNE-2 and 5-8F cell lines to investigate the role of KIF15 in NPC.
To investigate the role of KIF15 in NPC, we first generated stable cell lines with KIF15 knockdown using CNE-2 and 5-8F cell lines, which were named KIF15-shRNA/CNE-2, ScrshRNA/CNE-2, KIF15-shRNA/5-8F, and ScrshRNA/5-8F, respectively. To confirm successful knockdown of KIF15, we performed real-time PCR and Western blotting to examine the mRNA and protein expression levels in these cell lines. As depicted in Fig. 2A–E, KIF15 expression was significantly reduced in the stably transfected cell lines compared to the control group, indicating successful construction of the stable cell lines. We then evaluated cell proliferation in the cell lines with KIF15 knockdown by generating cell growth curves, which revealed that the growth of the KIF15-shRNA group was significantly inhibited compared to the normal controls (Fig. 2F, G). Additionally, clonogenic assays showed that silencing KIF15 inhibited the growth of NPC cells (Fig. 2H–K).
To clarify how KIF15 regulates NPC cell proliferation, we conducted flow cytometry experiments on both control and stably transfected KIF15-shRNA NPC cell lines. The results indicate that the proportion of apoptotic cells was significantly higher in the KIF15-shRNA group compared to the control group (Fig. 3A–D). Furthermore, we examined the mRNA expression of the apoptosis-related genes Bax and Bcl-2, and our data showed that silencing KIF15 significantly upregulated the mRNA expression of Bax, while suppressing the level of Bcl-2, leading to a significant increase in the Bax/Bcl-2 ratio (Fig. 3E–J). Additionally, we observed a significant increase in the level of cleaved-caspase3 protein in the cells of the KIF15-silenced group (Fig. 3K, L). Taken together, these findings suggest that silencing KIF15 induces apoptosis in NPC cells to a substantial degree.
We proceeded to investigate the impact of KIF15 on the migration and invasion of NPC cells. The Transwell assay results demonstrated a reduction in the number of invasive NPC cells upon KIF15 silencing, as compared to normal cells (Fig. 4A–D). In the scratch assay, NPC cells silenced with KIF15 exhibited a low scratch closure rate (Fig. 4E–H). These results indicated that KIF15 knockdown significantly suppressed the migration and invasion ability of NPC cells.
To further investigate the specific molecular mechanisms by which KIF15 regulates the proliferation, migration, and invasion of NPC cells, we examined the effects of KIF15 silencing on AKT and P53 signaling pathways using Western blotting. Fig. 5 shows that silencing KIF15 in both CNE-2 and 5-8F NPC cells inhibited AKT phosphorylation and activated P53 protein expression. Furthermore, silencing KIF15 also suppressed MMP2 and MMP9 mRNA and protein expression levels. These findings suggest that KIF15 may modulate NPC cell growth through AKT- and P53-dependent signaling pathways.
To investigate the involvement of p53-dependent signaling pathways in the regulation of KIF15-induced apoptosis, we synthesized siRNA interference sequences targeting p53 and confirmed the successful inhibition of p53 in NPC cells (Fig. 6A, B). Subsequently, we individually suppressed p53 expression in KIF15-shRNA-CNE-2/5-8F cell lines to observe its effect on apoptosis in NPC cells. The results demonstrated a significant decrease in the proportion of apoptotic cells in the KIF15-shRNA-sip53 group compared to the KIF15-shRNA group (Fig. 6C–F). Further analysis revealed a significant decrease in Bax mRNA expression and a significant increase in Bcl-2 mRNA levels in the cells of the KIF15-shRNA-sip53 group, leading to a notable reduction in the Bax/Bcl-2 ratio and inhibition of caspase 3 protein expression (Fig. 6G–N). These findings suggest that silencing KIF15 induces apoptosis in NPC cells by activating the p53 signaling pathway and modulating the expression of bax/bcl-2 and caspase 3.
To further validate the effect of silencing KIF15 on the growth of NPC cells in vivo, we established a nude mouse transplantation tumor model and subcutaneously injected KIF15-shRNA/CNE-2 and ScrshRNA/CNE-2 stably transfected cell lines into nude mice to observe tumor growth. The results demonstrated that the KIF15-shRNA group exhibited slower tumor growth and smaller tumor volume and weight than the control group (Fig. 7A–D). In vivo imaging data also revealed significantly weaker tumor signals in the KIF15-shRNA group compared to the control group (Fig. 7E, F). These findings provide further evidence that silencing KIF15 can inhibit the growth of NPC cells in vivo.
NPC is the most common head and neck tumor in Southeast Asia and South China, and its incidence has been steadily increasing. Although radiotherapy is an effective treatment, NPC is often detected at an advanced stage due to its hidden location and lack of clear early symptoms. This leads to poor prognosis and significant adverse effects [5]. Additionally, distant metastases and local recurrence remain major causes of treatment failure, and targeted therapy has emerged as a promising approach with high efficacy and low toxicity [6,17,18]. Given the intricate and complex pathogenesis of NPC, it is critical to deepen our understanding of its molecular mechanisms. The identification of therapeutic markers and targets relevant to NPC diagnosis and treatment is expected to generate novel treatment options. In this study, we report for the first time that KIF15 mRNA and protein expression were significantly upregulated in NPC cell lines compared to normal nasopharyngeal epithelial cells (NP69). These findings suggest that KIF15 may be involved in the development and progression of NPC.
In recent years, a growing body of evidence has demonstrated that aberrant expression or dysfunction of kinesins can contribute to disease development, including tumorigenesis [19]. Specifically, several kinesin family members, including KIF14, KIF2C, KIF18A, and KIF15, have been implicated in cancer progression [14,19]. KIF14 has been shown to promote AKT phosphorylation, resulting in resistance to therapeutic agents in breast cancer patients [20]. In addition, KIF2C has been independently associated with poor prognosis in patients with gastric and colorectal cancers and glioma [21]. Similarly, high expression of KIF18A has been linked to distant metastasis and poor survival in colon and breast cancer patients [22]. KIF15, which is closely associated with mitosis, sperm production, cell proliferation, and differentiation, has been implicated in various biological processes [23]. For instance, studies have shown that KIF15 affects axon growth during neuronal development and regeneration in zebrafish [24]. Furthermore, KIF15 has been shown to play a role in the development of auditory and vestibular organs, with one study suggesting that KIF15 silencing inhibits normal vestibular organ formation in zebrafish, leading to behavioral dysfunction [25]. In the context of tumorigenesis, KIF15 has been found to be highly expressed in most tumor cells, including breast cancer, prostate cancer, and gallbladder cancer [14,16]. Studies have shown that KIF15 overexpression is associated with enzalutamide resistance in prostate cancer cells, and that silencing KIF15 inhibits the growth of gallbladder cancer cells [26]. Despite these findings, the role of KIF15 in the development of NPC has not been reported. Therefore, in this study, we used lentivirus to construct a KIF15 gene-silencing NPC cell model and found that silencing KIF15 inhibited the proliferation, migration, and invasion of NPC CNE-2 and 5-8F cells. Furthermore, transplantation tumor experiments in nude mice showed that silencing KIF15 could inhibit the growth of NPC in vivo. These findings suggest that KIF15 is a potential new target for NPC treatment and further investigation is warranted.
Thus far, the molecular mechanisms underlying KIF15's regulation of the proliferation, migration, and invasion of NPC cells remain to be fully elucidated. As a critical tumor suppressor, p53 plays a pivotal role in regulating cell cycle progression, cell growth, and apoptosis in cancer cells [27]. One of p53's major tumor suppressive functions is its ability to induce apoptosis. As a nuclear transcription factor, p53 regulates a plethora of genes involved in apoptosis. Notably, p53 has been reported to translocate to the mitochondria where it interacts directly with BCL-2 family proteins to mediate the mitochondrial apoptotic pathway (also known as the intrinsic pathway) [28]. The BCL-2 family comprises both pro- and anti-apoptotic members that regulate mitochondrial outer membrane permeability and thereby activate the caspase cascade and induce apoptosis in cancer cells [29]. The p53-dependent mitochondrial apoptotic pathway is also implicated in NPC pathogenesis [30,31]. Our group's previous study demonstrated that (-)-epigallocatechin-3-gallate (EGCG), the main monomeric component of green tea polyphenols, induces apoptosis in NPC cells by activating the p53-dependent mitochondrial apoptotic pathway [32]. In the current study, we observed that silencing KIF15 increased the expression of p53, which in turn upregulated the expression of apoptotic genes such as Bax, Bcl-2, and caspase3 in the mitochondrial apoptotic pathway. These results suggest that KIF15 may regulate the p53-dependent signaling pathway to induce apoptosis and inhibit the proliferation of NPC cells.
However, the specific molecular mechanisms by which KIF15 regulates p53 are not known. Studies have shown that multiple mechanisms such as transcriptional regulation, subcellular localization, post-translational modifications, and protein stability can regulate the expression and activity of p53, which strictly control the level of p53 in normal cells but can rapidly activate p53 and clear damaged cells under stressful conditions [33]. The stability of p53 protein is important for the maintenance of cellular self-homeostasis. In normal cells without stress, p53 activity is maintained at low levels through a combination of p53 degradation and transcriptional repression, which is mainly mediated by Mdm2 (murine double minute clone2) [34]. Mdm2 regulates p53 activity through ubiquitination-dependent p53 degradation and inhibition of p53 transcriptional activity [34,35]. In addition, the Mdm2 gene is in turn activated by p53 transcription, constituting a feedback regulatory loop [36]. When cells are under stress such as DNA damage, there are various mechanisms that can block the degradation of p53 and promote the rapid increase of p53 protein to prevent abnormal growth or malignant transformation, such as post-translational modification of p53 and Mdm2, blockage of p53 ubiquitination pathway, disruption of p53-Mdm2 interaction and other non-Mdm2-dependent mechanisms. In vitro studies have shown that Akt phosphorylation of the Ser166 and/or Ser186 sites of Mdm2 promotes Mdm2 translocation into the nucleus [37,38] and reduces the transcriptional activity of p53-dependent genes [37]. In the present study, we found that silencing KIF15 could inhibit Akt phosphorylation. Therefore, we can speculate that silencing KIF15 can promote apoptosis in NPC by inhibiting Akt phosphorylation and thus reducing the phosphorylation of Mdm2 and decreasing the degradation of p53, ultimately exerting anti-NPC effects.
Invasion and metastasis are hallmarks of cancer and refer to the detachment of malignant cells from the primary site, subsequent invasion into adjacent normal tissues, and colonization at distant sites, where secondary tumors form [39]. Distant metastasis is a major cause of treatment failure in NPC [5]. The aberrant activation of the PI3K/AKT signaling pathway has been shown to play a crucial role in the migration and invasion of cancer cells. Specifically, activation of p-PI3K leads to the activation of AKT, which in turn activates downstream molecules, such as MMP2 and MMP9, promoting the migration and invasion of tumor cells [40,41]. In laryngeal squamous cell carcinoma, activation of the PI3K/Akt-NF-κB pathway upregulates MMP2/3 expression, enhancing cell proliferation and migration [42]. Similarly, the PI3K/AKT pathway has been implicated in the migration and invasion of NPC cells [43]. For instance, FOXD3 has been found to inhibit cell proliferation, migration, and invasion in NPC by regulating the PI3K-Akt pathway [44]. Additionally, YBX3 has been reported to mediate metastasis in NPC through PI3K/AKT signaling [45]. Furthermore, green tea polyphenol EGCG was shown to inhibit NPC cell migration by suppressing MMP2 expression [46]. Collectively, these findings highlight the importance of the PI3K/AKT/MMP signaling pathway in regulating the migration and invasion of NPC cells. Our study demonstrated that silencing KIF15 inhibited AKT phosphorylation levels and MMP2 and MMP9 protein expression, thereby inhibiting the proliferation, migration, and invasion of NPC cells through the regulation of AKT and p53-dependent signaling pathways (Fig. 8). However, the specific molecular mechanisms by which KIF15 regulates tumor growth remain unclear. For example, previous studies have shown that silencing KIF15 can inhibit tumorigenesis in non-small cell lung cancer by affecting Raf/MEK/ERK signaling [47], while KIF15 upregulation promotes smooth muscle sarcoma cell growth by promoting USP15-mediated DEK deubiquitination [48]. Therefore, further investigations are needed to determine whether these signaling pathways are involved in the regulatory role of KIF15 in NPC cells.
In this study, we have demonstrated for the first time that silencing KIF15 significantly inhibits the proliferation, migration, and invasion of NPC cells. Additionally, we found that inhibition of KIF15 can promote the growth of NPC in vivo, and its mechanism of action may be related to AKT and P53-dependent signaling pathways. However, our investigation of the role of KIF15 in NPC is still relatively limited, and further in-depth research is needed to elucidate its related downstream molecular mechanisms. Our work provides a preliminary experimental basis for establishing KIF15 as a novel therapeutic target for preventing and treating NPC.
REFERENCES
1. Tang SQ, Mao YP, Xu C, Guo R, Li WF, Tang LL, Sun Y, Ma J. 2020; The evolution of the nasopharyngeal carcinoma staging system over a 10-year period: implications for future revisions. Chin Med J (Engl). 133:2044–2053. DOI: 10.1097/CM9.0000000000000978. PMID: 32810045. PMCID: PMC7478675. PMID: 45b20d7da1104cda981fe16dd2ef952e.


2. Hung CC, Tu MY, Chien TW, Lin CY, Chow JC, Chou W. 2023; The model of descriptive, diagnostic, predictive, and prescriptive analytics on 100 top-cited articles of nasopharyngeal carcinoma from 2013 to 2022: bibliometric analysis. Medicine (Baltimore). 102:e32824. DOI: 10.1097/MD.0000000000032824. PMID: 36820592. PMCID: PMC9907932.


3. Pontoriero F, Silverman AM, Pascasio JM, Bajaj R. 2020; Nonkeratinizing nasopharyngeal carcinoma, undifferentiated type with trisomy 2: a case report and short review of cytogenetic and molecular literature. Pediatr Dev Pathol. 23:448–452. DOI: 10.1177/1093526620945861. PMID: 32755442.


4. Lam WKJ, Chan JYK. 2018; Recent advances in the management of nasopharyngeal carcinoma. F1000Res. 7:F1000 Faculty Rev-1829. DOI: 10.12688/f1000research.15066.1. PMID: 30519454. PMCID: PMC6249636. PMID: 8086e0a2c60c441698858e8bc2691c39.


5. Chen YP, Chan ATC, Le QT, Blanchard P, Sun Y, Ma J. 2019; Nasopharyngeal carcinoma. Lancet. 394:64–80. DOI: 10.1016/S0140-6736(19)30956-0. PMID: 31178151.


6. Lee HM, Okuda KS, González FE, Patel V. 2019; Current perspectives on nasopharyngeal carcinoma. Adv Exp Med Biol. 1164:11–34. DOI: 10.1007/978-3-030-22254-3_2. PMID: 31576537.


7. Lee YT, Tan YJ, Oon CE. 2018; Molecular targeted therapy: treating cancer with specificity. Eur J Pharmacol. 834:188–196. DOI: 10.1016/j.ejphar.2018.07.034. PMID: 30031797.


8. Hirokawa N, Noda Y, Tanaka Y, Niwa S. 2009; Kinesin superfamily motor proteins and intracellular transport. Nat Rev Mol Cell Biol. 10:682–696. DOI: 10.1038/nrm2774. PMID: 19773780.


9. Huo D, Yang H, Huang JD, Cai JP, Cui J. 2021; Roles of kinesin superfamily proteins in colorectal cancer carcinogenesis (Review). Oncol Rep. 46:121. DOI: 10.3892/or.2021.8072. PMID: 33955521.


10. Niwa S. 2015; Kinesin superfamily proteins and the regulation of microtubule dynamics in morphogenesis. Anat Sci Int. 90:1–6. DOI: 10.1007/s12565-014-0259-5. PMID: 25347970.


11. Wang Z, Chen M, Fang X, Hong H, Yao Y, Huang H. 2021; KIF15 is involved in development and progression of Burkitt lymphoma. Cancer Cell Int. 21:261. DOI: 10.1186/s12935-021-01967-z. PMID: 33985517. PMCID: PMC8117549. PMID: dbca50a309e04f1389cba3241fd2e9fd.


12. Sun RF, He N, Zhang GY, Yu ZY, Li LS, Ma ZJ, Jiao ZY. 2023; Combined inhibition of KIF11 and KIF15 as an effective therapeutic strategy for gastric cancer. Curr Cancer Drug Targets. 23:293–306. DOI: 10.2174/1568009622666220616122846. PMID: 35713129.
13. Klejnot M, Falnikar A, Ulaganathan V, Cross RA, Baas PW, Kozielski F. 2014; The crystal structure and biochemical characterization of Kif15: a bifunctional molecular motor involved in bipolar spindle formation and neuronal development. Acta Crystallogr D Biol Crystallogr. 70(Pt 1):123–133. DOI: 10.1107/S1399004713028721. PMID: 24419385. PMCID: PMC3919264.


14. Gao X, Zhu L, Lu X, Wang Y, Li R, Jiang G. 2020; KIF15 contributes to cell proliferation and migration in breast cancer. Hum Cell. 33:1218–1228. DOI: 10.1007/s13577-020-00392-0. PMID: 32578050.


15. Sun YF, Wu HL, Shi RF, Chen L, Meng C. 2020; KIF15 promotes proliferation and growth of hepatocellular carcinoma. Anal Cell Pathol (Amst). 2020:6403012. DOI: 10.1155/2020/6403012. PMID: 32318326. PMCID: PMC7157793. PMID: 56c6e1dbeba04ef68252ee05e62e5a77.


16. Gao L, Zhang W, Zhang J, Liu J, Sun F, Liu H, Hu J, Wang X, Wang X, Su P, Chen S, Qu S, Shi B, Xiong X, Chen W, Dong X, Han B. 2021; KIF15-mediated stabilization of AR and AR-V7 contributes to enzalutamide resistance in prostate cancer. Cancer Res. 81:1026–1039. DOI: 10.1158/0008-5472.CAN-20-1965. PMID: 33277366.


17. Perri F, Della Vittoria Scarpati G, Caponigro F, Ionna F, Longo F, Buonopane S, Muto P, Di Marzo M, Pisconti S, Solla R. 2019; Management of recurrent nasopharyngeal carcinoma: current perspectives. Onco Targets Ther. 12:1583–1591. DOI: 10.2147/OTT.S188148. PMID: 30881013. PMCID: PMC6396653.
18. Vasudevan HN, Yom SS. 2021; Nasopharyngeal carcinoma and its association with Epstein-Barr virus. Hematol Oncol Clin North Am. 35:963–971. DOI: 10.1016/j.hoc.2021.05.007. PMID: 34187713.


19. Yu Y, Feng YM. 2010; The role of kinesin family proteins in tumorigenesis and progression: potential biomarkers and molecular targets for cancer therapy. Cancer. 116:5150–5160. DOI: 10.1002/cncr.25461. PMID: 20661912.


20. Singel SM, Cornelius C, Zaganjor E, Batten K, Sarode VR, Buckley DL, Peng Y, John GB, Li HC, Sadeghi N, Wright WE, Lum L, Corson TW, Shay JW. 2014; KIF14 promotes AKT phosphorylation and contributes to chemoresistance in triple-negative breast cancer. Neoplasia. 16:247–256.e2. DOI: 10.1016/j.neo.2014.03.008. PMID: 24784001. PMCID: PMC4094827. PMID: 6c64beef889741d38f469bb388b70ed5.


21. Shimo A, Tanikawa C, Nishidate T, Lin ML, Matsuda K, Park JH, Ueki T, Ohta T, Hirata K, Fukuda M, Nakamura Y, Katagiri T. 2008; Involvement of kinesin family member 2C/mitotic centromere-associated kinesin overexpression in mammary carcinogenesis. Cancer Sci. 99:62–70. DOI: 10.1111/j.1349-7006.2007.00635.x. PMID: 17944972.


22. Nagahara M, Nishida N, Iwatsuki M, Ishimaru S, Mimori K, Tanaka F, Nakagawa T, Sato T, Sugihara K, Hoon DS, Mori M. 2011; Kinesin 18A expression: clinical relevance to colorectal cancer progression. Int J Cancer. 129:2543–2552. DOI: 10.1002/ijc.25916. PMID: 21213216.


23. Ding L, Li B, Yu X, Li Z, Li X, Dang S, Lv Q, Wei J, Sun H, Chen H, Liu M, Li G. 2020; KIF15 facilitates gastric cancer via enhancing proliferation, inhibiting apoptosis, and predict poor prognosis. Cancer Cell Int. 20:125. DOI: 10.1186/s12935-020-01199-7. PMID: 32322172. PMCID: PMC7160940. PMID: 477878391acb4aaebcaecb0a53c96a89.


24. Zheng S, Tang D, Wang X, Liu C, Zuo N, Yan R, Wu C, Ma J, Wang C, Xu H, He Y, Liu D, Liu S. 2022; Kif15 is required in the development of auditory system using zebrafish as a model. Front Mol Neurosci. 15:844568. DOI: 10.3389/fnmol.2022.844568. PMID: 35370541. PMCID: PMC8971910. PMID: 459e99e04b934a66bd00f63a2bbe352f.


25. Xu M, Liu D, Dong Z, Wang X, Wang X, Liu Y, Baas PW, Liu M. 2014; Kinesin-12 influences axonal growth during zebrafish neural development. Cytoskeleton (Hoboken). 71:555–563. DOI: 10.1002/cm.21193. PMID: 25250533. PMCID: PMC4236235.


26. Wang J, Wang D, Fei Z, Feng D, Zhang B, Gao P, Hu G, Li W, Huang X, Chen D, Ding X, Wu W. 2021; KIF15 knockdown suppresses gallbladder cancer development. Eur J Cell Biol. 100:151182. DOI: 10.1016/j.ejcb.2021.151182. PMID: 34781077.


27. Wade M, Li YC, Wahl GM. 2013; MDM2, MDMX and p53 in oncogenesis and cancer therapy. Nat Rev Cancer. 13:83–96. DOI: 10.1038/nrc3430. PMID: 23303139. PMCID: PMC4161369.


28. Blagih J, Buck MD, Vousden KH. 2020; p53, cancer and the immune response. J Cell Sci. 133:jcs237453. DOI: 10.1242/jcs.237453. PMID: 32144194.


29. Kale J, Osterlund EJ, Andrews DW. 2018; BCL-2 family proteins: changing partners in the dance towards death. Cell Death Differ. 25:65–80. DOI: 10.1038/cdd.2017.186. PMID: 29149100. PMCID: PMC5729540.


30. Weng C, Chen Y, Wu Y, Liu X, Mao H, Fang X, Li B, Wang L, Guan M, Liu G, Lu L, Yuan Y. 2019; Silencing UBE4B induces nasopharyngeal carcinoma apoptosis through the activation of caspase3 and p53. Onco Targets Ther. 12:2553–2561. DOI: 10.2147/OTT.S196132. PMID: 31040698. PMCID: PMC6459139.
31. Wang Z, Liao K, Zuo W, Liu X, Qiu Z, Gong Z, Liu C, Zeng Q, Qian Y, Jiang L, Bu Y, Hong S, Hu G. 2017; Depletion of NFBD1/MDC1 induces apoptosis in nasopharyngeal carcinoma cells through the p53-ROS-mitochondrial pathway. Oncol Res. 25:123–136. DOI: 10.3727/096504016X14732772150226. PMID: 28081741. PMCID: PMC7840771.


32. Jiang S, Huang C, Zheng G, Yi W, Wu B, Tang J, Liu X, Huang B, Wu D, Yan T, Li M, Wan C, Cai Y. 2022; EGCG inhibits proliferation and induces apoptosis through downregulation of SIRT1 in nasopharyngeal carcinoma cells. Front Nutr. 9:851972. DOI: 10.3389/fnut.2022.851972. PMID: 35548580. PMCID: PMC9084317. PMID: 6b11df6f0e6347b89f84c58a0682b4e7.


33. Hernández Borrero LJ, El-Deiry WS. 2021; Tumor suppressor p53: biology, signaling pathways, and therapeutic targeting. Biochim Biophys Acta Rev Cancer. 1876:188556. DOI: 10.1016/j.bbcan.2021.188556. PMID: 33932560. PMCID: PMC8730328.


34. Junttila MR, Evan GI. 2009; p53--a Jack of all trades but master of none. Nat Rev Cancer. 9:821–829. DOI: 10.1038/nrc2728. PMID: 19776747.
35. Moreira J, Almeida J, Saraiva L, Cidade H, Pinto M. 2021; Chalcones as promising antitumor agents by targeting the p53 pathway: an overview and new insights in drug-likeness. Molecules. 26:3737. DOI: 10.3390/molecules26123737. PMID: 34205272. PMCID: PMC8233907. PMID: 6b9d17e5acf149adb7d23cb2cbf99477.


36. Kruse JP, Gu W. 2009; Modes of p53 regulation. Cell. 137:609–622. DOI: 10.1016/j.cell.2009.04.050. PMID: 19450511. PMCID: PMC3737742.


37. Mayo LD, Donner DB. 2001; A phosphatidylinositol 3-kinase/Akt pathway promotes translocation of Mdm2 from the cytoplasm to the nucleus. Proc Natl Acad Sci U S A. 98:11598–11603. DOI: 10.1073/pnas.181181198. PMID: 11504915. PMCID: PMC58775.


38. Zhou BP, Liao Y, Xia W, Zou Y, Spohn B, Hung MC. 2001; HER-2/neu induces p53 ubiquitination via Akt-mediated MDM2 phosphorylation. Nat Cell Biol. 3:973–982. Erratum in: Nat Cell Biol. 2002; 4:736. DOI: 10.1038/ncb1101-973. PMID: 11715018.


39. Zanotelli MR, Zhang J, Reinhart-King CA. 2021; Mechanoresponsive metabolism in cancer cell migration and metastasis. Cell Metab. 33:1307–1321. DOI: 10.1016/j.cmet.2021.04.002. PMID: 33915111. PMCID: PMC9015673.


40. Liang W, Shi J, Xia H, Wei X. 2021; A novel ruthenium-fluvastatin complex downregulates SNCG expression to modulate breast carcinoma cell proliferation and apoptosis via activating the PI3K/Akt/mTOR/VEGF/MMP9 pathway. Oxid Med Cell Longev. 2021:5537737. DOI: 10.1155/2021/5537737. PMID: 34221232. PMCID: PMC8221895.


41. Zhu W, Wu X, Yang B, Yao X, Cui X, Xu P, Chen X. 2019; miR-188-5p regulates proliferation and invasion via PI3K/Akt/MMP-2/9 signaling in keloids. Acta Biochim Biophys Sin (Shanghai). 51:185–196. Erratum in: Acta Biochim Biophys Sin (Shanghai). 2019;51:980. DOI: 10.1093/abbs/gmy165. PMID: 30668826.


42. Zhu Y, Yan L, Zhu W, Song X, Yang G, Wang S. 2019; MMP2/3 promote the growth and migration of laryngeal squamous cell carcinoma via PI3K/Akt-NF-κB-mediated epithelial-mesenchymal transformation. J Cell Physiol. 234:15847–15855. DOI: 10.1002/jcp.28242. PMID: 30714134.


43. Li HL, Deng NH, He XS, Li YH. 2022; Small biomarkers with massive impacts: PI3K/AKT/mTOR signalling and microRNA crosstalk regulate nasopharyngeal carcinoma. Biomark Res. 10:52. DOI: 10.1186/s40364-022-00397-x. PMID: 35883139. PMCID: PMC9327212. PMID: 5e5616c222d647368dacc772e1aca5c1.


44. Xie X, Xiong G, Chen W, Fu H, Li M, Cui X. 2020; FOXD3 inhibits cell proliferation, migration, and invasion in nasopharyngeal carcinoma through regulation of the PI3K-Akt pathway. Biochem Cell Biol. 98:653–660. DOI: 10.1139/bcb-2020-0011. PMID: 32459973.


45. Fan X, Xie X, Yang M, Wang Y, Wu H, Deng T, Weng X, Wen W, Nie G. 2021; YBX3 mediates the metastasis of nasopharyngeal carcinoma via PI3K/AKT signaling. Front Oncol. 11:617621. DOI: 10.3389/fonc.2021.617621. PMID: 33816248. PMCID: PMC8010247. PMID: 358fd794b7514d329b00141c1817b77b.


46. Ho HC, Huang CC, Lu YT, Yeh CM, Ho YT, Yang SF, Hsin CH, Lin CW. 2019; Epigallocatechin-3-gallate inhibits migration of human nasopharyngeal carcinoma cells by repressing MMP-2 expression. J Cell Physiol. 234:20915–20924. DOI: 10.1002/jcp.28696. PMID: 31012106.


47. Luo Y, Zhang B, Xu L, Li M, Wu J, Zhou Y, Li Y. 2022; Downregulation of KIF15 inhibits the tumorigenesis of non-small-cell lung cancer via inactivating Raf/MEK/ERK signaling. Histol Histopathol. 37:269–285. DOI: 10.14670/HH-18-408. PMID: 34908156.
48. Ge W, Chen Y, Guo Y, Zhao D, Mu L, Zhang K, Zhuo W. 2021; KIF15 upregulation promotes leiomyosarcoma cell growth via promoting USP15-mediated DEK deubiquitylation. Biochem Biophys Res Commun. 570:117–124. DOI: 10.1016/j.bbrc.2021.07.042. PMID: 34280614.


Fig. 1
Expression of KIF15 in normal nasopharyngeal epithelial cells and NPC cell lines.
The mRNA (A) and protein (B) expression of KIF15 were detected in different cell lines (NP69, C666-1, CNE-2, SUNE-1, HONE-1, and 5-8F). Values are presented as mean ± SD. KIF15, kinesin superfamily member 15; NPC, nasopharyngeal carcinoma. *p < 0.05 vs. the NP69 group, n = 5.
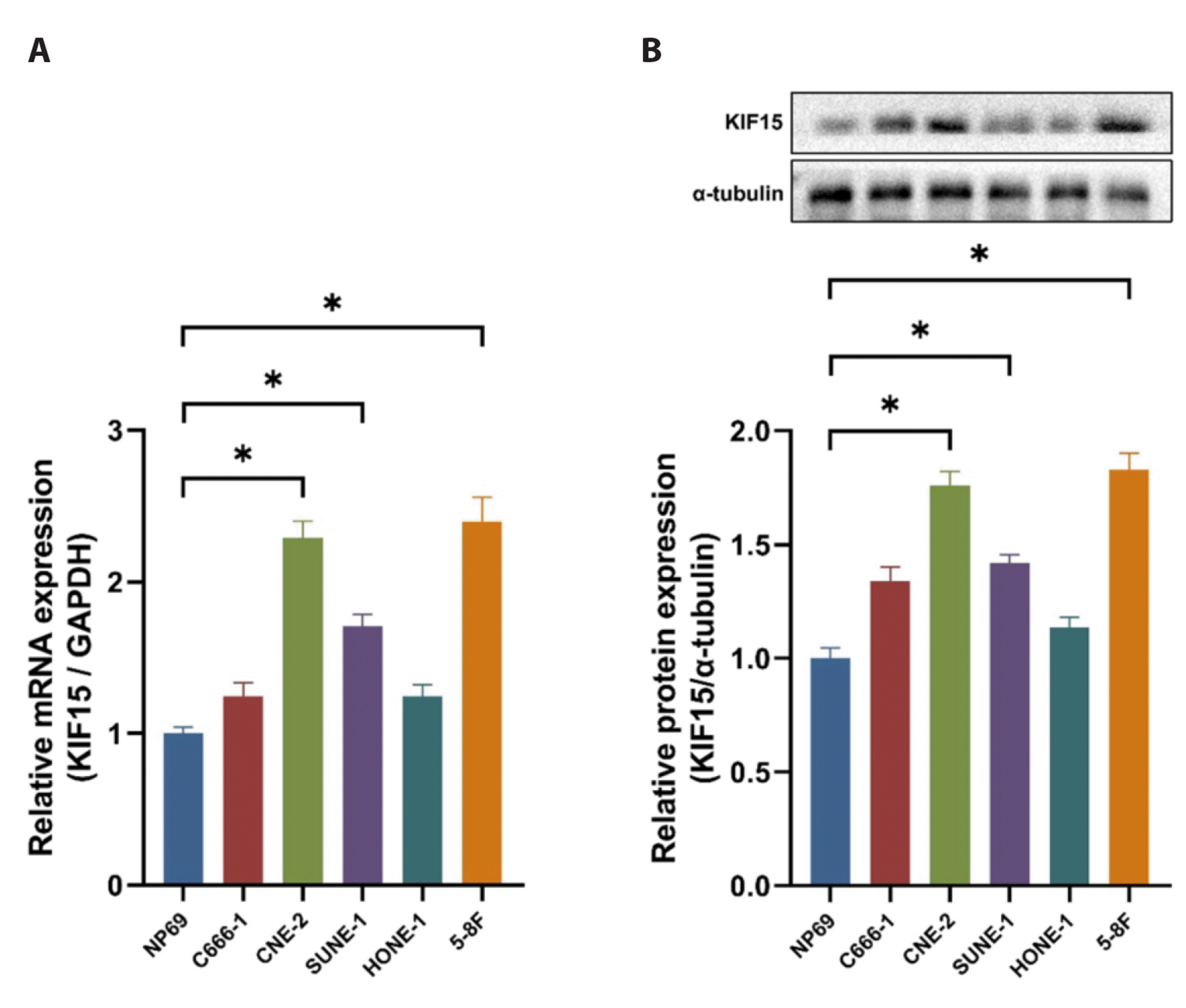
Fig. 2
Effect of KIF15 knockdown on the proliferation of NPC cell lines.
The mRNA (A) and protein expression (C, D) of KIF15 were detected in KIF15-shRNA/CNE-2 and ScrshRNA/CNE-2 cell lines. The mRNA (B) and protein expression (C, E) of KIF15 were detected in KIF15-shRNA/5-8F and ScrshRNA/5-8F cell lines. The cell viability (F) and relative colony formation (stained with 0.5% crystal violet staining solution) (H, I) was detected in KIF15-shRNA/CNE-2 and ScrshRNA/CNE-2 cell lines. The cell viability (G) and relative colony formation (stained with 0.5% crystal violet staining solution) (J, K) was detected in KIF15-shRNA/5-8F and ScrshRNA/5-8F cell lines. Values are presented as mean ± SD. KIF15, kinesin superfamily member 15; NPC, nasopharyngeal carcinoma. **p < 0.01 vs. the shcontrol group, n = 5.
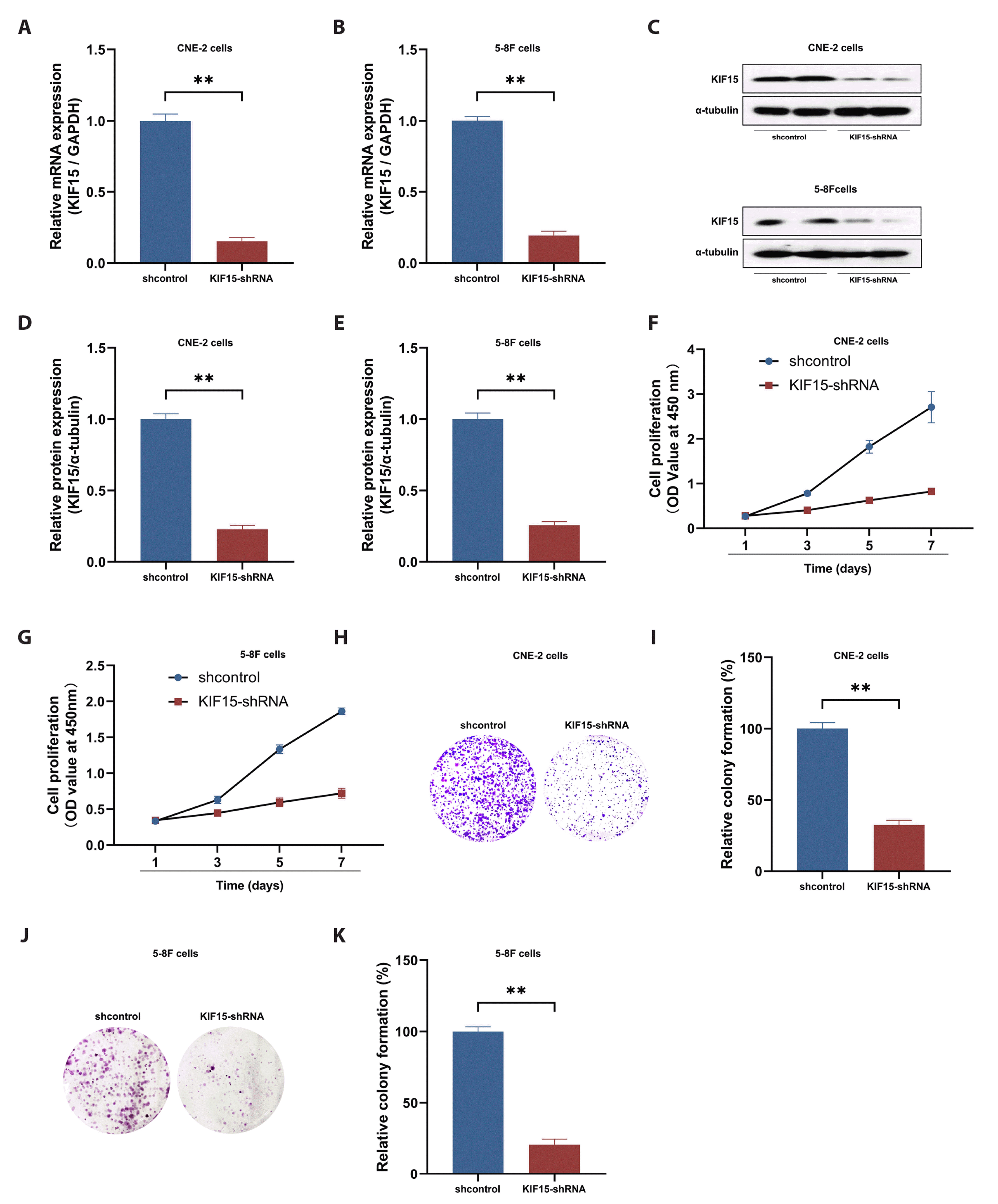
Fig. 3
KIF15 knockdown induces apoptosis in NPC cells.
(A, B) The flow cytometric analysis was detected in KIF15-shRNA/CNE-2 and ScrshRNA/CNE-2 cell lines. (C, D) The flow cytometric analysis was detected in KIF15-shRNA/5-8F and ScrshRNA/5-8F cell lines. The mRNA expression of Bax (E), Bcl-2 (G), and Bax/Bcl-2 (I) were detected in KIF15-shRNA/CNE-2 and ScrshRNA/CNE-2 cell lines. The mRNA expression of Bax (F), Bcl-2 (H), and Bax/Bcl-2 (J) were detected in KIF15-shRNA/5-8F and ScrshRNA/5-8F cell lines. The protein expression of cleaved-caspase 3 was detected in CNE-2 (K) and 5-8F (L) cells. Values are presented as mean ± SD. KIF15, kinesin superfamily member 15; NPC, nasopharyngeal carcinoma; ns, no significance. *p < 0.05 and **p < 0.01 vs. the shcontrol group, n = 5.
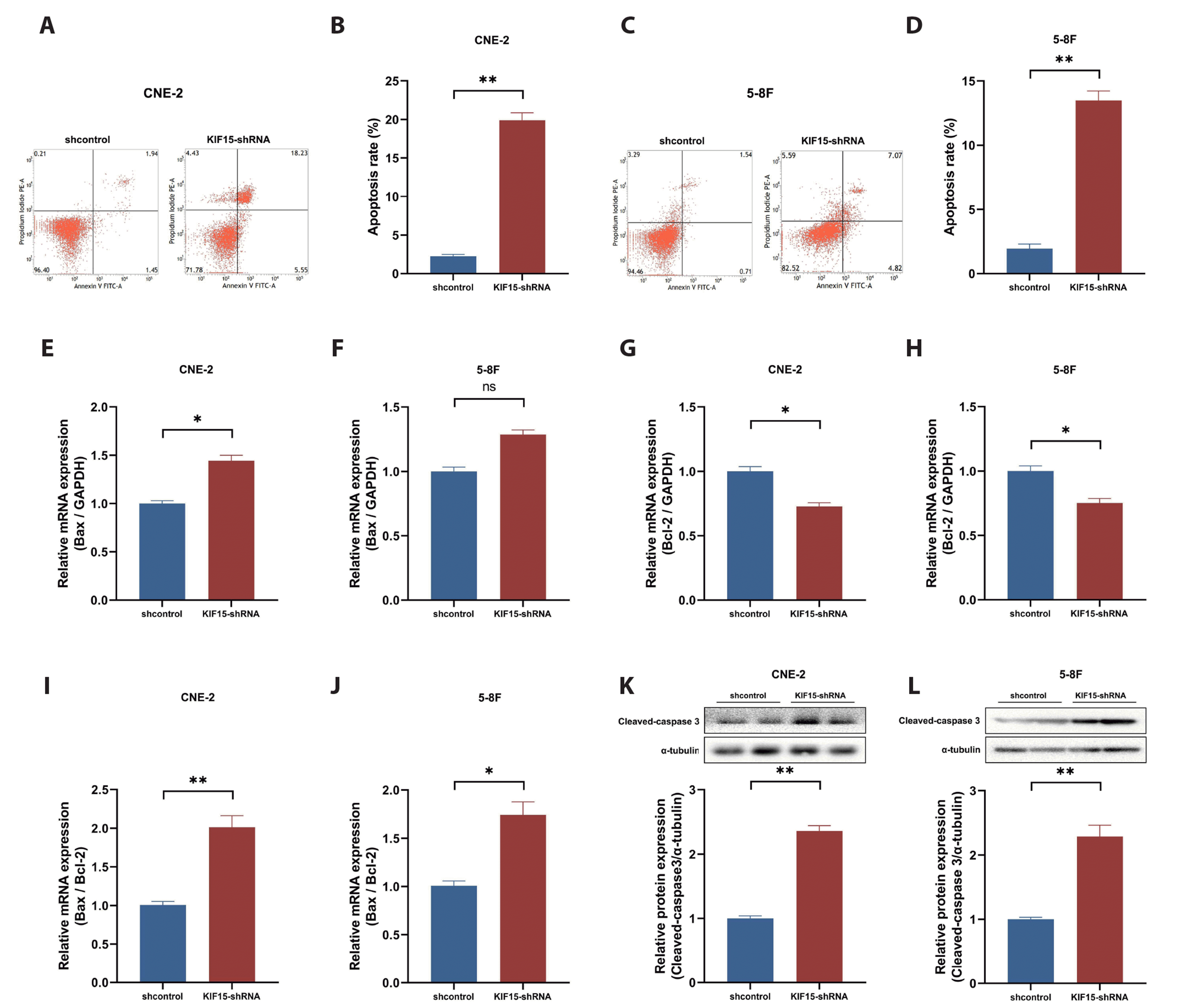
Fig. 4
Effect of KIF15 knockdown on migration and invasion of NPC cell lines.
Representative images of transwell-migration assays of CNE-2 (A) and 5-8F (B) cells and (C, D) quantification of cell migration expressed by cell counting. Representative images of scratch assay performed in CNE-2 (E) and 5-8F (F) cells. (G, H) The mobility (%) was measured 24 h after cells were scratched. Values are presented as mean ± SD. KIF15, kinesin superfamily member 15; NPC, nasopharyngeal carcinoma; transwell assays: stained with 0.1% crystal violet staining solution; original magnification ×200. *p < 0.05 and **p < 0.01 vs. the shcontrol group, n = 5.

Fig. 5
Effect of KIF15 knockdown on AKT and p53-dependent pathway of NPC cell lines.
(A) The protein expression of p-AKT, AKT (B), P53 (C), MMP2 (E), and MMP9 (G) were detected in KIF15-shRNA/CNE-2 and ScrshRNA/CNE-2 cell lines. (H) The protein expression of p-AKT, AKT (I), P53 (J), MMP2 (L), and MMP9 (N) were detected in KIF15-shRNA/CNE-2 and ScrshRNA/CNE-2 cell lines. The mRNA expression of MMP2 (D, F) and MMP9 (K, M) were detected. Values are presented as mean ± SD. KIF15, kinesin superfamily member 15; NPC, nasopharyngeal carcinoma. *p < 0.05 and **p < 0.01 vs. the shcontrol group, n = 5.
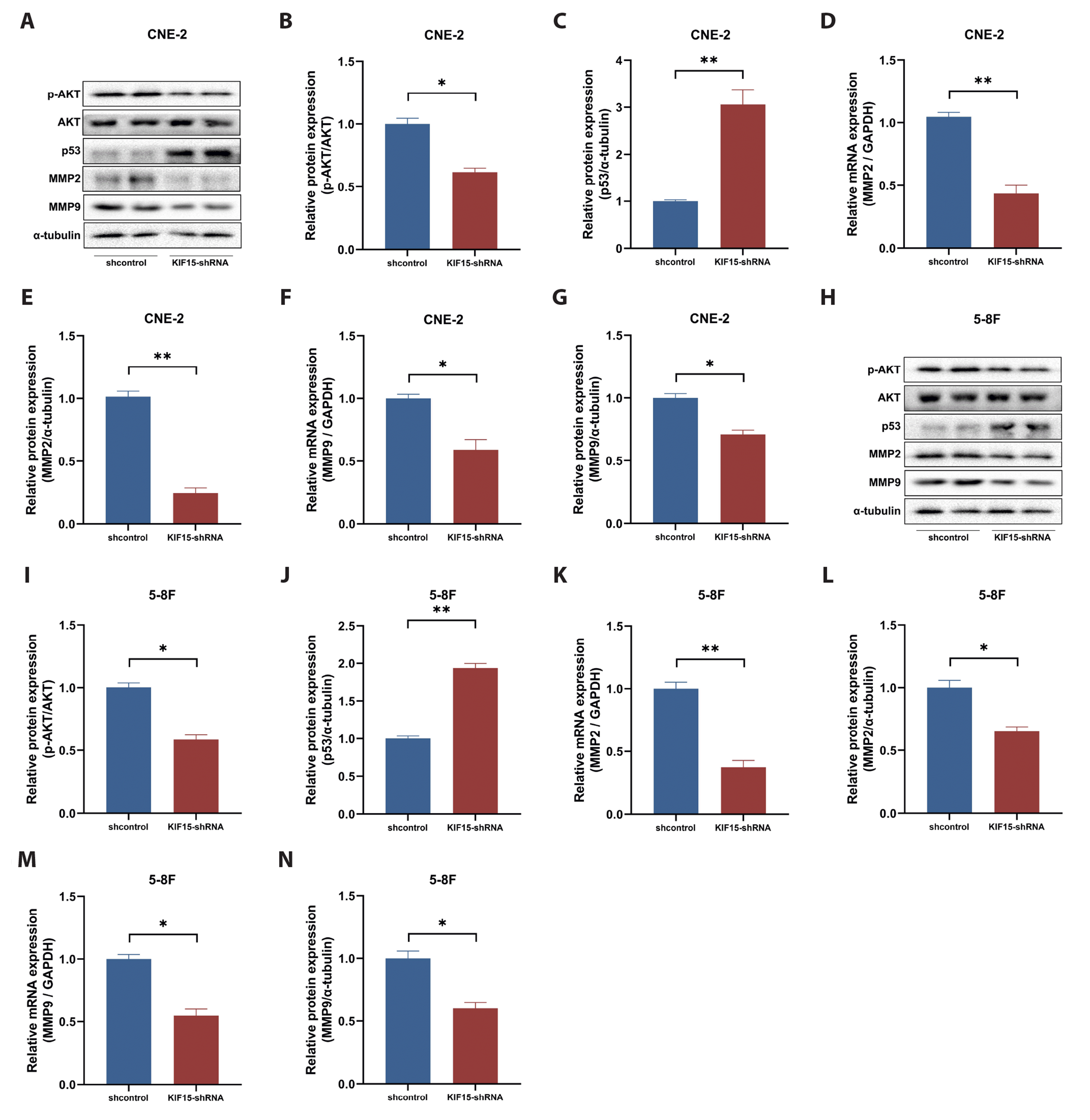
Fig. 6
KIF15 knockdown induces apoptosis in NPC cells through activation of p53.
CNE-2 (A) or 5-8F (B) cells were transfected with sip53 or negative control (NC) for 48 h, the mRNA expression of p53 was detected. KIF15-shRNA/CNE-2 (C, E) or KIF15-shRNA/5-8F (D, F) cells were transfected with sip53 or NC. The flow cytometric analysis was detected. The mRNA expression of bax (G, H), bcl-2 (I, J) and bax/bcl-2 (K, L) were detected. The protein expression of cleaved-caspase 3 was detected in CNE-2 (M) and 5-8F (N) cells. Values are presented as mean ± SD. KIF15, kinesin superfamily member 15; NPC, nasopharyngeal carcinoma. *p < 0.05 and **p < 0.01 vs. the KIF15-shRNA-NC group, n = 5.
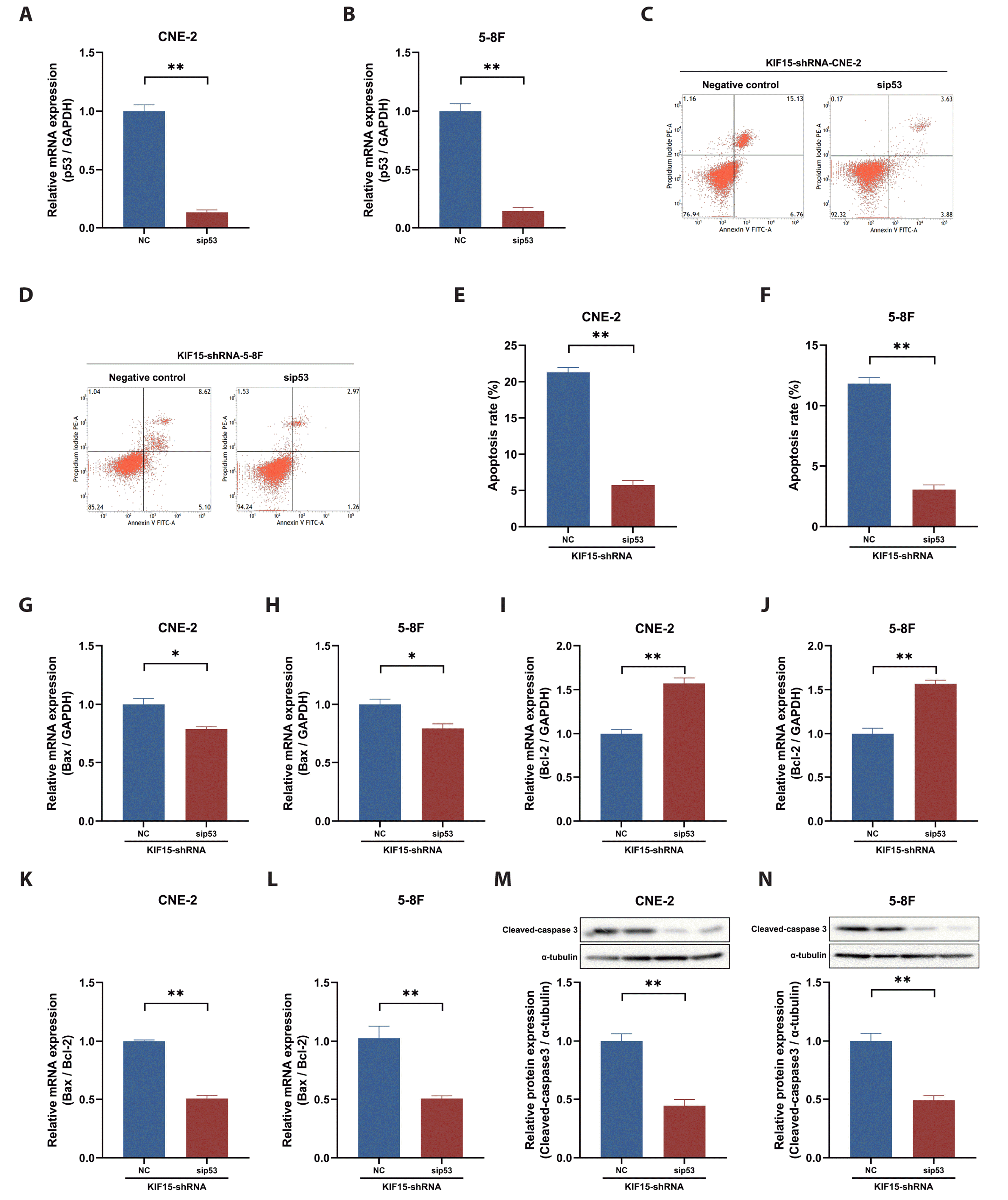
Fig. 7
Effect of KIF15 knockdown on NPC cell growth in vivo.
(A, B) Mice and tumors in the different groups were shown. Tumor volume (C) and body weight (D) were recorded. (E, F) Representative in vivo images of luminescence shown in the livers of the live nude mouse was injected in KIF15-shRNA/CNE-2 and ScrshRNA/CNE-2 cell lines, respectively. Values are presented as mean ± SD. KIF15, kinesin superfamily member 15; NPC, nasopharyngeal carcinoma. **p < 0.01 vs. the shcontrol group, n = 5.

Fig. 8
Molecular mechanism of KIF15 on NPC development.
KIF15, kinesin superfamily member 15; NPC, nasopharyngeal carcinoma.
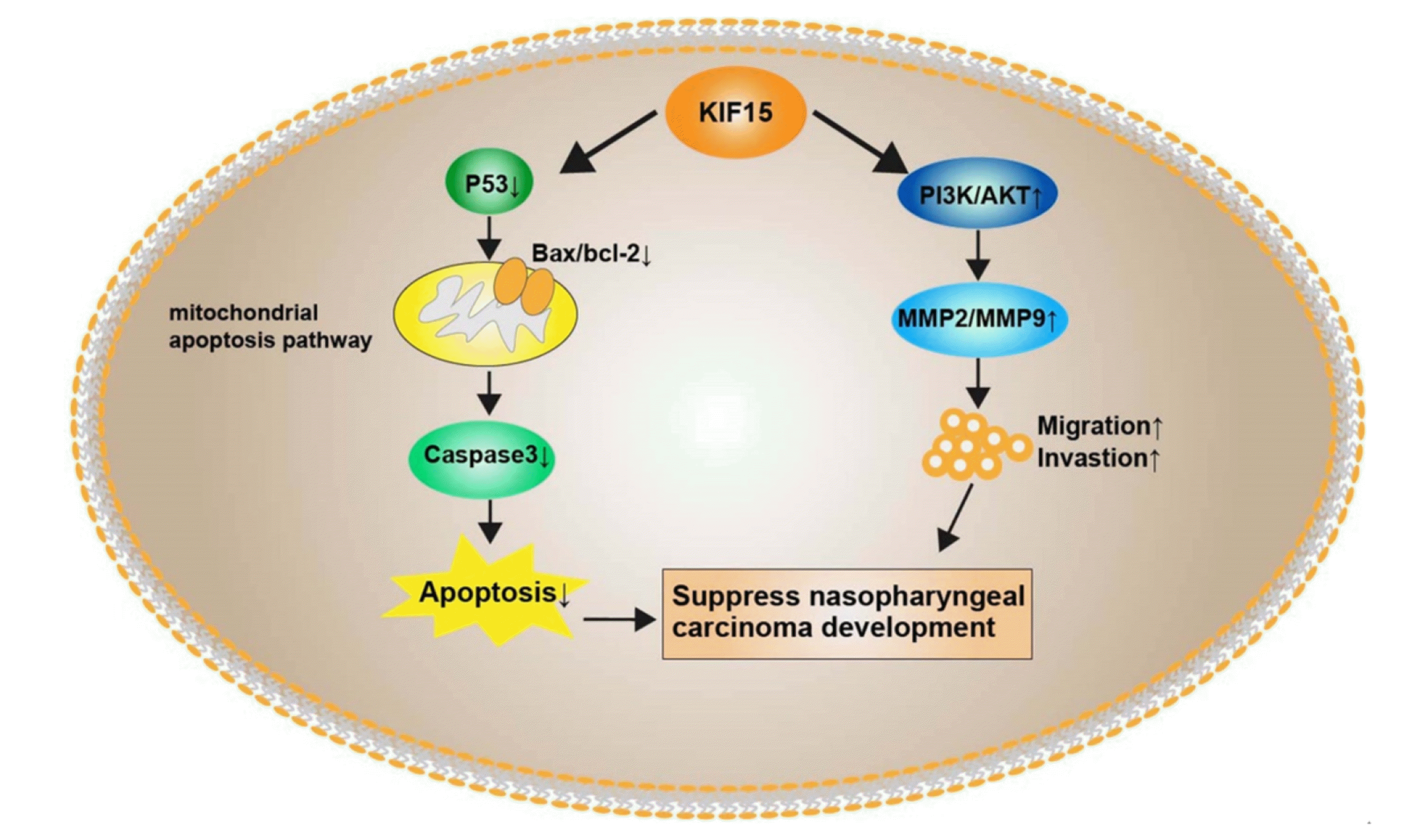
Table 1
The siRNA sequences for p53
siRNA | Sequences |
---|---|
p53-siRNA | Sense: 5'- UCAAAUCAUCCAUUGCUUGGG -3' |
Antisense: 5'- CAAGCAAUGGAUGAUUUGAUG -3' | |
Negative control |
Sense: 5'- GCAUCUCCCUAGACCGAUUTT -3' |
Antisense: 5'- AAUCGGUCUAGGGAGAUGCTT -3' |
Table 2
shRNA sequences for KIF15
Table 3
Primer sequences for qRT-PCR